Abstract
Context: Galbanic acid (GA) is a biologically active sesquiterpene coumarin from Ferula species (Apiaceae). This compound showed various biological properties including anticancer, cancer chemopreventive, anticoagulant, antiviral, and antileishmanial activities.
Objectives: This article is a review on the synthesis, biosynthesis, and biological activities of GA. In addition, we discussed gaps in our knowledge about GA that deserve future research. In this article, we also evaluated the drug-likeness of GA in silico.
Methods: All relevant databases were searched for the terms “galbanic acid”, “methyl galbanate”, “Ferula” and “sesquiterpene coumarin” without limitation, up to May 2013. Information on GA was collected via electronic search using Pubmed, Scopus, Web of Science, and Sciencedirect. All physicochemical parameters used for the prediction of drug-likeness were calculated using MarvinSketch (version 5.11, academic package, ChemAxon).
Results: This compound showed various biological properties including anticancer, cancer chemopreventive, anticoagulant, antiviral and antileishmanial activities. GA can inhibit the growth of prostate cancer cells via decreasing androgen receptor abundance. In silico physicochemical studies on GA showed that this compound has drug-like properties, the parameters that are important to predict potential of a compound for being a future drug.
Conclusion: GA is a valuable natural product with various biological activities. New discoveries with GA are to be expected.
Introduction
Galbanic acid (GA) and its ester, methyl galbanate, are natural products belonging to the class sesquiterpene coumarins () (Nazari & Iranshahi, Citation2011). Sesquiterpene coumarins mostly occur in the genus Ferula (Apiaceae), particularly F. galbaniflua Boiss. & Buhse, F. szowitsiana DC., and F. assa-foetida Boiss. & Buhse. These compounds have shown various biological activities including anti-inflammatory, antitumor, cytotoxic, and antiviral activities (Gliszczyńska & Brodelius, Citation2012; Iranshahy & Iranshahi, Citation2011; Nazari & Iranshahi, Citation2011). Among the sesquiterpene coumarins, GA has drawn special attention for its promising biological activities. For example, GA inhibits the growth of prostate cancer cells through decrease of androgen receptor (AR) abundance, a specific approach for inhibiting the growth of prostate cancer cells (Zhang et al., Citation2012). GA and methyl galbanate also showed antiviral activity against Influenza A virus (H1N1) (Lee et al., Citation2009). For these interesting biological properties (), researchers were encouraged to find the biosynthetic pathway of GA, and to synthesize it chemically.
Table 1. Tabulated overview of the biological activities of galbanic acid and methyl galbanate.
GA has three chiral centers in its structure. The determination of its absolute configuration was a topic of debate in recent years. The problem was that GA does not have a crystalline form, which provides a condition for an X-ray crystallographic analysis. However, researchers resolved this problem by X-ray crystallographic analysis of GA precursors (Bagirov et al., Citation1980; Borisov et al., Citation1975; Corbu et al., Citation2008; Lee et al., Citation1998).
The main objective of this review is to focus on the synthesis and biological activities of GA. Regardless of biological activities, our in silico studies on GA showed that GA possessed drug-like physicochemical properties, which might encourage medicinal chemists for preparation of its derivatives.
Chemistry
Chemical structure
The chemical structure of GA was a subject of debate during the last decades. Kunz et al. (Citation1937) were the first group to report GA from galbanum resin. In 1955, Pigulevskii et al. ascribed the structure 1a for GA (Bagirov et al., Citation1980; Pigulevskii & Naugol'naya, Citation1955) (). In 1973, Borisov et al. (Citation1975) revised the structure of GA as 1b on the basis of 1H-NMR and mass spectra. Later, Bagirov et al. (Citation1980) proposed a different structure (1c) for GA, as shown in . In the proposed structure by Bagirov et al. (Citation1980), the position of double bond and arrangements of methyl groups in the sesquiterpene part of GA structure differed from the previous ones. After that, Lee et al. (Citation1998) proposed a different stereochemistry for GA on the basis of 2D NMR techniques (1d). Ultimately, unambiguous structure elucidation of GA was carried out by Corbu et al. (Citation2008). They synthesized GA and determined absolute configuration at C10 by X-ray crystallographic analyses of the precursors. They confirmed the structure and the stereochemistry of GA (1c) reported by Bagirov et al. (Citation1980) (Corbu et al., Citation2008).
Biosynthesis of GA
Two distinct hypotheses for biosynthesis of GA (1) have been proposed by Appendino and Marner (). Appendino et al. (Citation1993) assumed the biosynthesis of GA from mogoltadone (2) through the reduction of the double bound, migration of the methyl group and subsequent cleavage of the cyclohexanone ring in mogoltadone. Marner and Kasel (Citation1995) assumed a different biosynthetic pathway through epoxide derivative of farnesyl diphosphate and further cyclization reactions (). Xiong et al. (Citation2006) proved Marner’s proposal using an Arabidopsis oxidosqualene cyclase enzyme. However, further investigations are necessary to unravel all aspects of the plant metabolism of GA including involved enzymes and gene clusters.
Total synthesis of GA
To the best of our knowledge, Corbu et al. (Citation2008) were the only group that studied the total synthesis of GA.
They synthesized GA from commercially available R-pulegone and 7-hydroxycoumarin (). The first step of synthesis was installation of methyl and ester at C9 position of R-pulegone by enolate formation. Reduction of the carbonyl moieties in 4 by LiAlH4 led to 5a and 5b. 6a and 6b were obtained by rearrangement of 5a in AcOH. Protection of OH at C11 with tert-butyldimethylsilyl (TBS) followed by a further Claisen rearrangement at 190 °C, gave 8. A Wittig reaction followed by oxidation of methoxy group by pyridinium chlorochromate (PCC) led to 10. Two remaining steps were desilylation of OH group at C11 and addition of umbelliferone to form methyl galbanate (12). Methyl galbanate was then saponified with LiOH to obtain GA (Corbu et al., Citation2009).
Figure 5. Synthesis of galbanic acid from 7-hydroxycoumarin (umbelliferone) and R-pulegone. Lithium diisopropyl amine, LDA; Tertiary butyldimethylsilyl chloride, TBSCl; Pyridium chlorochromate, PCC; p-Toluenesulfonyl chloride, p-TSCL; Microwave-assisted, MW.
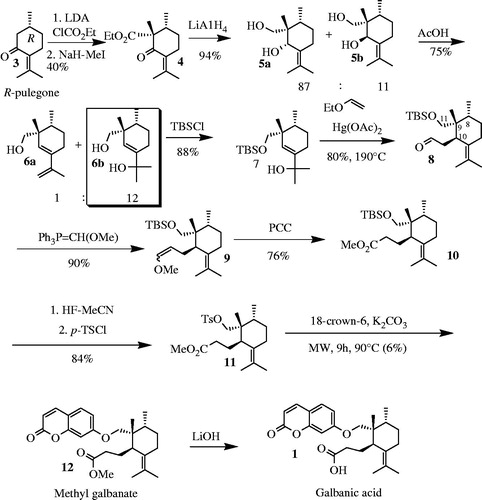
The main drawbacks of this synthesis pathway were inefficient formation of quaternary carbon center at C9 position (the first two steps of synthesis) and inefficient coupling of umbelliferone and 11.
Hence, Corbu et al. (Citation2009) revised their synthesis pathway () using ethyl cyanoformate instead of ethyl chloroformate that effectively increased the yield of reaction from 40 to 80%. They also used microwave-assisted coupling of umbelliferone and 11 applying the Mitsunobu etherification condition, which again increased the yield of reaction from 6 to 28%.
Photo-oxidative degradation of linear low-density (LLDPE) films by GA
LLDPE, a kind of polyethylene polymer, is commercially used for packaging. Investigations of photo-oxidative degradation of LLDPE films containing various amounts of GA revealed that GA was highly effective in accelerating photo-oxidation of LLDPE films. Jahanmardi and Assempour (Citation2008a) reported that an outstanding acceleration of photo-oxidation observed for the film containing 0.2% wt of GA in comparison with the film containing the same amount of benzophenone. Also, GA was known as an effective photo-sensitizing agent in polyethylene. Shahmir and Assempour (Citation2012) studied photo-oxidative degradation of LLDPE films in the presence of varying amounts of GA ferric salt (Fe-GA). Results showed that the photo-activity of GA in the polymer arises from the C=C bond presents in the side group attached to aromatic lactone rings of additive molecule. The other work by Jahanmardi and Assempour (Citation2008b) was carried out to study the effect of GA on the thermal and thermo-oxidative stabilities of LLDPE. Their results showed that the thermo-oxidative stabilizing activity of GA was related to the activity of allylic hydrogens present in the substituted moieties of GA molecules (Jahanmardi and Assempour, Citation2008b).
Biological activities
Anticoagulant activity
In 1988, Mansurov et al. studied the effect of GA sodium salt on platelet aggregation. They found that GA at concentrations of 50, 100, 200 µg/mL suppressed the development of platelet aggregation induced by ADP, thrombin, histamine, adrenaline, acetylcholine and collagen. Later in 1990, they showed that intravenous administration of GA, before bloodletting, prevented the development of experimental erythrocyte hyperaggregation (Mansurov & Martirosov, Citation1988, Citation1990). GA and some of its derivatives have hepatoprotective and anticoagulant properties (Grabley & Thiericke, Citation1999). Martirosov (Citation1986) found that the sodium salt of GA changed thrombocyte aggregation.
Mansurov and Martirosov (Citation1986) showed that the sodium salt of GA decreased erythrocyte aggregation both in vitro and in vivo at a single intravenous and repeated oral administration. Later on, Mansurov and Martirosov (Citation1990), showed that the sodium salt of GA prevents the development of erythrocyte hyperaggregation induced by ATP, thrombin, histamine, adrenaline and acetylcholine during in vitro experiments.
Acetylcholinesterase inhibitory activity
Inhibition of acetylcholinesterase is currently regarded as the leading strategy against Alzheimer’s disease. Karimi et al. (Citation2010) screened the in vitro inhibitory activity of eight naturally occuring terpenoid and coumarin derivatives (auraptene, diversin, diversolide D, farnesiferol A, GA, tschimgine, umbelliferone and umbelliprenin) from Ferula species at 100 µM concentration against human erythrocyte acetycholinesterase. Among the eight tested compounds, tschimgine, a monoterpene derivative, was the most potent inhibitor of acetylcholinesterase (inhibition 63.5%). GA inhibited the enzyme activity by 19.1%.
Anticancer and cancer chemopreventive properties
Recently, Zhang et al. (Citation2012) showed that GA decreased AR abundance and signaling and induced G1 arrest in prostate cancer cells. AR plays an important role in the progression of castration-resistant prostate cancer, which is relatively resistant to current chemotherapeutic agents. In addition, considering the resistance to current AR antagonists, there is a need to find alternative strategies for inhibiting AR signaling. GA has been demonstrated to exert anti-androgen and antineoplastic activities by the mentioned mechanism. Zhang et al. (Citation2012) reported that GA down-regulated AR protein abundance and signaling in LNCaP cells through promoting proteosomal degradation, which is a distinct mechanism from AR antagonists including bicalutamide. They also showed that GA induced G1 arrest in association with an inhibition of cyclin/CDK4/6/RB/E2F pathway (Zhang et al., Citation2012).
GA was also exhibited cancer chemopreventive activity. Iranshahi et al. (Citation2008) reported the inhibitory activity of nine sesquiterpene coumarins including GA and methyl galbanate isolated from different species of genus Ferula on the activation of Epstein-Barr Virus Early Antigen (EBV-EA) induced by 12-O-tetradecanoylphorbol 13-acetate (TPA) in Raji cells. IC50 values of GA (IC50 11.8 nM) and methyl galbanate (IC50 11.7 nM) were comparable to β-carotene (IC50 10.4 nM) and curcumin (IC50 8.3 nM) as positive controls. These studies revealed that GA exhibited significantly both anticancer and cancer chemopreventive activities.
Recently, it was revealed that GA also exerted its anticancer activities by inhibiting angiogenesis, the essential process for tumor growth and metastasis. GA significantly decreased vascular endothelial growth factor (VEGF)-induced proliferation and inhibited VEGF-induced migration and tube formation of human umbilical vein endothelial cells (HUVECs). These effects were accompanied by decreased phosphorylation of p38-mitogen-activated protein kinase (MAPK), c-Jun N-terminal kinase (JNK), and AKT, and decreased expression of VEGFR targets endothelial nitric oxide synthase (eNOS) and cyclin D1 in VEGF-treated HUVECs (Kim et al., Citation2011).
Overexpression of a plasma membrane protein ATP-binding cassette transporter 1 (ABCB1) or P-glycoprotein (P-gp) is one of the major resistance mechanisms in cancer cells. P-gp is responsible for decreased drug accumulation in multidrug-resistant cells and often mediated the development of resistance to anticancer drugs. It has been shown that GA possessed a potential to inhibit the activity of this protein. Hanafi-Bojd et al. (Citation2011) have recently studied the effect of GA on P-glycoprotein-mediated rhodamine efflux in breast cancer cell lines. Their results showed that GA at a concentration of 5 μg/mL inhibited P-gp activity higher than that of verapamil (5 μg/mL), the well-known inhibitor of P-gp (Hanafi-Bojd et al., Citation2011).
Farnesyl protein transferase (FTase) is an essential enzyme for tumor growth in some cancers including pancreas and colon cancers. Inhibiting this enzyme might be a specific target for cancer therapy. In a study, GA showed a promising inhibitory activity against this enzyme (Cha et al., Citation2011). The 50% inhibitory concentration (IC50) of GA against FTase in an enzyme-based assay was calculated as 2.5 µM. GA was also demonstrated to be a potent inhibitor of the proliferation of oncogenic ras-transformed NIH3T3/Hras-F in a dose-dependent manner. The effect of GA on the proliferation of oncogenic ras-transformed NIH3T3/Hras-F cells was calculated with an IC50 value of 16.2 µM, whereas its IC50 value on control vector-transfected normal ras-containing NIH3T3/ZIPneo cells was calculated as 58.5 µM (Cha et al., Citation2011).
Antimicrobial properties
GA by itself did not show a significant antimicrobial activity against those microbes that have been tested yet. However, GA possessed a potential to increase antibacterial activities of other antibiotics, particularly against resistant strains. In 2007, Shahverdi et al. reported that GA enhanced the antibacterial activity of penicillin G and cephalexin against Staphylococcus aureus. They carried out disk diffusion and broth dilution methods to determine the antibacterial activity of the mentioned antibiotics. Their results showed that in sub-inhibitory concentration of GA (100 µg/mL), the MIC value of penicillin G for S. aureus was reduced from 64 µg/mL in the absence of GA to 1 µg/mL in the presence of GA, while that of cephalexin decreased from 128 µg/mL in the absence of GA to 1 µg/mL in the presence of GA. Their results suggested that GA may act as an effective adjuvant in the chemotherapy of infectious diseases (Shahverdi et al., Citation2007). Later on in 2009, Fazly-Bazzaz et al. announced the enhancing effects of GA on isolates of S. aureus, which were clinically resistant to methicillin, tetracycline and ciprofloxacin. GA at 400 µg/mL reduced the MICs of methicillin, tetracycline and ciprofloxacin, from 10 to 80, 40 to 80 and 10 to 20 µg/mL, to less than 1.25 µg/mL, respectively. They also demonstrated that the observed effect was only due to the combination effect of GA and antibiotics (Fazly-Bazzaz et al., Citation2009). In addition, GA was evaluated for its effects on the reversal of multidrug resistance in clinical isolates of S. aureus and Escherichia coli. GA (up to 1000 µg/mL) by itself did not show antibacterial activity against tested strains. The minimum inhibitory concentrations (MICs) of ciprofloxacin and tetracycline were determined using macrodilution technique in the presence and absence of subinhibitory concentrations of GA (31.25–1000 µg/mL). However, it caused no change in MICs of the antibiotics against E. coli (Fazly-Bazzaz et al., Citation2010).
The authors also investigated antileishmanial activity of GA together with other terpenoids from Ferula szowitsiana. Our findings revealed that both umbelliprenin and GA inhibited the growth of Leishmania major promastigotes. Umbelliprenin and GA were found to have inhibitory activities against promastigotes of L. major after an incubation of 48 h. GA, which accounts for 44.4% of the total extract, showed a weak inhibitory activity against promastigotes with an IC50 value of 164.8 µM (Iranshahi et al., Citation2007).
There are a few reports on antiviral properties of sesquiterpene coumarins including GA and methyl galbanate. In 2009, Lee et al. studied antiviral activities of GA, methyl galbanate and nine other sesquiterpene coumarins isolated from an extract of Ferula assa-foetida against influenza A virus. The tested compounds showed considerable antiviral activities. IC50 and IC90 values of GA were determined to be 0.45 and 0.88 µg/mL, respectively. In addition, IC50 and IC90 values of methyl galbanate were determined to be 0.26 and 0.44 µg/mL, respectively. These potencies were comparable to those of amantadine (IC50 0.92 and IC90 1.73 µg/mL) as an antiviral standard. However, methyl galbanate showed higher antiviral activity than GA against influenza A virus (Zhang et al., Citation2012).
Inhibitors of the human rhinovirus (HRV) coat protein are promising candidates to treat and prevent a number of upper respiratory diseases. Rollinger et al. (Citation2008) designed a study to find antiviral compounds from nature, focusing on the HRV coat protein. Through computational structure-based screening of an in-house 3D database containing 9676 individual plant metabolites from ancient herbal medicines, combined with knowledge from traditional use, they reached a conclusion that some sesquiterpene coumarins from asafoetida including farnesiferol C, farnesiferol B, and kellerin had promising antiviral activities (Rollinger et al., Citation2008). GA was not tested in that study.
Miscellaneous activities
In 1990, Syrov et al. reported that orally administration of GA, isolated from the roots of Ferula kopetdaghensis, to rats in a dose of 50 mg/kg improved the course of toxic hepatitis induced by four-fold subcutaneous injections of a 50% oil solution of CCl4. GA produced much earlier normalization of the activity of the enzymes alanine and aspartate aminotransferase in blood serum, increased glycogen content and improved the parameters of the redox potential of lactic acid--pyruvic acid system in the liver, as compared with control (Syrov et al., 1990).
Several natural and synthetic compounds which exert anti-inflammatory effects by inhibiting nitric oxide (NO) production have been reported to date. In 2011, Kohno et al. reported that methyl galbanate significantly decreased NO production in LPS/IFN-c-stimulated RAW264.7 cells at a concentration of 10 µM, but GA at the same concentration did not show any effect. In the presence of methyl galbanate, LPS/IFN-c-induced iNOS mRNA expression significantly decreased to 52% of the level found with LPS/IFN-c stimulation alone. In addition, methyl galbanate slightly attenuated COX-2 mRNA expression. Their findings revealed that the membrane permeability of methyl galbanate was higher than that of GA due to the presence of a hydrophobic methyl-esterified group (Kohno et al., Citation2011).
Drug-like physicochemical properties of GA
In today’s era of drug development, a large number of bioactive compounds are discovered from nature or combinatorial synthesis by high throughput screening, but only a few bioactive compounds reach the phases clinical trials, mostly because, the lack of solubility and permeability in aqueous media under thermodynamic equilibrating conditions. In recent years, a number of parameters have been introduced for the prediction of drug-like physicochemical properties of drug candidates including bioavailability, intestinal absorption and cell penetration (Hann & Oprea, Citation2004; Quinn et al., Citation2008). In this regard, the most well-known rule is “rule of five” or Lipinski’s rule. According to this rule, an oral drug-like molecule should have an octanol/water partition coefficient (Log P) of <5, <10 hydrogen bond donors (HBD), <5 hydrogen bond acceptors (HBA) and a molecular weight of <500 Da (Lipinski et al., Citation2001). Two more requirements for drug-like molecules, raised up by Veber et al. (Citation2002) include NROT (number of rotatable bonds) of <10 and a PSA (polar surface area) of <140 Å2. With respect to this description, we evaluated the above parameters for GA, GA sodium salt and methyl galbanate to see their eligibility for being future drugs. Interestingly, GA, GA sodium salt and methyl galbanate satisfied these criteria (). The drug-like properties of these three compounds guarantee that they will show acceptable ADME (absorption, distribution, metabolism and excretion) and translate effectively into clinical trials. It seems that GA can translate into clinical trial if it shows safety/efficacy in in vivo studies.
Table 2. In silico physico-chemical properties of galbanic acid, galbanic acid sodium salt and methyl galbanate.
Conclusion and future perspectives
GA is a biologically active sesquiterpene coumarin from Ferula species. This compound showed various biological activities including antiviral, cytotoxic, cancer chemopreventive and anticoagulant properties. F. assa-foetida and F. szowitsiana are rich sources of GA. For instance, we isolated and purified almost 5 g of GA from 250 g of the dried roots of F. szowitsiana (yield 2%, w/w) (Iranshahi et al., Citation2007).
Considering the limitation of natural sources including wild plants, chemical synthesis strategy of bioactive natural products was required to solve the supply problem and provide sufficient amounts for extensive biological testing, including mechanism and target identification studies, in vivo studies and clinical trials. Due to the structural simplicity of GA, as reviewed in this article, total synthesis of GA is practical in few steps (Corbu et al., Citation2008). Regarding the availability of GA, it seems there is no the supply problem for future researches including in vivo studies.
There still remains a number of questions regarding the biosynthetic pathway, molecular mechanisms and targets, and toxicity of GA. Due to the multiple targets of GA in the field of cancer, including antiangiogenic activity, P-gp inhibitiory effect and inducing apoptosis via decreasing AR abundance in AR (+) prostate cancer cells, exploring our knowledge of the anticancer activities of GA and their analogues can be a topic of research in the future.
Clearly, GA has inspired multidisciplinary research and continues to do so. Undoubtedly, new discoveries with GA are to be expected.
Declaration of interest
The authors report no conflicts of interests. The authors alone are responsible for the content and writing of this article.
Acknowledgements
The authors thank Dr. Seyed Ahmad Emami and Dr. Javad Asili for their kind support.
References
- Appendino G, Tagliapietra S, Mario GN, Jakupovic J. (1993). Sesquiterpene coumarin ethers from asafetida. Phytochemistry 35:183–6
- Bagirov VY, Sheichenko VI, Veselovskaya NV, et al. (1980). Structure and stereochemistry of galbanic acid. Chem Nat Compd 16:439–41
- Borisov VN, Ban'kovskii AI, Sheichenko VI, et al. (1975). The structure of galbanic acid. Chem Nat Compd 9:400–1
- Cha MR, Choi YH, Choi CW, et al. (2011). Galbanic acid, a cytotoxic sesquiterpene from the gum resin of Ferula asafoetida, blocks protein farnesyltransferase. Planta Med 77:52–4
- Corbu A, Aquino M, Perez M, et al. (2009). Natural and unnatural A-seco terpenes from pulegone: Synthesis of galbanic acid and marneral revisited. Eur J Org Chem 36:6386–92
- Corbu A, Perez M, Aquino M, et al. (2008). Total synthesis and structural confirmation of ent-galbanic acid and marneral. Org Lett 10:2853–6
- Fazly-Bazzaz BS, Du AR, Iranshahi M, et al. (2009). Evaluating the potentiating effect of galbanic acid from Ferula szowitsiana on three common antibiotics against resistant hospital isolates of Staphylococcus aureus. Iran J Pharm Res 8:217–21
- Fazly-Bazzaz BS, Memariani Z, Khashiarmanesh Z, et al. (2010). Effect of galbanic acid, a sesquiterpene coumarin from Ferula szowitsiana, as an inhibitor of efflux mechanism in resistant clinical isolates of Staphylococcus aureus. Braz J Microbiol 41:574–80
- Gliszczyńska A, Brodelius PE. (2012). Sesquiterpene coumarins. Phytochem Rev 11:77–96
- Grabley S, Thiericke R. (1999). Drug Discovery from Nature. Heidelberg, Germany: Springer-Verlag
- Hanafi-Bojd MY, Iranshahi M, Mosaffa F, et al. (2011). Farnesiferol A from Ferula persica and galbanic acid from Ferula szowitsiana inhibit P-glycoprotein-mediated rhodamine efflux in breast cancer cell lines. Planta Med 77:1590–3
- Hann MM, Oprea TI. (2004). Pursuing the leadlikeness concept in pharmaceutical research. Curr Opin Chem Biol 8:255–63
- Iranshahi M, Arfa P, Ramezani M, et al. (2007). Sesquiterpene coumarins from Ferula szowitsiana and in vitro antileishmanial activity of 7-prenyloxycoumarins against promastigotes. Phytochemistry 68:554–61
- Iranshahy M, Iranshahi M. (2011). Traditional uses, phytochemistry and pharmacology of asafoetida (Ferula assa-foetida oleo-gum-resin) -- A review. J Ethnopharmacol 134:1–10
- Iranshahi M, Kalategi F, Rezaee R, et al. (2008). Cancer chemopreventive activity of terpenoid coumarins from Ferula species. Planta Med 74:147–50
- Jahanmardi R, Assempour H. (2008a). Study on the effect of galbanic acid on photo-oxidative degradation of linear low density polyethylene (LLDPE) films. E-Polymers 30:1–11
- Jahanmardi R, Assempour H. (2008b). Effects of galbanic acid on thermal and thermo-oxidative stabilities of LLDPE. Iran Polym J 17:799–806
- Karimi G, Iranshahi M, Hosseinalizadeh F, et al. (2010). Screening of acetylcholinesterase inhibitory activity of terpenoid and coumarin derivatives from the genus Ferula. Pharmacologyonline 1:566–74
- Kim KH, Lee HJ, Jeong SJ, et al. (2011). Galbanic acid isolated from Ferula assafoetida exerts in vivo anti-tumor activity in association with anti-angiogenesis and anti-proliferation. Pharm Res 28:597–609
- Kohno S, Murata T, Sugiura A, et al. (2011). Methyl galbanate, a novel inhibitor of nitric oxide production in mouse macrophage RAW264.7 cells. J Nat Med 65:353–9
- Kunz K, Woldicke E. (1937). About the resin components of Galbanums. Chem Ber 70:359--67
- Lee CL, Chiang LC, Cheng LH, et al. (2009). Influenza A (H 1N 1) antiviral and cytotoxic agents from Ferula assa-foetida. J Nat Prod 72:1568–72
- Lee SG, Ryu SY, Ahn JW. (1998). Re-investigation of the structure of galbanic acid by 2D NMR techniques including 2D inadequate. B Korean Chem Soc 19:384–5
- Lipinski CA, Lombardo F, Dominy BW, Feeney PJ. (2001). Experimental and computational approaches to estimate solubility and permeability in drug discovery and development settings. Adv Drug Deliv Rev 46:3–26
- Mansurov MM, Martirosov MS. (1986). Effect of the sodium salt of galbanic acid on erythrocyte aggregation in an experiment. Farmakol Toksikol 49:19–20
- Mansurov MM, Martirosov MS. (1988). Effect of sodium salt of galbanic acid on platelet aggregation. Farmakol Toksikol 51:47–8
- Mansurov MM, Martirosov MS. (1990). Effect of a sodium salt of galbanic acid during experimental erythrocyte hyperaggregation. Farmakol Toksikol 53:51–3
- Marner FJ, Kasel T. (1995). Biomimetic synthesis of the iridal skeleton. J Nat Prod 58:319–23
- Martirosov MS. (1986). Changes in thrombocyte aggregation as affected by the sodium salt of galbanic acid in an experiment. Farmakol Toksikol 49:49–51
- Nazari ZE, Iranshahi M. (2011). Biologically active sesquiterpene coumarins from Ferula species. Phytother Res 25:315–23
- Pigulevskii GV, Naugol'naya TN. (1955). Resin from the roots of Ferula gummosa. Trudy Bot Inst Akad Nauk SSR 5:80
- Quinn RJ, Carroll AR, Pham NB, et al. (2008). Developing a drug-like natural product library. J Nat Prod 71:464–8
- Rollinger JM, Steindl TMB, Schuster DB, et al. (2008). Structure-based virtual screening for the discovery of natural inhibitors for human rhinovirus coat protein. J Med Chem 51:842–51
- Shahmir MA, Assempour H. (2012). Comparative study on the effects of galbanic acid, galbanic ferric salt and tetrabromo galbanic acid on photo-oxidative degradation of linear low density (LLDPE) films. E-Polymers 22:1–8
- Shahverdi AR, Fakhimi A, Zarrini G, et al. (2007). Galbanic acid from Ferula szowitsiana enhanced the antibacterial activity of penicillin G and cephalexin against Staphylococcus aureus. Biol Pharm Bull 30:1805–7
- Syrov VN, Khushbaktova ZA, Nabiyev AN. (1990). Effect of galbanic acid on the course of experimental hepatitis. Farmakol Toksikol 53:41–3
- Veber DF, Johnson SR, Cheng HY, et al. (2002). Molecular properties that influence the oral bioavailability of drug candidates. J Med Chem 45:2615–23
- Xiong Q, Wilson WK, Matsuda SPT. (2006). An Arabidopsis oxidosqualene cyclase catalyzes iridal skeleton formation by grob fragmentation. Angew Chem Int Edit 45:1285–8
- Zhang Y, Kim KH, Zhang W, et al. (2012). Galbanic acid decreases androgen receptor abundance and signaling and induces G 1 arrest in prostate cancer cells. Int J Cancer 130:200–12