Abstract
Context: Altered vitamin B12 levels have been correlated with hepatotoxicity; however, further evidence is required to establish its protective role.
Objective: To evaluate the effects of vitamin B12 supplement in protecting N′-nitrosodimethylamine (NDMA)-induced hepatic fibrosis in Wistar rats.
Materials and methods: Hepatic fibrosis was induced by administering NDMA in doses of 10 mg/kg body weight thrice a week for 21 days. Another group received equal doses (10 mg/kg body weight) of vitamin B12 subsequent to NDMA treatment. Animals from either group were sacrificed weekly from the start of the treatment along with their respective controls. Progression of hepatic fibrosis, in addition to the effect of vitamin B12, was assessed biochemically for liver function biomarkers, liver glycogen, hydroxyproline (HP) and B12 reserves along with histopathologically by hematoxylin and eosin (H & E) as well immunohistochemical staining for α-SMA expression.
Results and discussion: Elevation in the levels of aminotransferases, SALP, total bilirubin and HP was observed in NDMA treated rats, which was concomitant with remarkable depletion in liver glycogen and B12 reserves (p < 0.05). Liver biopsies also demonstrated disrupted lobular architecture, collagen amassing and intense fibrosis by NDMA treatment. Immunohistochemical staining showed the presence of activated stellate cells that was dramatically increased up to day 21 in fibrotic rats. Following vitamin B12 treatment, liver function biomarkers, glycogen contents and hepatic vitamin B12 reserves were restored in fibrotic rats, significantly. Vitamin B12 administration also facilitated restoration of normal liver architecture.
Conclusion: These findings provide interesting new evidence in favor of protective role for vitamin B12 against NDMA-induced hepatic fibrosis in rats.
Introduction
Hepatic fibrosis is a dynamic pathological condition that includes a series of biochemical and histological changes in liver due to insults caused by viral and parasitic infections or exposure to drugs and toxins (Ahmad & Ahmad, Citation2012). The diagnostic features of hepatic fibrosis are irregular proliferation and deposition of types I, III and IV collagen-rich tough fibrous connective tissue that creates an inequity between synthesis and degradation of extracellular matrix (ECM) within liver (Friedman, Citation2010; Lotersztajin, Citation2005; Wells, Citation2005). Subsequently, inflammatory immune cells are stimulated to secrete cytokines, growth factors and other reactive molecules to activate quiescent hepatic stellate cells (HSCs). Upon adopting myofibroblast-like phenotype, activated HSCs become proliferative, fibrogenic and start expressing α-smooth muscle actin (α-SMA).
Under laboratory conditions, a number of chemical compounds such as CCl4, thioacetamide and N′-nitrosodiethylamine (NDEA) induce hepatic fibrosis (Bruck et al., Citation2001; Eidi et al., Citation2013; George et al., Citation2001; Krajka-Kuzniak et al., Citation2012). However, N′-nitrosodimethylamine (NDMA) has the advantage of inducing fibrosis within shortest duration of 21 days, with lesser animal mortality and better reproducibility (George & Chandrakasan, Citation1996). Induction of hepatic fibrosis and tumorigenesis in rats by NDMA occur via the activation of CYP 450 enzymes (George et al., Citation2001; Kasprzyk-Hordern et al., Citation2005), which adversely affects biochemical and molecular parameters defining liver function (Ahmad et al., Citation2009; George & Chandrakasan, Citation2000; Krajka-Kuzniak et al., Citation2012). Changes in fibrotic liver also include modulation in functions or levels of vitamins, which are important participants during recovery of liver from diseased conditions (Chattopadhyay et al., Citation2012; Chung et al., Citation2010; Himmerich et al., Citation2001). While several published reports dealing with the effects of vitamins C and E in recovery of hepatic disorders are available (Harrison et al., Citation2003; Kugelmas et al., Citation2003), the role of vitamin B12 in alleviating fibrosis is still being debated and needs additional evidence.
Since liver is the functional, storage and transportation site of vitamin B12 (Soda et al., Citation1985), it is reasonable to speculate that liver pathology may also be associated with the changes in intrinsic concentration of vitamin B12. There already exists some evidence that attributes a mitigating role to vitamin B12 in liver disorders by way of preserving the appropriate biochemical ambience as well as the integrity of lobular architecture (Baker et al., Citation1998; Birch et al., Citation2009; Chattopadhyay et al., Citation2012; Ermens et al., Citation2003; Isoda et al., Citation2008; Krautler, Citation2005). Therefore, we undertook the present study on biochemical, histological and immunohistological aspects with the focus on the role of vitamin B12 in NDMA-induced fibrotic rats. Based on our findings, we hereby propose a plausible protection mechanism by which this vitamin appears to alleviate fibrosis.
Materials and methods
Animals
Adult, 6–8 weeks old and healthy male albino rats (Rattus norvegicus) of Wistar strain weighing around 140 ± 10 g were used in the present study. The rats were maintained at 12 h light:dark cycle in animal house facility of the department. They were kept in polycarbonate cages with wire mesh top and a bed of husk under proper hygienic conditions. Acclimation of rats for a week preceded start of the experiment. During this period, they were fed regularly with sterilized soy diet and water ad libitum. Experiments were performed according to the guidelines of the Committee for the Purpose of Control and Supervision of Experiments on Animals (CPCSEA), India.
Chemicals
NDMA was purchased from Sigma-Aldrich Chemicals Pvt. Ltd. (St. Louis, MO). Hematoxylin and eosin stains were obtained from SRL (Bombay, Maharashtra, India). α-SMA antibodies and goat anti-mouse IgG-HRP conjugated antibodies were procured from Trends Bio-product Pvt. Ltd., New Delhi, India and CALTAG Laboratories, Bangkok, Thailand. LFT assay kits were purchased from Coral/AutoZyme Diagnostics Pvt. Ltd., New Delhi, India. Vitamin B12 was a gift from Glaxo-Smith (Remidex Pharma Pvt. Ltd., Bangalore, Karanataka, India), while all other chemicals and reagents were of analytical grade.
Induction of hepatic fibrosis and vitamin B12 treatment
Animals were divided into four groups of 15 each. First group received intraperitoneal injections of NDMA (10 µL diluted to 1 mL with 0.15 M sterile NaCl) in doses of 10 mg kg−1 body weight. The second group of animals received intraperitoneal injections of vitamin B12 in doses of 10 mg kg−1 body weight after 2 h of administering NDMA. The 2 h time interval of vitamin B12 administration was selected based on some pilot studies carried out under similar conditions. The third group served as a control and received normal saline in equal volumes, while the fourth group (also a control) was maintained under identical conditions and given the same concentration of vitamin B12 only. Injections were given on three consecutive days of each week for three weeks, as described earlier (Ahmad et al., Citation2011, Citation2012; George & Chandrakasan, Citation1996). Five animals each from all the treatment groups were anaesthetized and sacrificed on days 7, 14 and 21 from the start of the treatment. Animal body and liver weights were monitored throughout the experiment.
Sera collection and protein estimation
Blood from treated and control groups of anesthetized animals was collected through cardiac puncture in sterilized tubes on weekly basis. Sera were separated in the conventional way after incubating the blood samples at room temperature for ∼50–60 min. Samples were centrifuged at 3000 rpm under cooling for 8–10 min. The resulting clear and pale yellow color supernatant (or sera) was either freshly analyzed or stored in different aliquots at −20 °C for further analysis.
Protein concentration was determined by the method of Bradford (Citation1976) using Coomassie Blue G-250 as a color reagent and bovine serum albumin as the standard. Optical density (absorbance) was read at 595 nm on a UV-1700 Pharma-spec UV-Visible spectrophotometer.
Estimation of liver function biomarkers, hydroxyproline (HP), glycogen and vitamin B12 reserves
Liver function test (LFT) markers: alkaline phosphatase (SALP), glutamic oxaloacetic transaminase (SGOT), glutamic pyruvic transaminase (SGPT) and bilirubin were estimated in sera of control and treated groups of rats. HP levels were estimated in liver and sera of control and treated group of rats essentially by the protocol of Woessner (Citation1961). For glycogen estimation in liver samples, fresh anthrone reagent (0.2% w/v) was prepared by dissolving anthrone in 95% H2SO4. Liver extract (∼2 mL), free of any suspension, was mixed with equal volumes of 10 N KOH and kept in boiling water for 1 h. When the contents were cool, excess alkali was neutralized by adding 1 mL of glacial acetic acid and raised to 20 mL with distilled water. Approximately 2 mL of the solution was added gently to 4 mL of anthrone reagent, keeping the mixing tubes under crushed ice. After thorough mixing, the samples were shifted to boiling water bath and kept for 10–15 min for color development. The samples were read at 650 nm against blank lacking glycogen source. Since the estimation of glycogen in liver extracts using anthrone reagent includes a simultaneous hydrolysis of glycogen to glucose; hence, glycogen concentration was calculated from the glucose standard (Vies, Citation1954).
To determine vitamin B12 concentrations, calibrated quantities of respective samples were pipetted into the antibody-coated wells of immunoassay kit. Vitamin B12 present in the samples was immobilized by the rat vitamin B12 polyclonal antibody. Following washing of unbound biotinylated antibody, avidin–biotin–peroxidase complex is pipetted in to the wells. Wells were washed again and 3,3′,5,5′-tetramethylbenzidine (TMB) substrate was added to each sample well. After incubation for 25–30 min, stop solution (160 mM sulfuric acid) was added to each well that causes change in color intensities that were recorded at 450 nm.
Histopathological assessment of hepatic fibrosis and immunohistochemical localization of α-SMA
The progression of hepatic fibrosis was assessed by histochemical staining of serial sections of liver (∼5 µm) with hematoxylin and eosin (H & E). Stained slides were observed under microscope provided with an LCD attachment (Nikon; Model: 80i, Melville, NY) to examine the degree of hepatic fibrosis.
Serial liver sections were stained for α-SMA using α-SMA monoclonal antibodies, which is an established molecular marker for tracking (or identifying), activated HSCs. Briefly, endogenous peroxidase activity was quenched by incubating the liver sections with 3% H2O2 solution for 15 min. Liver sections were washed with PBS before layering with pre-diluted monoclonal α-SMA antibodies. The setup was incubated overnight in a moist chamber kept under cooling. Following washing of unbound antibodies with PBS, the slides were incubated with HRP-conjugated goat anti-mouse IgG (secondary antibody) for 40 min at room temperature. Subsequent to washing with PBS, sections were stained with 3% solution of 3,3′-diaminobenzidine tetrachloride hydrate (DAB) for 12–15 min. Finally, developed sections were rinsed with PBS, counter-stained with Mayer’s hematoxylin and mounted with DPX for further investigations.
Slides from controls and treated specimens were selected and photographed under microscope at desirable magnifications.
Statistical analysis
The values for all biochemical parameters of controls as well as the treated groups were compared and presented as mean ± SD (n = 5). To test the significant differences among the obtained values and enzyme activities, Student’s t-test was applied.
Results
Animal body and liver weight
A significant decrease in the mean body and liver weight was observed in the NDMA-treated animal group on days 14 and 21 of the experiment (p < 0.05). Rats receiving NDMA + vitamin B12 showed a considerable increase in the mean body and liver weight during the course of treatment. Relative to their respective controls, the % liver weight per gram body weight of animal showed a considerable decrease of 32.52% in NDMA-treated animals, which significantly recovered up to 95.76% by vitamin B12 administration.
Sera ALP, GOT, GPT, bilirubin, glycogen and vitamin B12 levels
Estimates of sera ALP, GOT, GPT and TBil, which are diagnostic markers of liver function, showed significant differences among different groups of rats (NDMA and NDMA + vitamin B12 and controls). Increase in the levels of ALP, GOT, GPT and TBil was observed in NDMA-treated fibrotic rats, while these parameters tend to normalize in the group receiving vitamin B12 with NDMA. There were insignificant differences in the levels of direct bilirubin (DBil) and total sera proteins (TSP). For HP, our results showed significant increase in HP levels in the sera of fibrotic rat as compared to the corresponding control group (p<0.001). Treatment of rats with vitamin B12 resulted in significant decline in sera levels of this non-essential amino acid on day 21 (). Subsequent to NDMA treatment, the decline in glycogen contents was noticed on days 7 and 14, which became more striking on day 21. In contrast, vitamin B12 supplemented group showed recovery from glycogen depletion within the first week that attained near normal levels by the end of 21 days ().
Figure 1. Liver glycogen reserves in experimental animals during NDMA-induced hepatic fibrosis and subsequent treatment with vitamin B12. Values are expressed as mean ± SD (*p < 0.05, **p < 0.001).
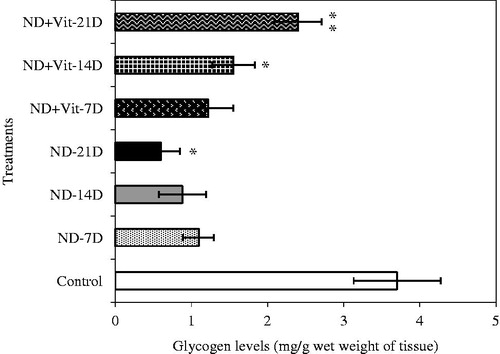
Table 1. Changes in biochemical parameters of hepatic injury in the sera and liver of control and treated rats.
In NDMA-treated group of rats, a marked decline in the levels of liver vitamin B12 corresponded with increased level of vitamin B12 in plasma in a time- and dose-dependent manner, up to 21 days of treatment (). Administering vitamin B12 subsequent to NDMA injection convincingly reflected a recovery, as liver vitamin B12 reserves returned to near normal value with a significant corresponding decline in plasma vitamin B12 concentrations (p < 0.001) ().
Histopathological and immunohistochemical assessment of hepatic damage
The H & E stained liver sections of NDMA, NDMA + vitamin B12 administered and control groups are shown in . The liver of control animals showed normal lobular architecture (). On day 7 of NDMA treatment, centrilobular congestion and disintegration of liver parenchyma were observed that were also followed by neutrophilic infiltration and severe necrosis on day 14 (). Intensive hepatic fibrosis, characterized by severe disruption of lobular architecture and abnormal collagen deposition, was distinct on day 21 of NDMA treatment (). Vitamin B12 treatment declined the levels of hepatic damage in a time- and dose-dependent manner, which is evident in rat liver sections of days 14 and 21 by the presence of moderate infiltration of inflammatory cells, mild necrosis and regenerating hepatocytes ().
Figure 3. Hematoxylin and eosin (H & E) staining of rat liver during NDMA-induced hepatic fibrosis and subsequent treatment with vitamin B12. (A) Control liver showing central vein and characteristic lobular architecture (10 × ). (B) Day 7. Peripheral lymphocyte infiltration (4×). (C) Day 14. Demonstrating Kupffer cell hyperplasia (40×). (D) Day 21. Liver congestion, lymphocyte infiltration and well developed fibrosis (10×). (E) Control liver with normal structure subsequent to vitamin B12 treatment only (4×). (F) Day 7. Liver showing lymphocyte and neutrophilic infiltration with mild fibrosis (40×). (G) Day 14. Inflammation absent with decreased fibrosis (10×). (H) Day 21. Restoration of normal liver architecture with increasing density of regenerating hepatocytes (10×).
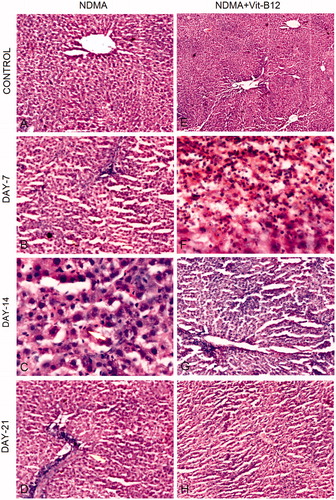
Immunohistochemical staining for α-SMA expression was performed to visualize activated hepatic stellate cells (HSCs). Expression of α-SMA was lacking in liver sections of saline (NaCl) control groups due to the absence of activated HSCs (). The number of α-SMA-positive stellate cells dramatically increased from day 7 to day 21 in liver biopsies of NDMA-treated rats (). The expression of α-SMA was also assessed in vitamin supplemented groups of animals (). Similar to saline control (), the α-SMA staining was absent in vitamin B12 control specimens which showed intact liver parenchyma and cell types (). In the vitamin B12 supplemented group, α-SMA expression continuously decreased along with the decreasing number of irregularly distributed activated HSCs from day 7 onwards (). Immunohistochemical staining of day 21 NDMA + vitamin B12 treated liver biopsies lacked α-SMA expression that was collateral with the regeneration of hepatocytes and restoration of normal liver parenchyma ().
Figure 4. Immunohistochemical staining for α-SMA during the pathogenesis of NDMA-induced hepatic fibrosis and subsequent treatment with vitamin B12. (A) Normal liver with central vein (4×). (B) Day 7. Focal α-SMA staining around central vein positively stained stellate cells (10×). (C) Day 14. Focal positivity and α-SMA stained regions showing widespread activation of hepatic stellate cells (40×). (D) Day 21. Stellate cells showing intense focal staining of α-SMA in fibrotic region (40×). (E) Normal liver architecture in vitamin B12 treated rats (4×). (F) Day 7. Focal positivity of α-SMA (4×). (G) Day 14. Scattered and decreased focal positive staining of α-SMA showing reduced number of activated hepatic stellate cells (10×). (H) Day 21. Regenerating hepatocytes with scant positivity around central vein (4×).
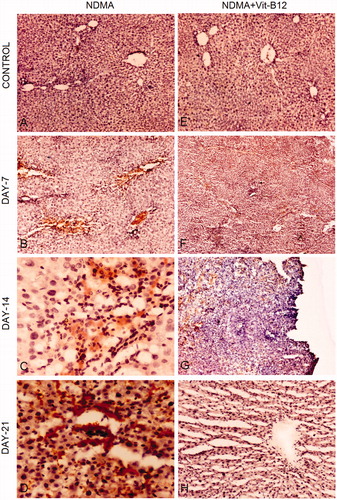
Discussion
Since NDMA-induced hepatic fibrosis in rats imitates fibrosis in human liver (Fine, Citation1982; George & Chandrakasan, 1996; Herron & Shank, Citation1980), it is one of the most extensively adopted experimental models to understand the mechanism of hepatic fibrosis. More importantly, the model has been useful in evaluating hepatoprotective potential of various natural and synthetic compounds (Ahmad et al., Citation2009; George & Chandrakasan, Citation2000; Wang et al., Citation2012; Zhen et al., Citation2007). In the present study, we have demonstrated the efficacy of vitamin B12 in alleviating NDMA-induced intensive fibrosis in rats. Our results on biochemical and histological markers are supported by immunohistochemical evidence showing increased disruption in liver architecture and enhanced expression of α-SMA in NDMA-treated rats. Major protein of cytoskeleton, α-SMA, is a sensitive marker of fibroblast formation and an established molecular marker of identifying activated hepatic stellate cells (HSCs). A continuous increase in the number of activated HSCs adds more of collagenous connective tissue to liver, resulting in the formation of distinct fibrotic areas (Das & Vasudevan, Citation2007; George et al., Citation2001; Isoda et al., Citation2008; Rockey et al., Citation1992). In our experiments, all these changes corresponded well with the duration of NDMA treatment, but with an obvious alleviating effect in the presence of vitamin B12.
NDMA treatment to rats caused a significant decline in body and liver weight from day 14 onwards and a gradual elevation in SALP, sera SGOT, sera SGPT and total bilirubin (Tbil) levels. Decreased protein synthesis, cellular necrosis and corrosion of liver parenchyma in severely fibrotic rats appear to be the most obvious causes of the weight loss and the changes observed in liver function markers (Chattopadhyay et al., Citation2012; Chowdhury & Taylor, Citation1998; Krajka-Kuzniak et al., Citation2012; Lee et al., Citation2009; Wang et al., Citation2012). Significantly increased HP (liver/sera) levels have been demonstrated in patients with various liver pathologies (Mata et al., Citation1975; Yamada & Hirayama, Citation1983). We also observed a significant increase in HP on all days of NDMA treatment. This shows that substantial degradation of newly synthesized collagen occurs in the liver due to collagenolysis, which is governed by the collagenolytic enzymes during progression of fibrosis. Increased hepatic collagenolytic activity has been reported in carbon tetrachloride induced early fibrosis (Murawaki et al., Citation1990). Vitamin B12 supplement almost normalized the above parameters by day 21. Moreover, vitamin B12 also lowered HP level, a marker of decline in collagen hydrolysis, which explains a lessened passage of this non-essential amino acid into serum. It is thus obvious that similar to other hepato-protectants, vitamin B12 restored HP levels; although it is still debatable whether it happens by way of blocking the pathways to collagenesis or by increasing the levels of collagenolytic enzymes (Ahmad et al., Citation2009; George & Chandrakasan, Citation2000).
Our serum analysis data showed that higher values of hepatic enzymes and vitamin B12 levels correspond with the increase in hepatocellular damage. The most probable causes of the observed changes are (i) failure of the damaged liver to take up cobalamin or its analogues from sera (Green, Citation1983; Kanazawa & Herbert, Citation1985) and/or; (ii) vitamin B12 binding and storage of transcobalamin (TC) in fibrotic liver is disrupted, which causes vitamin B12 to leak from liver into the circulation (Baker et al., Citation1998). The net consequence of lower binding of vitamin B12 to TC-II, which is transporter of vitamin B12 to bone marrow erythroblasts, would be impaired erythropoiesis. Our results are similar to previous reports on massive liver damage, wherein a deficiency in tissue vitamin B12 bound to TC-I and TC-III has been shown, though its concentration in blood remained high (Arendt & Nexo, Citation2012; Baker et al., Citation1998). As a result, in spite of a high total concentration of vitamin B12 (> threshold value), the concentration of TC-II bound exogenous vitamin was too low.
Concomitant with the increase in the levels of hepatic enzymes is a remarkable depletion in liver glycogen and vitamin B12 reserves by day 21 of NDMA treatment. Probably, an early but temporary depletion of vitamin B12 that manifests during NDMA treatment initiates a series of degenerative changes in liver and its cell fractions, particularly within mitochondria. Impaired mitochondrial integrity due to depleted liver vitamin B12 and glutathione levels would contribute to the increased glycolytic activity and hence cause severe depletion of hepatic glycogen reserves in fibrotic animals (Depeint et al., Citation2006; Majumdar et al., Citation2009).
It is evident from the literature that methylmalonyl Co-A mutase (MCoAM) catalyzes the isomerization of methylmalonyl CoA (MMCoA) to succinyl CoA (SCCoA) in the mitochondria. Propionyl CoA, a precursor of MMCoA, is derived from the catabolism and digestion of various amino acids, cholesterol or odd chain fatty acids. We hypothesize that vitamin B12 deficit in liver may have contributed to the declined levels of adenosylcobalamin, which signaled termination of collagen hydrolysis so as to provide derivatives for the synthesis of precursor, propionyl CoA. Adenosylcobalamin is a cofactor in conversion of MMCoA into SCCoA which participates in TCA cycle. Vitamin B12 supplement significantly decreases activated stellate cells thereby bringing down the expression of α-SMA, and thus the collagen contents by catalytic isomerization of MMCoA to SCoA and enhanced hydrolysis of deposited collagen that eventually regenerates hepatocytes within the experimental duration of 3 weeks. It can therefore be inferred that vitamin B12 may be a promising alleviating agent to be recommended for inclusion in various drug formulations to combat liver ailments; specifically, the hepatic fibrosis. Well-designed exhaustive studies are needed to confirm this hypothesis and to elucidate the modulatory or interfering role of vitamin B12 at gene level. The proposed hypothesis can, however, serve as the “unifying explication” for several published findings on chronic liver damage that causes impairment of vitamin B12-dependent isomerization and the accumulation of collagen in liver.
Declaration of interest
The authors declare no potential conflict of interest and are responsible for the writing and content of the paper.
Acknowledgements
This work is supported by grant [INSPIRE Fellowship (IF10293)/ DST], awarded to AA by DST, Ministry of Science & Technology, New Delhi. The authors sincerely thank the Chairman, Department of Zoology, for providing necessary facilities and to Prof. Veena Maheshwari, Department of Pathology, JNMC, for generously extending the microscopy facility. We are obliged to Dr. Absar-ul Hasnain, Department of Zoology, Aligarh Muslim University, for significant and necessary suggestions that helped in improving the manuscript. We also acknowledge timely help of Mr. Rashid Saleem and Mr. Mukhtar Alam for performing few biochemical estimates of our samples.
References
- Ahmad A, Fatima R, Maheshwari V, Ahmad R. (2011). Effect of N′-nitrosodimethylamine on red blood cell rheology and proteomic profiles of brain in male albino rats. Interdiscip Toxicol 4:125–31
- Ahmad A, Ahmad R. (2012). Understanding the mechanism of hepatic fibrosis and potential therapeutic approaches. Saudi J Gastroenterol 18:155–67
- Ahmad A, Maheshwari V, Ahmad A, et al. (2012). Observation of esterase-like-albumin activity during N′-nitrosodimethylamine induced hepatic fibrosis in a mammalian model. Maced J Med Sci 5:55–61
- Ahmad R, Ahmed S, Khan NU, Hasnain A. (2009). Operculina turpethum attenuates N′-nitrosodimethylamine induced toxic liver injury and clastogenicity in rats. Chem Biol Interact 181:145–53
- Arendt JFB, Nexo E. (2012). Cobalamine related parameters and disease patterns in patients with increased serum cobalamin levels. PLoS ONE 7:e45979
- Baker H, Leevy CB, DeAngelis B, et al. (1998). Cobalamin (vitamin B12) and holotranscobalamin changes in plasma and liver tissue in alcoholics with liver disease. J Am Col Nutr 17:235–8
- Birch CS, Brash NE, McCaddan A, William JHH. (2009). A novel role for vitamin B12: Cobalamins are intracellular antioxidants in vitro. Free Rad Biol Med 47:184–8
- Bradford MM. (1976). A rapid and sensitive method for the quantitation of microgram quantities of protein utilizing the principle of protein-dye binding. Anal Biochem 72:248–54
- Bruck R, Genina O, Aeed H, et al. (2001). Halofuginone to prevent and treat thioacetamide-induced liver fibrosis in rats. Hepatology 33:379–86
- Chattopadhyay S, Deb B, Maiti S. (2012). Hepatoprotective role of vitamin B12 and folic acid in arsenic intoxicated rats. Drug Chem Toxicol 35:81–8
- Chowdhury SA, Taylor R. (1998). Insulin sensitivity in experimental cirrhosis. Mol Cell Biochem 89:69–72
- Chung MY, Yeung SF, Park HJ, et al. (2010). Dietary α- and γ-tocopherol supplementation attenuates lipopolysaccharide induced oxidative stress and inflammatory-related responses in an obese mouse model of nonalcoholic steatohepatitis. J Nutr Biochem 21:1200–6
- Das SK, Vasudevan DM. (2007). Alcohol-induced oxidative stress. Life Sci 81:177–87
- Depeint F, Bruce WR, Shangari N, et al. (2006). Mitochondrial function and toxicity: Role of the B vitamin family on mitochondrial energy metabolism. Chem Biol Interact 163:94–112
- Eidi A, Moghadam JZ, Mortazavi P, et al. (2013). Hepatoprotective effects of Juglans regia extract against CCl4-induced oxidative damage in rats. Pharm Biol 51:558–65
- Ermens AAM, Vlasveld LT, Lindemans J. (2003). Significance of elevated cobalamin (vitamin B12) levels in blood. Clin Biochem 36:585–90
- Fine DH. (1982). Presence of nitrosamines in human beings. In: Magee PN, ed. Banbury Report: Nitrosamines and Human Cancer, vol. 12. New York: Cold Spring Harbor Laboratory, 281
- Friedman SL. (2010). Evolving challenges in hepatic fibrosis. Nat Rev Gastroenterol Hepatol 7:425–36
- George J, Chandrakasan G. (2000). Biochemical abnormalities during the progression of hepatic fibrosis induced by dimethylnitrosamine. Clin Biochem 33:563–70
- George J, Chandrakasan G. (1996). Molecular characteristics of dimethylnitrosamine induced fibrotic liver collagen. Biochim Biophys Acta 1292:215–22
- George J, Rao JR, Stern S, Chandrakasan G. (2001). Dimethylnitrosamine-induced liver injury in rats: The early deposition of collagen. Toxicology 156:129–38
- Green PH. (1983). Alcohol, nutrition and malabsorption. Clin Gastroenterol 12:563–74
- Harrison SA, Torgerson S, Hayashi P, et al. (2003). Vitamin E and vitamin C treatment improves fibrosis in patients with nonalcoholic steatohepatitis. Am J Gastroenterol 98:2485–90
- Herron DC, Shank RC. (1980). Methylated purines in human liver DNA after probable dimethylnitrosamine poisoning. Cancer Res 40:3116–17
- Himmerich H, Anghelescu I, Klawe C, Szegedi A. (2001). Vitamin B12 and hepatic enzyme serum levels correlate in male alcohol-dependent patients. Alcohol Alcohol 36:26–8
- Isoda K, Kagaya N, Akamatsu S, et al. (2008). Hepatoprotective effect of vitamin B12 on dimethylnitrosamine-induced liver injury. Biol Pharm Bull 31:309–11
- Kanazawa S, Herbert V. (1985). Total corrinoid, cobalamin and cobalamin analogue levels may be normal in serum despite cobalamin in liver depletion in patients with alcoholism. Lab Invest 53:108–10
- Kasprzyk-Hordern B, Andrzejewski P, Nawrocki J. (2005). The hazard of N′-nitrosodimethylamine (NDMA) formation during water disinfection with strong oxidants. Desalination 176:37–45
- Krajka-Kuzniak V, Szaefer H, Ignatowicz E, et al. (2012). Beetroot juice protects against N-nitrosodiethylamine-induced liver injury in rats. Food Chem Toxicol 50:2027–33
- Krautler B. (2005). Vitamin B12: Chemistry and biochemistry. Biochem Soc Trans 33:806–10
- Kugelmas M, Hill DB, Vivian B, et al. (2003). Cytokines and NASH: A pilot study of the effects of lifestyle modification and vitamin E. Hepatology 38:413–19
- Lee HS, Jung KH, Park IS, et al. (2009). Protective effect of morin on dimethylnitrosamine-induced hepatic fibrosis in rats. Dig Dis Sci 54:782–8
- Lotersztajin S, Julien B, Teixeira-Clerc F, et al. (2005). Hepatic fibrosis: Molecular mechanisms and drug targets. Ann Rev Pharmacol Toxicol 45:605–28
- Majumdar S, Mukherjee S, Maiti A, et al. (2009). Folic acid or combination of folic acid and vitamin B12 prevents short-term arsenic trioxide-induced systemic and mitochondrial dysfunction and DNA damage. Environ Toxicol 24:377–87
- Mata JM, Kershenobich D, Villarreal E, Rojkind M. (1975). Serum free proline and free hydroxyproline in patients with chronic liver disease. Gastroenterology 68:1265–9
- Murawaki Y, Yamada S, Koda M, Hirayama C. (1990). Collagenase and collagenolytic cathepsin in normal and fibrotic rat liver. J Biochem 108:241–4
- Rockey DC, Boyles JK, Gabbiani G, Friedman SL. (1992). Rat hepatic lipocytes express smooth muscle actin upon activation in vivo and in culture. J Submicroscopic Cytol Pathol 24:193–203
- Soda R, Tavassoli M, Jacobson DW. (1985). Receptor distribution and the endothelial uptake of transcobalamin II in liver cell suspensions. Blood 65:795–802
- van der Vies. (1954). Two methods for the determination of glycogen in liver. Biochem J 57:410–16
- Wang BS, Lee CP, Chen ZT, et al. (2012). Comparison of the hepatoprotective activity between cultured Cordyceps militaris and natural Cordyceps sinensis. J Funct Food 4:489–95
- Wells RG. (2005). The role of matrix stiffness in hepatic stellate cell activation and liver fibrosis. J Clin Gastroenterol 39:158–61
- Woessner JF Jr. (1961). The determination of hydroxyproline in tissue and protein samples containing small proportions of this imino acid. Arch Biochem Biophys 93:440–4
- Yamada S, Hirayama C. (1983). Clinical significance of serum hydroxyproline containing peptides with special reference to hyproprotein. Eur J Clin Invest 13:129–33
- Zhen MC, Wang Q, Huang XH, et al. (2007). Green tea polyphenol epigallocatechin-3-gallate inhibits oxidative damage and preventive effects on carbon tetrachloride-induced hepatic fibrosis. J Nutr Biochem 18:795–805