Abstract
Context: Blackcurrant (Ribes nigrum L.) is a classical fruit that has long been used to make juice, liqueur and sometimes medicines in Europe. The beneficial effects of blackcurrant, which are inhibition of lipopolysaccharide-stimulated inflammatory, anticarcinogenesis and other health effects, have been reported.
Objective: Previously, we reported the antimutagenic activities of blackcurrant using a yeast gene mutation assay. In this study, we investigated whether this antimutagenicity of blackcurrant was confirmed in human cells.
Materials and methods: We prepared four types of aqueous blackcurrant extracts (BCE) from mature and premature with or without heat treatment by microwave. Antioxidant activities of BCE were measured by the DPPH radical scavenger assay. In the DPPH radical scavenger assay, the maximum concentration of BCE was 1.6 mg/reaction. We investigated the antigenotoxic activities of BCE by the comet assay and micronucleus test using the human lymphoblastoid cell line TK6. In the comet assay, TK6 was treated with 300 μM H2O2 without or with BCE at concentrations of 0.5, 1.0, 2.0 and 3.0 mg/mL. In the micronucleus test, TK6 was treated with 1 mg/mL BCE without or with H2O2.
Results: All BCEs exhibited more than 90% of inhibition rates of DPPH radicals at the maximum concentration of BCE. DNA damage and micronuclei induced by H2O2 significantly decreased in the each BCE-treated condition.
Conclusion: The results suggest that BCE treatment can reduce the genomic instability induced by H2O2 in human cells. We consider that these antigenotoxic effects are related to polyphenols, l-ascorbic acid and other antioxidant compounds.
Introduction
In recent years, cancer and lifestyle-related diseases have received a lot of global attention. This is particularly true in Japan, where cancer is the leading cause of death and about 30% of Japanese people are suffering from some kind of lifestyle-related disease. Against this background, we have focused on the relationships between DNA damage, chromosomal aberration, gene mutation and these diseases. It is considered that the starting point of these diseases is gene mutation, following DNA damage induced by endogenous and exogenous stresses, such as cigarette smoke, ultraviolet radiation, ionizing radiation and reactive oxygen species (ROS) generated in energy metabolism (Alexandrov et al., Citation2010; Ikehata & Ono, Citation2011; Lagerwerf et al., Citation2011; Mladenov & Iliakis, Citation2011; Murata et al., Citation2012). These stresses induce DNA damage and genomic dysfunction. Studies on natural foods and other products have attracted attention for the prevention of carcinogenesis and lifestyle-related diseases (Furtado et al., Citation2010; Sardaş et al., 2001; Sato et al., Citation2005; Scolastici et al., Citation2008). Numerous plants have been widely used as food and for medical ingredients in different cultures from time immemorial. It has been reported that a range of natural foods and other products can prevent carcinogenesis and lifestyle-related diseases in association with their antioxidant and antimutagenic activities (Jain et al., Citation2009; Nakata et al., Citation2012; Weisburger, Citation2001). Thus, we consider the daily reduction of DNA damage by natural food intake to be an important approach to prevent these diseases.
Recently, we reported that the extract of mature and premature blackcurrant (Ribes nigrum L.) fruit had high antioxidant and antimutagenic activities, as evaluated using a yeast loss of heterozygosity (LOH) system (Yamamoto et al., Citation2012). Blackcurrant is a classical fruit that has long been used to make juice, jam, liqueur and sometimes medicines in Europe. Currently, Aomori prefecture, located in the northeast of Japan, accounts for approximately 90% of the blackcurrant production in Japan. In our recent study, the induction of mutations by several mutagens, namely, hydrogen peroxide (H2O2), methylmethanesulfonate (MMS) and ultraviolet radiation (UV), was significantly suppressed by the combined treatment of different types of blackcurrant extract (BCE). It is not clear, however, whether this antimutagenic activity is functional in human cells.
Oxidative stress associated with various factors including energy metabolism, exogenous chemicals, and ultraviolet and ionizing radiation is related to a range of diseases, such as carcinogenesis, arteriosclerosis, metabolic syndrome and other lifestyle-related diseases (Gray & Bennett, Citation2011; Hutcheson & Rocic, Citation2012; Pan et al., Citation2012; Tudek & Speina, Citation2012). In the present study, the antioxidant activity of several types of BCE against DPPH free radicals was evaluated. In addition, we report the antigenotoxic effects of BCEs extracted from mature and premature fruit, with or without heat treatment, against H2O2-induced oxidative stress in human lymphoblastoid cells using the comet and micronucleus (MN) assays. Since one of the major food preparation methods of blackcurrants is microwave cooking to make jam, the evaluation of heat-treated fruit is important.
Materials and methods
Cell culture
The human lymphoblastoid cell line TK6 was grown on RPMI 1640 medium (Nakalai Tesque, Kyoto, Japan) supplemented with 100 U/ml penicillin, 100 μg/ml streptomycin, 0.25 μg/m amphotericin B, 10% heat-inactivated fetal bovine serum and 200 μg/ml sodium pyruvate (Yamamoto et al., Citation2011). TK6 was incubated at 37 °C in a 5% CO2 atmosphere with 100% humidity.
Chemicals
Hydrogen peroxide (CAS No.7722-84-1) was purchased from Wako Pure Chemical Industries, Ltd., Osaka, Japan.
Preparation of BCE
Mature and premature blackcurrant fruits were provided by Aomori Blackcurrant Association. The preparation of BCE was conducted as follows. To prepare heat-treated blackcurrant extract, 300 g of blackcurrant fruit was heated in a microwave at 500 W for 3 min. The types of BCE made from mature fruit and heated-mature fruit are abbreviated as M-BCE and MH-BCE, and those from premature fruit and heated-premature fruit are abbreviated as P-BCE and PH-BCE, respectively.
Antioxidant activity assay (DPPH radical scavenger assay)
DPPH radical scavenger assay was performed as follows. First, 0.1 mL samples were mixed with 8.9 mL of DPPH reaction solution (0.1 M acetate buffer, 0.016% 1,1-diphenyl-2-picrylhydrazyl in ethanol, 84% xylene) and absorbance at 510 nm was measured. The inhibition ratio was calculated using the below formula (Yamamoto et al., Citation2012). A.S. is the absorbance of the sample and A.C. is the absorbance of the control, in which there are no antioxidant reagents.
Comet assay
The alkaline comet assay (single-cell gel electrophoresis, SCGE) was conducted as described previously (Kawaguchi et al., Citation2010). TK6 was treated with H2O2 for 4 h at a concentration of 300 μM without or with BCE at concentrations of 0.5, 1.0, 2.0 and 3.0 mg/mL. Briefly, 1.0 mL of treated cell suspension was centrifuged, washed and suspended with 1.0 mL of PBS. Then, 10 μl of the cell solution was mixed with 0.5% low-melting agarose gel. The mixture including cells was spread on an MAS-coated microscope slide (Matsunami Glass Ind., Ltd., Osaka, Japan). The agarose gel was then allowed to solidify for 10 min at room temperature. The cells packaged in the agarose gel were lysed at 4 °C overnight in 2.5 M NaCl, 100 mM Na2EDTA, 10 mM Tris, 10% DMSO and 1% TritonX-100, pH 10. After the cell lysis, slides were transferred to an electrophoresis chamber filled with an ice-cold solution of 300 mM NaOH and 1 mM EDTA at pH > 13 for 20 min to allow the DNA to unwind. Then, electrophoresis was carried out for 15 min at 300 mA. Finally, the slides were neutralized for 5 min in 0.4 M Tris buffer (pH 7.5), rinsed with 99% EtOH for 5 min and then air-dried. The cells were subsequently stained with ethidium bromide (Wako Pure Chemical Industries, Ltd., Osaka, Japan), and 100 cells were analyzed per concentration with a fluorescence microscope (Olympus, Tokyo, Japan). The entire length of the comet (“migration”) was measured.
MN assay
MN assay was conducted as described previously (Yamamoto et al., Citation2011). TK6 was treated with H2O2 for 4 h at the concentrations of 20, 30 and 40 μM without or with BCE at a concentration of 1.0 mg/mL. In MN assay, H2O2 concentration was lower than that used in the comet assay, because the detection sensitivity of MN assay was higher than the comet assay (Kawaguchi et al., Citation2010). Briefly, after 48 h of treatment, approximately 106 cells were suspended in 0.075 M KCl and then incubated for 10 min at room temperature. The suspended cells were fixed with ice-cold methanol containing 25% acetic acid. Then, the fixed cells were centrifuged and re-suspended with ice-cold methanol containing 25% acetic acid. In the final fixation, the fixed cells were suspended in ice-cold methanol containing 1.0% acetic acid. A drop of fixing cell solution was spotted on slide glasses, and then the glasses were air-dried. The fixed cells were stained with acridine orange (Wako Pure Chemical Industries, Ltd., Osaka, Japan) and analyzed with a fluorescence microscope (Olympus, Tokyo, Japan).
Measurements of intracellular ROS
Intracellular ROS was measured using 50 µM BES-H2O2-Ac purchased from Wako Pure Chemical Industries (Osaka, Japan). TK6 cells (5 × 105/mL) were incubated with BES-H2O2-Ac for 1 h. After washing with PBS, TK6 cells were treated with 300 μM H2O2 with or without BCEs for 1 h at 37 °C. And then, cells more than 100 were counted to estimate the percentage or ROS positive cells.
Total polyphenols, anthocyanins and l-ascorbic acids
Quantification of total polyphenolic content using the Folin–Ciocalteu assay was conducted as described previously (Takahashi et al., Citation2011). First, 500 μL of 1 N Folin–Ciocalteu’s phenol reagent (Kanto Chemial Co., Inc., Tokyo, Japan) and 500 μL of diluted sample, standard or blank were added. This mixture was left to stand for 3 min before the addition of 2.5 mL of 0.4 M Na2CO3. The solution was again left to stand for 5 min at 50 °C. After this incubation, the absorbance at 765 nm was recorded with a U-1500 spectrophotometer (Hitachi High-Technologies Corporation, Tokyo, Japan). (+)-Catechin (Sigma-Aldrich, Co., LLC, St. Louis, MO) was used to create a standard curve, and the results were expressed as milligrams of (+)-catechin equivalents per milliliter of BCE. To analyze the concentration of BCE anthocyanin compounds, the absorbance of BCE was recorded with a U-1500 spectrophotometer. The anthocyanin concentration was calculated from the absorbance at 520 nm using a molar extinction coefficient of 29 600 M−1 cm−1 (Yamamoto et al., Citation2012). l-Ascorbic acid concentration was measured using the F-kit purchased from Roche Diagnostics (Basel, Switzerland).
Statistical analysis
For all the assays, statistical analyses were performed using Student’s t-test. The standard deviation (SD) was calculated from three separate experiments. Data analyses were carried out with Excel software (Microsoft, New York, NY).
Results
Antioxidant activity
shows the results of the antioxidant activities. BCEs, which were extracted from mature blackcurrant fruits () and premature blackcurrant fruits (), dose-dependently removed DPPH radicals. At the maximum concentration of BCE (1.6 mg/reaction), inhibition rates of DPPH radicals of 92.4, 91.9, 91.2 and 91.3% were obtained for M-BCE, MH-BCE, P-BCE and PH-BCE, respectively. The IC50 values for M-BCE, MH-BCE, P-BCE and PH-BCE were 347.2, 318.5, 441.7 and 331.8 μg/reaction, respectively. Intriguingly, antioxidant activities of PH-BCE were slightly higher than those of P-BCE with a significant difference (p < 0.05).
Figure 1. DPPH free radical scavenging activity of BCEs. The ordinate axis indicates the ratio of inhibition of DPPH radicals. In this experiment, 0.016% of DPPH reaction solution and 0 to 1.6 mg of blackcurrant extract from mature fruit (filled circle), heated-mature fruit (opened circle), premature fruit (filled diamond) and heated-premature fruit (opened diamond) were used. Each symbol represents the mean value of three experiments. An asterisk (*) indicates p < 0.05 in the t-test comparing P-BCE and PH-BCE.
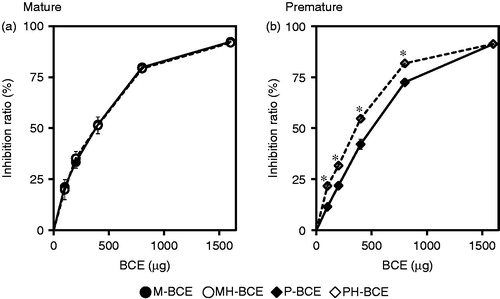
Inhibition of H2O2-induced DNA damage
shows the DNA damage inhibition effect of several types of BCE. The DNA migration of each BCE single treatment was similar to that of H2O2-untreated cells. This indicates the absence of a genotoxic effect in each type of BCE. The H2O2 treatment, as a DNA oxidative damage inducer, induced a significantly high level of DNA strand breaks compared with that in H2O2-untreated cells (p < 0.00005). DNA migration of H2O2 and BCE-untreated cells was 2.31 ± 0.12 μm. On the other hand, H2O2 alone induced 9.38 ± 0.11 μm of DNA migration. In combination with H2O2 and each BCE, the DNA migration significantly decreased when the cells were treated with BCE at all concentrations. At a concentration of 3.0 mg/mL BCE, the DNA migration decreased to less than 50% of H2O2 alone treatment in all types of BCE-treated conditions. Thus, BCE treatments clearly inhibited DNA damage induced by H2O2 treatment in TK6 cells. As was the case for antioxidant activity, the DNA strand breaks were significantly decreased by PH-BCE treatment compared with those by P-BCE treatment at 2.0 mg/mL treatment condition (p < 0.05).
Figure 2. Effects of BCEs on H2O2-induced DNA damage (DNA migration) in human lymphoblastoid TK6 cells. The effects of BCE extracted from mature fruit (a) and premature fruit (b) are separately presented. In this experiment, 0 to 3.0 mg/mL of blackcurrant extract was treated without or with 300 µM of H2O2. Filled square, unheated blackcurrant fruit without H2O2-treatment; Opened square without H2O2-treatment; Filled circle, unheated blackcurrant fruit with H2O2-treatment; Opened circle, heated blackcurrant fruit with H2O2-treatment. Each symbol represents the mean value of three experiments. A dagger (†) indicates p < 0.00005 in the t-test comparing H2O2-treated (300 μM) and untreated cells. A number sign (#) indicate p < 0.05 in the t-test comparing P-BCE and PH-BCE. An asterisk (*) and two asterisks (**) indicate p < 0.05 and p < 0.005, respectively, in the t-test comparing H2O2-treated and H2O2 + BCE-treated cells.
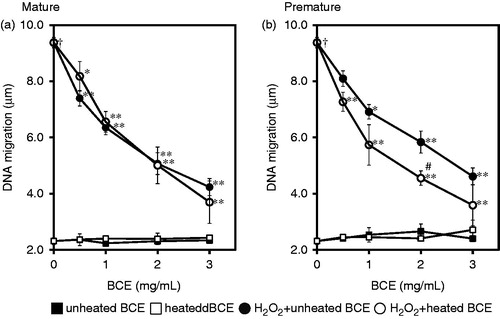
Inhibition of H2O2-induced micronucleus
shows the effect of suppression of H2O2-induced MN formation by combined-treatment of BCE in TK6 cells. Treatment with H2O2 alone enhanced the frequency of MN formation, that is, the number of cells with MN per 1000 cells, in a dose-dependent manner. In the combined treatments, the MN frequencies significantly decreased in a dose-dependent manner. The highest inhibitory effect was observed in the M-BCE-treated cells, which inhibition ratio was 40% at a concentration of 40 μM H2O2. In the PH-BCE-treated cells, the frequency of MN formation tended to be lower than in P-BCE-treated cells, which inhibition trend did significant, as was the case for the results of antioxidant activity and comet assay.
Figure 3. Effects of BCEs on H2O2-induced chromosomal aberration (micronucleus formation) in human lymphoblastoid TK6 cells. The effects of BCE extracted from mature fruit (a) and premature fruit (b) are separately presented. Dark gray column, H2O2 single treatment; Light gray column, H2O2 treatment combined with unheated blackcurrant extract; White column, H2O2 treatment combined with heated blackcurrant extract. Each column represents the average of three experiments. A dagger (†) indicates p < 0.005 in the t-test comparing H2O2-treated (20, 30, 40 μM) and untreated cells. An asterisk (*), two asterisks (**) and three asterisks (***) indicate p < 0.1, p < 0.05 and p < 0.005, respectively, in the t-test comparing H2O2-treated and H2O2 + BCE (1.0 mg)-treated cells.
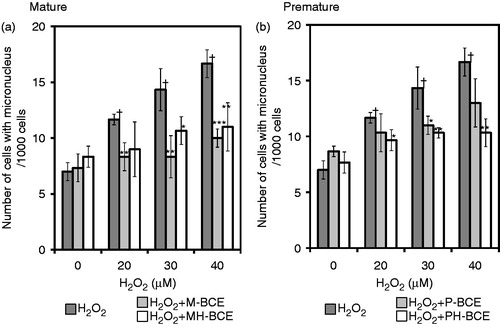
Intracellular ROS scavenging activity of BCE
We investigated the intracellular H2O2 as an index of ROS to evaluate the ROS scavenging activity of BCE against H2O2-induced ROS using fluorescent probe BES-H2O2-Ac, which was the detection reagent for intracellular H2O2. The percentage of intracellular H2O2 positive cells was approximately 12% in untreated cells, while the percentage of the ROS positive cells induced by H2O2-treatment significantly increased to 35% (p < 0.05) (). On the other hand, the intracellular ROS production was significantly blocked by BCE-combined treatment (p < 0.05). There was no significant difference between each BCE inhibition ratio.
Figure 4. Intracellular ROS scavenging activity of BCE. Intracellular ROS was measured using BES-H2O2-Ac fluorescent probe. H2O2-induced ROS was reduced by BCE (1.0 mg/mL). The ROS-positive cells more than 100 were counted in each treatment. A dagger (†) indicates p < 0.05 in the t-test comparing H2O2-treated (300 μM) and untreated cells. An asterisk (*) indicate p < 0.05 in the t-test comparing H2O2-treated and H2O2 + BCE-treated cells.
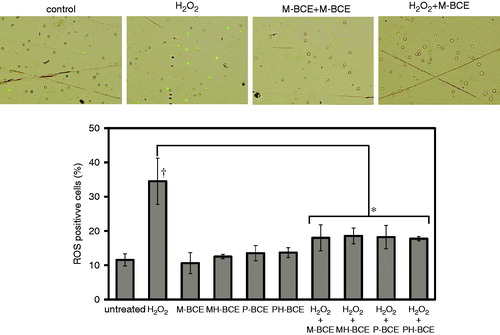
Total polyphenols, anthocyanins and l-ascorbic acid concentrations
Total polyphenolic contents calculated as (+)-catechin equivalents are shown in . While the total polyphenol contents of M-BCE, MH-BCE and P-BCE were almost the same, interestingly, PH-BCE showed a higher polyphenolic content. Total anthocyanins of each BCE estimated by calculation with the molar absorbance coefficient are shown in . Mature blackcurrant had a high concentration of anthocyanins compared with premature blackcurrant. In both mature and premature BCE, the concentration of anthocyanins extracted from heat-treated blackcurrant was decreased compared with that of natural blackcurrants. In mature BCE, the anthocyanin concentration of MH-BCE was approximately 37% of that of M-BCE. l-Ascorbic acid concentration was higher in the premature BCEs than in the mature BCEs. In both premature and mature BCEs, heat-treated BCEs showed an abundance of l-ascorbic acid.
Table 1. Total polyphenols, anthocyanins and l-ascorbic acid content in the BCEs.
Discussion
Since the interest in daily healthcare by the intake of natural foods and other products is continuing to rise against the background of an aging society, increasing environmental pollution and a growing awareness of various factors related to quality of life, it is important to evaluate such foods for antioxidative and antimutagenic activities. Blackcurrant is well known for its positive impact on health due to a high level of polyphenols, including anthocyanins, vitamins, minerals and other beneficial compounds (Bakowska-Barczak et al., 2009; Matsumoto et al., Citation2001). The health benefits of blackcurrant have been reported all over the world. In a previous study, blackcurrant extract exhibited high antioxidant activity, inhibition of enlargement of globe component dimensions and suppression of the proliferation of HT29 colon cancer cells and MCF-7 breast cancer cells (Olsson et al., Citation2004). Recently, we also reported the antimutagenic effect of blackcurrant extracts, from premature and mature fruit, evaluated using a yeast LOH system (Yamamoto et al., Citation2012). Both BCEs inhibited DNA mutations induced by H2O2 as an oxidative damage agent, MMS as an alkylating agent and UV as physical stress.
In this study, we investigated the antigenotoxic effect of several types of BCE, which were extracted from mature or premature fruit with or without heat treatment, against DNA damage induced by H2O2 in human lymphoblastoid TK6 cells using two end-point methods, namely, comet assay relating to primary DNA damage and MN assay relating to chromosome aberration. Additionally, we demonstrated evaluation of the antioxidative activity by DPPH radical scavenger assay and of the intracellular ROS scavenging activity using a fluorescent probe. The results obtained showed that all BCEs used in this study had high antioxidant activity which was observed intracellular ROS analysis, and they presented antigenotoxic effects against H2O2-induced DNA damage and chromosomal aberration in human lymphoblastoid cells. H2O2 is the major inducer of oxidative stress, and it induces several types of genomic instability, including DNA adducts such as 8-hydroxyguanosine (8-oxodG, 8OHdG, 8OHG), sister chromatid exchange, DNA strand breaks and histone modification (Klaunig et al., Citation2010; Limoli et al., Citation2003; Steinboeck et al., Citation2010; Tomita et al., Citation2003; Turner et al., Citation2003; Ventura et al., Citation2010). As polyphenolic compounds and l-ascorbic acid have antioxidant activity with radical scavenging function, BCEs including various polyphenols and vitamins exhibit antimutagenicity (Yamamoto et al., Citation2012). The mature BCE treatments exhibited high activity compared with premature BCE treatments in terms of both antioxidant activity and antimutagenic activity. In a recent study, it was reported that blackcurrant seed extract, prepared differently from the approaches used in this study, decreased the spontaneous MN frequency in human lymphocyte cells (Gođevac et al., Citation2012). While we could not detect an inhibitory effect on spontaneous genomic aberrations in our experiment, these data strongly suggested that blackcurrant has the potential to act in the defense of genomic DNA. Since the difference of antioxidative activity was associated with the composition of antioxidant, our previous study showed that M-BCE exhibited higher activities than P-BCE in the yeast cells. In human lymphoblastoid cells, mature BCEs also showed high genome defense activities, which were associated with high antioxidant activity, compared with premature BCEs. The several activities of MH-BCE were not quite increased compared to other BCEs, while MH-BCE included highest polyphenols and l-ascorbic acid. As it was reported that fruit dietary soluble fiber had good antioxidant capacity (Martinez et al., Citation2012), we consider there is a possibility that the contents and properties of soluble fiber are related to antioxidative and antigenotoxic activities of BCE. Thus, it is important to analyze the composition of soluble fiber included in BCEs and to evaluate these antioxidative capacities. Interestingly, in the premature BCE treatments, PH-BCE had high antioxidative and antigenotoxic activities compared with unheated P-BCE. This means that premature blackcurrants are made more useful by heat processing. Indeed, some previous studies reported that antioxidant activity was increased by heat treatment (Akira & Yamashita, Citation2000; Ikeba & Kashima, Citation2006; Kang et al., Citation2007; Shibata et al., Citation2005). In this study, we measured the concentrations of total polyphenols, anthocyanins and l-ascorbic acid, which are major antioxidants. As the concentrations of total polyphenols and l-ascorbic acid in PH-BCE were higher than in P-BCE, it is possible that PH-BCE showed high antioxidant and antigenotoxic activities compared with P-BCE. While it is considered possible that this increment of antioxidative activity is attributable to the promotion of cell inclusion elution with plant cell wall destruction by heat treatment, this mechanism has not yet been elucidated (Ikeba & Kashima, Citation2006). While total polyphenolic content for PH-BCE was higher than for M-BCE and MH-BCE, PH-BCE activities were almost the same as those for M-BCE and MH-BCE. We should clarify the levels and constituents among polyphenols and other antioxidants before or after heat treatment in BCE in the near future.
Conclusions
In conclusion, we have demonstrated that BCEs, which were extracted from mature and premature blackcurrants with or without heat treatment using a microwave oven, exhibited high antioxidative and antigenotoxic activities against H2O2-induced oxidative stress in the human lymphoblastoid TK6 cell line. Since oxidative stress is one of the major factors associated with carcinogenesis and lifestyle-related diseases, we consider these BCE activities have useful roles in daily healthcare for the protection of genomic DNA. However, further studies should be performed to obtain a deeper understanding of the active compounds, antigenotoxicity mechanisms and in vivo activity.
Declaration of interest
The authors report no declarations of interest. This work was supported by JSPS Grant-in-Aid for Young Scientists (B) Grant Number 24780136, by Grant-in-Aid from the Japan Cassis Association and by the Agriculture, Forestry and Fisheries Department of Aomori city.
Acknowledgements
The authors are grateful to Dr. Kazuo Yamamoto, emeritus professor at Tohoku University, for his advice about DNA damage and repair mechanisms. The authors are also grateful to Dr. Yu F. Sasaki, professor at Hachinohe National College of Technology, for his advice about the comet assay.
References
- Akira K, Yamashita S. (2000). Antioxidative activity of fresh and heated vegetable extracts. Bull Fukuoka Agric Res Cent 19:81–4
- Alexandrov K, Rojas M, Satarug S. (2010). The critical DNA damage by benzo(a)pyrene in lung tissues of smokers and approaches to preventing its formation. Toxicol Lett 198:63–8
- Bakowska-Barczak AM, Schieber A, Kolodziejczyk P. (2009). Characterization of Canadian black currant (Ribes nigrum L.) seed oils and residues. J Agric Food Chem 57:11528–36
- Furtado RA, de Araújo FR, Resende FA, et al. (2010). Protective effect of rosmarinic acid on V79 cells evaluated by the micronucleus and comet assays. J Appl Toxicol 30:254–9
- Gođevac D, Tešević V, Vajs V, et al. (2012). Chemical composition of currant seed extracts and their protective effect on human lymphocytes DNA. J Food Sci 77:779–83
- Gray K, Bennett M. (2011). Role of DNA damage in atherosclerosis-Bystander or participant? Biochem Pharmacol 82:693–700
- Hutcheson R, Rocic P. (2012). The metabolic syndrome, oxidative stress, environment, and cardiovascular disease: The great exploration. Exp Diabetes Res [Online]. Available from: http://www.hindawi.com/journals/edr/2012/271028/ [last accessed 11 Nov 2012]. Article ID:271028
- Ikeba T, Kashima K. (2006). Evaluation of antioxidant activity of raw and cooked vegetable extracts produced in Ibaraki prefecture. Bull Ibaraki Agric cent 14:27–33
- Ikehata H, Ono T. (2011). The mechanisms of UV mutagenesis. J Radiat Res 52:115–25
- Jain N, Naseem I, Ahmad J. (2009). Evaluation of DNA damage and metabolic syndrome parameters in diabetic rabbits supplemented with antioxidants. Fundam Clin Pharmacol 23:197–205
- Kang KS, Yamabe N, Kim HY, et al. (2007). Increase in the free radical scavenging activities of American ginseng by heat processing and its safety evaluation. J Ethnopharmacol 113:225–32
- Kawaguchi S, Nakamura T, Yamamoto A, et al. (2010). Is the comet assay a sensitive procedure for detecting genotoxicity? J Nucleic Acids [Online]. Available from: http://www.hindawi.com/journals/jna/2010/541050/ [last accessed 11 Nov 2012]. Article ID:541050
- Klaunig JE, Kamendulis LM, Hocevar BA. (2010). Oxidative stress and oxidative damage in carcinogenesis. Toxicol Pathol 38:96–109
- Lagerwerf S, Vrouwe MG, Overmeer RM, et al. (2011). DNA damage response and transcription. DNA Repair (Amst) 10:743–50
- Limoli CL, Giedzinski E, Morgan WF, et al. (2003). Persistent oxidative stress in chromosomally unstable cells. Cancer Res 63:3107–11
- Martínez R, Torres P, Meneses MA, et al. (2012). Chemical, technological and in vitro antioxidant properties of mango, guava, pineapple and passion fruit dietary fibre concentrate. Food Chem 135:1520–6
- Mladenov E, Iliakis G. (2011). Induction and repair of DNA double strand breaks: The increasing spectrum of non-homologous end joining pathways. Mutat Res 711:61–72
- Matsumoto H, Hanamura S, Kawakami T, et al. (2001). Preparative-scale isolation of four anthocyanin components of black currant (Ribes nigrum L.) fruits. J Agric Food Chem 49:1541–5
- Murata M, Thanan R, Ma N, Kawanishi S. (2012). Role of nitrative and oxidative DNA damage in inflammation-related carcinogenesis. J Biomed Biotechnol [Online]. Available from: http://www.hindawi.com/journals/biomed/2012/623019/ [last accessed 11 Nov 2012]. Article ID:623019
- Nakata R, Takahashi S, Inoue H. (2012). Recent advances in the study on resveratrol. Biol Pharm Bull 35:273–9
- Olsson ME, Gustavsson KE, Andersson S, et al. (2004). Inhibition of cancer cell proliferation in vitro by fruit and berry extracts and correlations with antioxidant levels. J Agric Food Chem 52:7264–71
- Pan MH, Lai CS, Tsai ML, et al. (2012). Molecular mechanisms for anti-aging by natural dietary compounds. Mol Nutr Food Res 56:88–115
- Sardaş S, Yilmaz M, Oztok U, et al. (2001). Assessment of DNA strand breakage by comet assay in diabetic patients and the role of antioxidant supplementation. Mutat Res 490:123–9
- Sato I, Suzuki T, Kobayashi H, Tsuda S. (2005). Antioxidative and antigenotoxic effects of Japanese horse chestnut (Aesculus turbinata) seeds. J Vet Med Sci 67:731–4
- Scolastici C, Alves de Lima RO, Barbisan LF, et al. (2008). Antigenotoxicity and antimutagenicity of lycopene in HepG2 cell line evaluated by the comet assay and micronucleus test. Toxicol In Vitro 22:510–14
- Shibata K, Watanabe Y, Negishi Y, Yasuhara Y. (2005). Effects of cooking methods on chlorogenic acid derivatives and DPPH radical scavenging activity of sweet potato. Jpn Soc Cookery Sci 38:324–32
- Steinboeck F, Hubmann M, Bogusch A, et al. (2010). The relevance of oxidative stress and cytotoxic DNA lesions for spontaneous mutagenesis in non-replicating yeast cells. Mutat Res 688:47–52
- Takahashi T, Ichita J, Kato Y. (2011). Production of vinegar from apple pomace and its physiological function. Nippon Shokuhin Kagaku Kougaku Kaishi 58:37–42
- Tomita K, Barnes PJ, Adcock IM. (2003). The effect of oxidative stress on histone acetylation and IL-8 release. Biochem Biophys Res Commun 301:572–7
- Tudek B, Speina E. (2012). Oxidatively damaged DNA and its repair in colon carcinogenesis. Mutat Res 736:82–92
- Turner DR, Dreimanis M, Holt D, et al. (2003). Mitotic recombination is an important mutational event following oxidative damage. Mutat Res 522:21–6
- Ventura I, Russo MT, De Luca G, Bignami M. (2010). Oxidized purine nucleotides, genome instability and neurodegeneration. Mutat Res 703:59–65
- Weisburger JH. (2001). Antimutagenesis and anticarcinogenesis, from the past to the future. Mutat Res 480–481:23–5
- Yamamoto A, Kawaguchi S, Nakashima K, et al. (2012). The antimutagenic activities of blackcurrant extract: Evaluation using saccharomyces cerevisiae LOH system. Genes Environ 34:115–22
- Yamamoto A, Sakamoto Y, Masumura K, et al. (2011). Involvement of mismatch repair proteins in adaptive responses induced by N-methyl-N’-nitro-N-nitrosoguanidine against γ-induced genotoxicity in human cells. Mutat Res 713:56–63