Abstract
Context: Diabetes mellitus (DM) type 2 is a chronic disease characterized by hyperglycemia and insulin resistance. Oxidative stress participates in development and progression of DM, in which changes of superoxide dismutase (SOD) and catalase (CAT) were noted in DM mice. Berberine has been widely used as an alternative medicine and proved to be effective for the treatment of DM and dyslipidemia.
Objective: Impacts of berberine on transcriptional regulation of SOD and CAT and their enzyme activities, including the level of malondialdehyde (MDA) formation, were examined in the DM type 2-induced mice to clarify its antioxidation potential, compared with a common hypoglycemic drug, glibenclamide.
Materials and methods: Noninsulin-dependent diabetes was induced in mice by a single intraperitoneal streptozotocin–nicotinamide injection. Diabetic mice were treated daily with glibenclamide (10 mg/kg/d) and/or berberine (100 mg/kg/d) for 2 weeks. The fasting blood glucose and the MDA levels in the mouse liver, brain and kidneys were monitored using Glucometer® (Accu-Check® Advantage II Performa kits, Roche Diagnostics, Germany) and thiobarbituric acid substance assay, respectively. The expression of SOD and CAT mRNA were determined in the mouse liver and the activities of SOD and CAT enzymes were determined in mouse liver, brain and kidneys, respectively.
Results: Berberine exhibited similar hypoglycemic potential as glibenclamide to lower area under the curve of the fasting blood glucose. In DM type 2 mice, berberine increased the hepatic CuZn-SOD mRNA expression and the kidney SOD and CAT activities to normal levels. Moreover, DM-induced lipid peroxidation by increasing of MDA levels in both the liver and brain and lipid peroxidation status was restored by berberine.
Conclusion: Berberine possessed hypoglycemic properties and strong potential to improve the oxidant–antioxidant balance, though the combination treatment of berberine and glibenclamide did not show additional benefit over the treatment with berberine alone.
Introduction
Berberine () is a major plant alkaloid found in several Thai, Indian and Chinese herbal plants, i.e., Berberis aristata L. (Berberidaceae), Berberis vulgaris L. (Berberidaceae), Coptis chinensis Franch. (Ranunculaceae), Coptis japonica Makino (Ranunculaceae), Coscinium fenestratum Colebr. (Menispermaceae), Hydrastis Canadensis L. (Ranunculaceae) and Tinospora cordifolia Thunb. (Menispermaceae) (Piyanuch et al., Citation2006; Sato & Yamada, Citation1984). Extensive research has shown the pharmacological activities of berberine, in which the well known benefit of berberine is hypoglycemic action. Zhang et al. (Citation2008, Citation2011) reported that berberine at a daily dose of 1.0 g for 3 months was effective and safe for the treatment of diabetes mellitus (DM) and dyslipidemia in human. Chronic DM results in several complications such as hypertension, heart attack, kidney failure and finally, morbidity or premature death. Therefore, advanced research for exploring new strategies to improve DM status and quality of life in patients are still required.
Oxidative stress is a widely affirmed participant in the development and progression of DM (Maritim et al., Citation2003). The oxidative stress in DM may be induced by a continuing cycle of metabolic stress, tissue damage and cell death, leading to an increase in free radical production (Baynes, Citation1991). In addition, high blood glucose level causes the overproduction of reactive oxygen species (ROS) by the mitochondrial electron transport chain (Piconi et al., Citation2003). Such studies may lead to therapeutic approaches for limiting or delaying the damage from the oxidation reaction and for complementing existing therapies for treatment of the complications of DM (West, Citation2000). The free radical tissue defense enzyme, such as catalase (CAT) and superoxide dismutase (SOD), are important antioxidation mechanisms against ROS and particularly superoxide anion radicals in mammals (Morel & Barouki, Citation1999; Zelko et al., Citation2002).
Our previous studies revealed that berberine possessed hypoglycemic properties in noninsulin-dependent DM in ddY mice (DM type 2) compared to metformin, while the herb–drug interactions of berberine can be problematic (Chatuphonprasert et al., Citation2012). Moreover, berberine restored the levels of mRNA and enzyme activities of GPx, SOD and glutathione contents in the livers of DM type 2-induced mice. The study noted the potential of berberine as a hypoglycemic agent with the added benefit of an antioxidant effect (Lao-ong et al., Citation2012). However, the data of berberine on oxidative stress system in other organs and information of co-treatment with other drugs were not yet completed. Therefore, in this study, we provided information on the effects of berberine on antioxidation enzymes, namely CAT and SOD, and the level of malondialdehyde (MDA) in the livers and brains of streptozotocin–nicotinamide-induced DM type 2 ICR male mice, compared with a common hypoglycemic drug glibenclamide. The results convey a complement any benefit of berberine for treatment regimens of DM2 in the future.
Materials and methods
Materials
Berberine chloride (Ber) and streptozotocin (STZ) were supplied by Wako Pure Chemical Ind. (Osaka, Japan) and Merck (Damstadt, Germany), respectively. Glibenclamide (Gli), MDA, SOD, xanthine, xanthine oxidase, nitroblue tetrazolium (NBT) and ammonium molybdate were purchased from Sigma Chemical Co. (St. Louis, MO). ReverTraAce® and Taq DNA polymerase for reverse transcription and polymerase chain reaction were products of Toyobo Co., Ltd. (Osaka, Japan) and Invitrogen® (Life Technologies Corporation, Carlsbad, CA), respectively. Forward and reverse primers of CuZn-SOD, Mn-SOD, CAT and GAPDH genes were synthesized by Bio Basic, Inc. (Markham Ontario, Canada). The primers of each gene are shown in . All other laboratory chemicals were of the highest available purity from commercial suppliers.
Table 1. Primer sequences for PCR.
Animals
Male ICR mice at 6 weeks of age were supplied by the National Laboratory Animal Center, Mahidol University, Nakhonpathom, Thailand. All mice were housed in the Northeast Laboratory Animal Center, Khon Kaen University, Khon Kaen, Thailand, under the supervision of certified laboratory veterinarians and treated according to a research protocol approved by the Animal Ethics Committee for Use and Care of Khon Kaen University (Approval No. AEKKU06/2553).
Induction of type 2-diabetes in mice
Noninsulin-dependent diabetes or DM type 2 was induced by a single intraperitoneal injection of STZ in 0.05 M sodium citrate, pH 4.5, at a dose of 100 mg/kg at 15 min after a single intraperitoneal injection of nicotinamide in saline buffer at a dose of 1000 mg/kg (Matsuyama-Yokono et al., Citation2009). Two weeks after the induction, tail vein blood was collected to determine fasting blood glucose (FBG) level to identify oral glucose tolerance (Chatuphonprasert et al., Citation2012).
Oral glucose tolerance test
To assess oral glucose tolerance, mice were fasted overnight and the blood glucose response to the oral administration (by gavage) of a 20% glucose solution (2 g/kg) was determined. Tail vein blood samples were taken 30 min before (time −30) and at 0, 30, 60 and 120 min after the administration of glucose. Glucose concentrations and the area under the curve (AUC) were determined (Reed et al., Citation2000).
Experimental design
Male ICR mice at 6 weeks of age were mainly divided into two groups including normal and diabetic mice. Each major group was randomly divided into four groups including control, Gli-treated, Ber-treated and co-administration of Gli and Ber, respectively. Gli (10 mg/kg/d) and Ber (100 mg/kg/d) were dissolved in phosphate buffer saline (PBS) and given intragastrically to mice every day for 2 weeks. The control group was given 0.1 ml of PBS intragastrically every day to assure a nonsignificant change in the mRNA expression of the investigated genes or enzyme activities from the vehicle. The FBG level of all mice were monitored weekly before and after 2 weeks of the treatment using a glucometer (Accu-Check® Advantage II Performa kits, Roche Diagnostics, Germany) and mouse urine was checked using Pretest 7aII strips (Wako®, Osaka, Japan) to determine the diabetic condition (Sakuma et al., Citation2001). During fasting, the mice were deprived of food for 12 h but had free access to water. The mice were sacrificed at 24 h after the last treatment and the livers, brains and kidneys were excised immediately and kept at −80 °C for further analysis.
Expression of SOD and CAT mRNAs
Hepatic CuZn-SOD, Mn-SOD, CAT and GAPDH mRNAs were semi-quantified by RT–PCR. Hepatic total RNA was reverse-transcribed using ReverTraAce® reverse transcriptase and then the cDNA was amplified under the conditions recommended by the supplier of Invitrogen® (Life Technologies Corporation, Carlsbad, CA). The conditions of PCR cycle were followed by the method of Mouatassim et al. (Citation1999), Chatuphonprasert et al. (Citation2012) and Lao-ong et al. (Citation2012). After separation of the PCR products by 2% agarose gel electrophoresis, the target cDNA were detected under ultraviolet light in the presence of Novel Juice of GeneDirex® (Bio-Helix Co., Ltd., Taiwan) and semi-quantified by Syngene® gel documentation (Ingenius L, Cambridge, UK) and the GeneTools match program (Syngene®). The mRNA levels of the targets were normalized to that of GAPDH.
Determination of the thiobarbituric acid reactive substances content
Lipid peroxidation was determined by measuring formation of thiobarbituric acid reactive substances (TBARS) as described previously (Chatuphonprasert et al., Citation2011). In brief, a 500 µl aliquot of liver, brain or kidney homogenates was incubated at 37 °C for 1 h. Then, 1 ml of an equal volume of 40% (w/v) trichloroacetic acid (TCA) and 0.2% (w/v) thiobarbituric acid (TBA) was added. The reaction mixture was heated at 100 °C for 15 min, before it was immediately cooled down. The reaction was then stopped by 40% (w/v) TCA before being centrifuged at 3500 rpm at 4 °C for 5 min. The supernatant was used to measure TBARS formation by using spectrofluorometry with an excitation wavelength of 528 nm and an emission wavelength of 551 nm compared with a standard of MDA.
Determination of SOD activity
An aliquot of sample homogenate was extracted using chloroform and ethanol (3:5) and then centrifuged at 13 000 g at 4 °C for 30 min for further analysis (Chatuphonprasert et al., Citation2013). The supernatant was mixed with the reagent mixture (containing 1.2 mM xanthine, 140 mM ethylenediamine tetraacetic acid, 140 mM NBT, 56 mM sodium carbonate and 70 mg/ml BSA), followed by the xanthine oxidase solution (12.5 µU/ml). The reaction was incubated at 25 °C for 20 min and stopped by 0.1 mM copper chloride before measuring the production of formazan at a wavelength of 550 nm. The percentage of formazan inhibition was determined by comparing with the standard bovine CuZn-SOD.
Determination of CAT activity
CAT activity was determined using the method of Chatuphonprasert et al. (Citation2013). An aliquot of sample homogenate was incubated in 100 mM H2O2 substrate at 37 °C for 1 min. The enzymatic reaction was terminated by the addition of 16 mM ammonium molybdate followed by measurement of the yellow complex of molybdate and H2O2 at a wavelength of 405 nm. The percentage of inhibition of the yellow product forming was analyzed and compared with the standard hepatic bovine CAT.
Statistical analysis
The results were analyzed by one-way analysis of variance (ANOVA) followed by Tukey post hoc test using SPSS version 17.0 (SPSS Inc., Chicago, IL). Differences with p < 0.05 was considered to be statistically significant.
Results
Effect of berberine on the level of fasting blood glucose
The induction of DM type 2 in a mouse by the co-treatment of nicotinamide and streptozotocin was assured. Tail vein blood was collected before and every week after the induction to determine the FBG level by a glucometer, corresponding to the previous study (Matsuyama-Yokono et al., Citation2009). To confirm the potential of berberine to lower the blood glucose level of the DM type 2-induced mice, a glucose tolerance test was carried out. Two weeks after receiving berberine every day at a dose of 100 mg/kg/d, all mice were given a single dose of glucose solution (2 g/kg) intragastrically and the level of FBG was monitored over a 150 min period (). The success rate of STZ–nicotinamide-induced hyperglycemia was ∼70–80%. Hence, only mice with glucose tolerance status were included in the experiment. shows the AUC level of normal and diabetic mice after treatments. The STZ–nicotinamide-induced mice showed 2.5-times higher blood glucose AUC than the normal ones (p < 0.001). Glibenclamide, a common hypoglycemic drug, extensively suppressed the FBG level in the DM type 2-induced mice to nearly the same level as the normal ones ( and ). Berberine significantly lowered the level of FBG in the mice, though it was inferior to that of glibenclamide. The additional effect on the FBG level of the DM type 2-induced mice was not seen by co-treatment with berberine and glibenclamide. Neither berberine nor glibenclamide showed a negative effect on the blood glucose AUC level of the normal mice (). These observations supported the hypoglycemic potential of berberine on the DM type 2 (Lao-ong et al., Citation2012; Zhang et al., Citation2008, Citation2011).
Figure 2. Area under the curve of fasting blood glucose. (A) The tail vein-blood of all mice was collected at 30 min intervals over a 120 min period after a single intragastric administration of glucose solution at a dose of 2 g/kg for the glucose tolerance test to determine AUC of the fasting blood glucose using a glucometer. (B) The AUC of fasting blood glucose was calculated from 3–4 mice in the same group. A significant difference was determined by one-way ANOVA followed by Tukey post hoc test. *p < 0.01, **p < 0.001 versus NT-NT; #p < 0.01, ##p < 0.001 versus DM-NT.
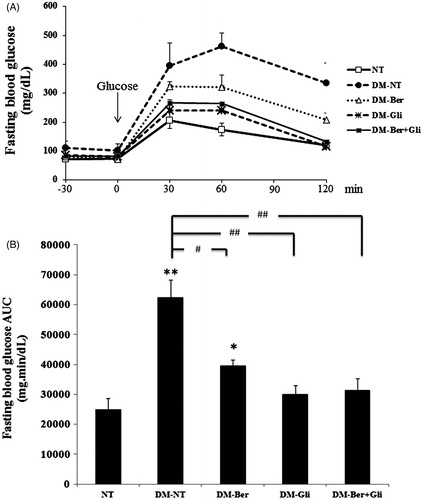
Table 2. The AUC level and blood glucose levels of normal and diabetic mice at 2 weeks after the treatments.
Modification of the hepatic mRNA expression of genes-related redox status in the DM type 2-induced mice by berberine and glibenclamide
To examine how berberine participated in the regulatory mechanism of gene-related redox status, the expression of CuZn-SOD, Mn-SOD and CAT mRNA were determined in the livers of the DM type 2-induced mice. The hepatic mRNA expression of all investigated genes was not changed by either berberine and/or glibenclamide in the normal mice (). DM significantly suppressed the expression of CuZn-SOD (p < 0.001) and CAT (p < 0.01) mRNAs with no change in Mn-SOD mRNA. Berberine restored the level of CuZn-SOD to nearly the same level as that in the normal, whereas glibenclamide did not (). The co-administration of berberine and glibenclamide resulted in a negative outcome on the level of CuZn-SOD mRNA (p < 0.001). The expression of Mn-SOD mRNA was not significantly affected by either the DM type 2 status or the treatments (). Neither berberine nor glibenclamide improved the expression of CAT mRNA ().
Figure 3. Expression of CuZn-SOD, Mn-SOD and CAT mRNA in mouse livers. Once a day, both the normal (NT) and the diabetic mice (DM) were given glibenclamide intragastrically (Gli, 10 mg/kg/d) and/or berberine (Ber, 100 mg/kg/d) or PBS (NT), respectively, for 2 weeks. Mice were sacrificed 24 h after the last treatment and the livers were immediately excised to prepare total RNA. Semi-quantitative determination of mRNA expression was performed using a pair of primers specific to the investigated genes as described. The data are presented as the mean ± SD (n = 5). A significant difference was determined by one-way ANOVA followed by Tukey post hoc test. *p < 0.01, **p < 0.001 versus NT-NT; #p < 0.01 versus DM-NT.
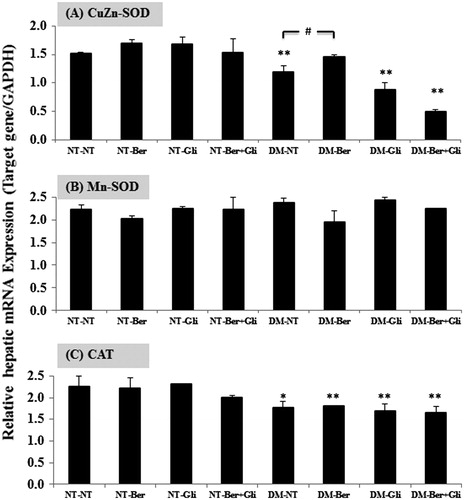
Decrease of MDA formation in the DM type 2-induced mice by berberine and glibenclamide
Formation of MDA from the breakdown of polyunsaturated fatty acids serves as a convenient index for determining the extent of lipid peroxidation reaction. The level of MDA was significantly increased in the livers and brains of the DM type 2 mice (p < 0.01) (), whereas those of the kidneys were not changed (). Interestingly, the single treatment of berberine or glibenclamide reduced the MDA formation in the livers and brains of the DM type 2 mice to comparable levels with the normal ones. In addition, the co-treatment of berberine and glibenclamide extensively lowered the levels of MDA in both the normal and the DM type 2 mice (p < 0.01).
Figure 4. Effect of berberine and/or glibenclamide on the level of TBARSs in mouse (A) liver (B) brain and (C) kidneys. Once a day, both the normal (NT) and the diabetic mice (DM) were given glibenclamide intragastrically (Gli, 10 mg/kg/d) and/or berberine (Ber, 100 mg/kg/d) or PBS (NT), respectively, for 2 weeks. Mice were sacrificed 24 h after the last treatment and the livers, brains and kidneys were immediately excised to examine the levels of TBARs as described. The data are presented as the mean ± SD (n = 5). A significant difference was determined by one-way ANOVA followed by Tukey post hoc test. *p < 0.01 versus NT-NT; #p < 0.01 versus DM-NT.
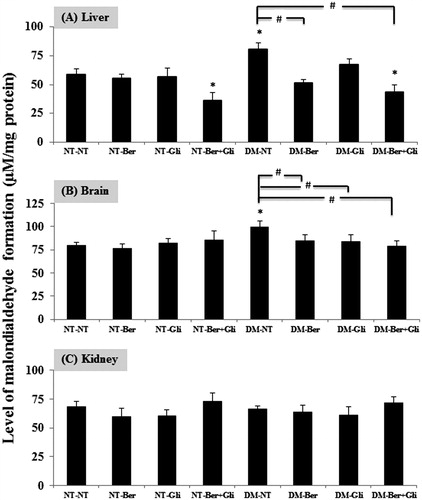
Improvement of SOD activity in the DM type 2-induced mice by berberine and glibenclamide
SOD activity was determined in mouse liver, brain and kidneys. The DM type 2 did not significantly change SOD activity in the liver, brain and kidneys. In the liver, there was no significant change of SOD activity by any treatment (). Berberine plus glibenclamide significantly enhanced the SOD activity in the brains of normal mice (p < 0.001) (). Both of the single glibenclamide treatment and the co-administration with berberine raised the SOD activity in the brain (p < 0.01) of the DM type 2 mice. In the kidneys, berberine and/or glibenclamide showed undesirable effects on the SOD activity in the normal (p < 0.001) and the DM type 2 mice (p < 0.001), except the berberine-treated DM type 2 mice ().
Figure 5. Effect of berberine and/or glibenclamide on the activities of SOD in the mouse liver (A), brain (B) and kidneys (C). Both the normal (NT) and the diabetic mice (DM) were daily given glibenclamide intragastrically (Gli; 10 mg/kg/d) and/or berberine (Ber, 100 mg/kg/d) or PBS (NT), respectively, for 2 weeks. Mice were sacrificed 24 h after the last treatment and the livers, brains and kidneys were immediately excised to examine the activity of SOD enzymes as described. The data are presented as the mean ± SD (n = 5). A significant difference was determined by one-way ANOVA followed by Tukey post hoc test. *p < 0.01, **p < 0.001 versus NT-NT; #p < 0.01, ##p < 0.001 versus DM-NT.
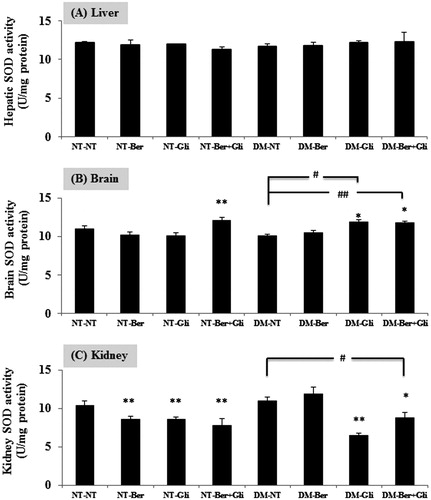
Improvement of CAT activity in the DM type 2-induced mice by berberine and glibenclamide
The DM type 2 status significantly reduced the hepatic CAT activity (p < 0.01) (). In the liver, varied results on the effect of berberine and/or glibenclamide on CAT activity were observed. Berberine decreased the CAT activity in the normal (p < 0.01), while it increased the CAT activity in the DM type 2 mice (p < 0.01). Glibenclamide significantly raised CAT activity both in the normal (p < 0.01) and the DM type 2 mice (p < 0.01, compared with the DM-NT). The co-treatment of berberine and glibenclamide suppressed CAT activity in the normal (p < 0.01), but it restored the CAT activity in the DM type 2 to the normal level. In the brain, the single treatment of glibenclamide or the co-treatment with berberine, significantly raised CAT activity in normal mice (p < 0.01) (). The DM type 2 did not change the level of CAT activity, but the single treatment of glibenclamide or berberine significantly increased the activity of CAT. In the kidneys, both berberine and glibenclamide brought undesirable effects on the CAT activity (p < 0.01; ).
Figure 6. Effect of berberine and/or glibenclamide on the activities of CAT in mouse liver (A), brain (B) and kidneys (C). Once a day, both the normal (NT) and the diabetic mice (DM) were given glibenclamide intragastrically (Gli, 10 mg/kg/d) and/or berberine (Ber, 100 mg/kg/d) or PBS (NT), respectively, for 2 weeks. Mice were sacrificed 24 h after the last treatment and the livers, brains and kidneys were immediately excised to examine the activity of CAT enzymes as described. The data are presented as the mean ± SD (n = 5). A significant difference was determined by one-way ANOVA followed by Tukey post hoc test. *p < 0.01 versus NT-NT; #p < 0.01 versus DM-NT.
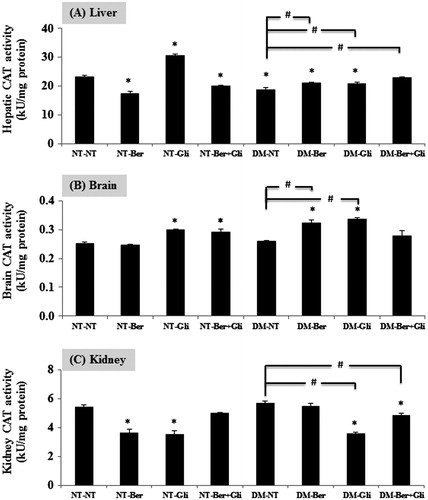
Discussion
DM type 2, affecting >90% of diabetic patients, is a heterogeneous and polygenic metabolic disease characterized by hyperglycemia and insulin resistance (Cavaghan et al., Citation2000). STZ induces diabetes by inhibition of insulin secretion and direct cytotoxic action on pancreatic β-cells (Szkudelski, Citation2001). In this study, the method of STZ–nicotinamide injection was used to induce the DM status in mice. Nicotinamide protected some β-cells. Therefore, intraperitoneal injection of STZ at a dose of 100 mg/kg at 15 min after a single intraperitoneal injection of nicotinamide in saline buffer at a dose of 1000 mg/kg introduced type 2 diabetic mice (Matsuyama-Yokono et al., Citation2009).
Berberine has been reported to improve glucose and lipid metabolic disorders by decreasing FBG levels (Tang et al., Citation2006). Intragastric administration of berberine at a dose of 100 mg/kg/d for 2 weeks or 250 mg/kg/d (orally) for 4 weeks, decreased the level of FBG in mice (Tang et al., Citation2006; Wang et al., Citation2010; Zhang et al., Citation2008). In this study, the DM type 2 mice that received berberine daily at a dose of 100 mg/kg/d for 2 weeks had the AUC level of FBG comparable with the glibenclamide-treated DM type 2 with the lowered of those than the non-treated DM type 2. These observations assured antihyperglycemic potentialities of berberine. The combination of berberine and glibenclamide did not show the additional effect on lowering blood glucose level.
Moreover, impact of berberine and glibenclamide on the oxidative stress pathway including regulatory expression of genes-related antioxidation were determined in the livers, the enriched organ of antioxidative enzymes. As expected, a decrease of CuZn-SOD directly involved in the pathogenic process of diabetes was observed (Likidlilid et al., Citation2007). The hepatic mRNA expression of CuZn-SOD and CAT were suppressed in the DM type 2 mice while that of Mn-SOD was not changed. The different changes of CuZn-SOD and Mn-SOD might be explainable by the different localization of these two enzymes. CuZn-SOD was located in the cytoplasmic compartment, whereas Mn-SOD was abundant in mitochondria (Zelko et al., Citation2002). Berberine improved the expression of CuZn-SOD mRNA but that of CAT was not affected by berberine. The combination of berberine and glibenclamide did not yield a benefit on the antioxidative enzyme-related genes, but an undesirable outcome on lowering the level of CuZn-SOD mRNA was observed. Although CuZn-SOD mRNA was decreased in the livers of the DM mice, a change of the SOD activity was not significantly noted. These phenomena might be explained in that the SOD activity represents activities of all types of SOD including CuZn-SOD, Mn-SOD and extracellular SOD.
The level of MDA was increased in the hepatic, pancreatic and renal tissues in alloxan-induced DM mice (Sharma & Garg, Citation2009). Corresponding to this study, MDA formation was increased in the livers and brains of STZ–nicotinatime-induced DM type 2 mice. Berberine and glibenclamide restored the MDA level to that of the normal level. The co-administration of berberine and glibenclamide exhibited an additional effect on decreasing MDA formation. In kidneys, berberine and glibenclamide decreased SOD activity, while berberine alone improved SOD activity in DM type 2 mice. A previous study by Liu et al. (Citation2008) revealed that berberine ameliorated renal injury in the STZ-induced DM rat by increasing SOD activity and decreasing MDA formation.
Hyperglycemia not only generates more ROS, such as superoxide and hydrogen peroxide, but also attenuates antioxidative mechanisms through glycation of the scavenging enzymes including SOD and CAT (Liu et al., Citation2008). The CAT activity in livers and kidneys were decreased in the STZ–nicotinamide-induced rats, and it was improved by berberine (Punitha et al., Citation2005). Accordingly, in this study, berberine improved CAT activities in the livers and brains of the DM type 2 mice.
Berberine exhibited positive effects on both hypoglycemic action and the oxidant–antioxidant balance in the DM type 2 mice, but the co-administration of berberine and glibenclamide did not yield superior benefits. These findings supported the use of berberine as an alternative antidiabetic compound in DM type 2, according to its hypoglycemic action and the added beneficial antioxidation potential.
Declaration of interest
The authors declare no conflicts of interest. The authors thank the Research Group for Pharmaceutical Activities of Natural Products using Pharmaceutical Biotechnology (PANPB), Faculty of Pharmaceutical Sciences, Khon Kaen University, Thailand, for the grant and facilities.
References
- Baynes JW. (1991). Role of oxidative stress in development of complications in diabetes. Diabetes 40:405–12
- Cavaghan MK, Ehrmann DA, Polonsky KS. (2000). Interactions between insulin resistance and insulin secretion in the development of glucose intolerance. J Clin Invest 106:329–33
- Chatuphonprasert W, Nemoto N, Sakuma T, Jarukamjorn K. (2012). Modulations of cytochrome P450 expression in diabetic mice by berberine. Chem Biol Interact 196:23–9
- Chatuphonprasert W, Sangkawat T, Nemoto N, Jarukamjorn K. (2011). Suppression of beta-naphthoflavone induced CYP1A expression and lipid-peroxidation by berberine. Fitoterapia 82:889–95
- Chatuphonprasert W, Udomsuk L, Monthakantirat O, et al. (2013). Effects of Pueraria mirifica and miroestrol on the antioxidation-related enzymes in ovariectomized mice. J Pharm Pharmacol 65:447–56
- Lao-ong T, Chatuphonprasert W, Nemoto N, Jarukamjorn K. (2012). Alteration of hepatic glutathione peroxidase and superoxide dismutase expression in streptozotocin-induced diabetic mice by berberine. Pharm Biol 50:1007–12
- Likidlilid A, Patchanans N, Poldee S, Peerapatdit T. (2007). Glutathione and glutathione peroxidase in type 1 diabetic patients. J Med Assoc Thailand 90:1759–67
- Liu W, Hei Z, Nie H, et al. (2008). Berberine ameliorates renal injury in streptozotocin-induced diabetic rats by suppression of both oxidative stress and aldose reductase. Chin Med J 121:706–12
- Maritim AC, Sanders RA, Watkins JB. (2003). Diabetes, oxidative stress, and antioxidants: A review. Biochem Mol Toxicol 17:24–38
- Matsuyama-Yokono A, Tahara A, Nakano R, et al. (2009). Antidiabetic effects of dipeptidyl peptidase-IV inhibitors and sulfonylureas in streptozotocin--nicotinamide-induced mildly diabetic mice. Metab Clin Exper 58:379–86
- Morel Y, Barouki R. (1999). Repression of gene expression by oxidative stress. Biochem J 342:481–96
- Mouatassim S, Guerin P, Menezo Y. (1999). Expression of genes encoding antioxidant enzymes in human and mouse oocytes during the final stages of maturation. Mol Human Reprod 5:720–5
- Piconi L, Quagliaro L, Ceriello A. (2003). Oxidative stress in diabetes. Clin Chem Lab Med 41: 1144–9
- Piyanuch P, Gritsanapan W, Suntornsuk L. (2006). Determination of berberine content in the stem extracts of Coscinium fenestratum by TLC densitometry. Med Princ Pract 15:373–8
- Punitha ISR, Shirwaikar A, Shirwaikar A. (2005). Antidiabetic activity of benzyl tetra isoquinoline alkaloid berberine in streptozotocin--nicotinamide induced type 2 diabetic rats. Diabetologia Croatica 34:117–28
- Reed MJ, Meszaros K, Entes LJ, et al. (2000). A new rat model of type 2 diabetes: The fat-fed, streptozotocin-treated rat. Metabolism 49:1390–4
- Sakuma T, Honnia R, Maguchi S, et al. (2001). Different expression of hepatic and renal cytochrome P450s between the streptozotocin induced diabetic mouse and rat. Xenobiotica 31:223–37
- Sato F, Yamada Y. (1984). High berberine-producing cultures of Coptis japonica cells. Phytochemistry 23:281–5
- Sharma N, Garg V. (2009). Antidiabetic and antioxidant potential of ethanolic extract of Butea monosperma leaves in alloxan-induced diabetic mice. Indian J Biochem Biophys 46:99–105
- Szkudelski T. (2001). The mechanism of alloxan and streptozotocin action in B cells of the rat pancreas. Physiol Res 50:536–46
- Tang LQ, Wei W, Chen LM, Liu S. (2006). Effects of berberine on diabetes induced by alloxan and a high-fat/high-cholesterol diet in rats. J Ethnopharmacol 108:109–15
- Wang Y, Campbell T, Perry B, et al. (2010). Hypoglycemic and insulin-sensitizing effects of berberine in high-fat diet- and streptozotocin-induced diabetic rats. Metabolism 60:298–305
- West IC. (2000). Radicals and oxidative stress in diabetes. Diabetes Med 17:171–80
- Zelko IN, Mariani TJ, Folz RJ. (2002). Superoxide dismutase multigene family: A comparison of the CuZn-Sod (Sod1), Mn-Sod (Sod2), and Ec-Sod (Sod3) gene structures, evolution, and expression. Free Radical Biol Med 33:337–49
- Zhang Y, Li X, Zou D, et al. (2008). Treatment of type 2 diabetes and dyslipidemia with the natural plant alkaloid berberine. J Clin Endocrinol Metab 93:2559–65
- Zhang Q, Xiao X, Feng K, et al. (2011). Berberine moderates glucose and lipid metabolism through multi pathway mechanism. Evid Based Complement Alternat Med 2011:1–10