Abstract
Context: Injury to normal tissues is the major limiting side effect of using cyclophosphamide (CP), an antineoplastic alkylating compound.
Objective: This study was undertaken to evaluate the protective effect of an extract of Origanum vulgare L. (Lamiaceae), an antioxidative medicinal plant, against CP-induced oxidative lung damage in mice.
Materials and methods: Mice were pre-treated with various doses of O. vulgare extract (50, 100, 200, and 400 mg/kg) for 7 consecutive days followed by an injection with CP (200 mg/kg b.w.) One hour after the injection of O. vulgare on the last day, mice were injected with CP; 24 h later, they were euthanized, their lungs were immediately removed, and biochemical and histological studies were conducted.
Results: A single dose of CP markedly altered the levels of several biomarkers associated with oxidative stress in lung homogenates. Pretreatment with O. vulgare significantly reduced the levels of lipid peroxidation and attenuated the alterations in glutathione content and superoxide dismutase activity induced by CP in lung tissue. In addition, O. vulgare effectively alleviated CP-induced histopathological changes in lung tissue.
Conclusions: Our results revealed that O. vulgare protects lung tissues from CP-induced pulmonary damage and suggest a role for oxidative stress in the pathogenesis of lung disease produced by CP. Because O. vulgare has been extensively used as an additive agent and is regarded as safe, it may be used concomitantly as a supplement for reducing lung damage in patients undergoing chemotherapy.
Introduction
Reactive oxygen species (ROS) play an important role in the pathogenesis of acute and chronic lung injury. Because oxygen is indispensable for life, the cells lining the airways and the alveolar surface are continuously in contact with oxygen and do not appear to suffer. The partial reduction of oxygen to ROS occurs during cellular metabolism and during cellular injury of any type. Of the types of pulmonary defense cells, neutrophils, monocytes, and macrophages appear to be particularly predisposed to converting molecular oxygen to ROS; this process may be involved in both their phagocytic and antimicrobial activities (Doelman & Bast, Citation1990). Under physiological conditions, ROS are in balance with liver antioxidants, but a number of agents, including drugs, have been recognized to increase the production of ROS. Under these conditions, endogenous antioxidants are not able to prevent the actions of ROS and their clinical manifestations. Alkylating compounds, including anti-neoplastic drugs, form a large and important group of drugs that disturb the oxidant–antioxidant balance. One such anticancer drug is cyclophosphamide (CP), which is used to treat many types of cancer, including microcellular lung cancer (Bunn & Carney, Citation1997). CP is hydroxylated by the cytochrome P-450 mixed function oxidase (MFO) system, mainly in the liver, or co-oxidized via arachidonic acid metabolism through prostaglandin H synthetase (Patel, Citation1990; Patel & Block, Citation1985; Sladek, Citation1971). The activity of the enzymes involved in metabolic transformations of CP shows significant differences among tissues, which result in tissue-specific CP toxicity (Cooper et al., Citation1986). CP has many side effects, but injury to normal tissues is the major factor that limits its use (Bukowski, Citation1996; Fraiser et al., Citation1991). It has been reported that oxidative stress-mediated disruption of redox balance after CP exposure generates biochemical and physiological disturbances (Das et al., Citation2002; Ghosh et al., Citation2002). The lack of the detoxifying enzymes aldehyde oxidase and aldehyde dehydrogenase in the lungs causes selective CP toxicity in lung tissue. CP is well known to cause various histopathological patterns of lung injury (Kim et al., Citation2012).
Several classes of antioxidant dietary compounds have been suggested to have health benefits. Consumption of these products leads to decreases in various proinflammatory and/or oxidative stress biomarkers (Vouldoukis et al., Citation2004). Biological compounds with antioxidant properties may contribute to the protection of cells and tissues against the deleterious effects of ROS and other free radicals induced by CP (Manda & Bhatia, Citation2003; Navarova et al., Citation1999). Compounds that reduce these side effects and stimulate immunity could be of great help in improving cancer treatment. Recently, researchers have become interested in potential compounds of plant origin that are capable of minimizing the adverse effects of chemotherapy on normal cells without compromising its antineoplastic activity (Pratheeshkumar & Kuttan, Citation2010).
Origanum vulgare L. (Lamiaceae) (OV), also known as oregano, is a flavoring herb widely used around the world. O. vulgare is an aromatic plant with a wide distribution throughout Asia, particularly in Iran. It is used to cure respiratory diseases, hypoglycemic disease, and leukemia (Sheibani et al., Citation2011). The major aqueous constituents of oregano are rosmarinic acid, eriocitrin, luteolin-7-oglucoside, apigenin-7-O-glucoside, origanol A and B, and ursolic acid (Sheibani et al., Citation2011). Rosmarinic acid and origanol A and B, which are the most abundant components of the aqueous extract of oregano, have antioxidative activities (Kulisic et al., Citation2007; Matsuura et al., Citation2003). Previous studies have reported that the essential oil of O. vulgare has antioxidant capacity, which has been linked to components such as thymol, carvacrol, δ-terpinene, and p-cymene (Halici et al., Citation2005; Odabasoglu et al., Citation2004; Russo et al., Citation2002). In addition, there are many reports showing that components of the aqueous oregano extract, such as ursolic acid and rosmarinic acid, exert potent antioxidant activities by scavenging free radicals (Di Sotto et al., Citation2010; Lambert et al., Citation2001).
We recently reported that O. vulgare pretreatment attenuates radiation-induced oxidative stress and subsequent DNA damage in human blood lymphocytes. The protective effect of O. vulgare on DNA could be explained by its ability to increase the activity of the antioxidant defense system, scavenge ROS that induce lipid peroxidation as well as peroxidative damage, and quench free radicals induced by internal radiation (Arami et al., Citation2013). Since oregano contains high levels of phenolic compounds and has antioxidative properties, it is likely that O. vulgare could protect against CP-induced pulmonary injury. Because OV has been used extensively as an additive agent and is thus regarded as safe, this study was undertaken to assess the effects of OV on the oxidative lung damage induced by CP. Biochemical and histological examinations of lung tissue were performed to test for possible mitigating effects of the OV extract.
Materials and methods
Chemicals
CP (Endoxan®) was obtained from Baxter Oncology GMBH (Westfalen, Germany). Bovine serum albumin and a kit for protein measurement were purchased from Ziest Chem Co. (Tehran, Iran). All other chemicals were either at or purer than analytical grade.
Preparation of extracts
Dried powder made from the O. vulgare plant was purchased from the Giah Essence Phyto-Pharmaceutical Co., Gorgan, Iran. Aliquots of the dried powder (200 g) were extracted with 2000 ml of ethanol (75%) for 72 h. After evaporation of the solvent under reduced vacuum at a temperature below 50 °C, 25 g of dried powder was obtained.
Measurement of free radical-scavenging activity
The free radical-scavenging capacity of the ethanol extracts was determined by measuring the bleaching of stable DPPH, as described in our previous studies (Ahmadi et al., Citation2011; Azadbakht et al., Citation2011; Karimian et al., 2013). Various concentrations of the Origanum extract (0.025–0.8 mg/ml) were added, at an equal volume, to an ethanol solution of DPPH (100 μM). After 15 min, in the dark at room temperature, the absorbance was recorded at 517 nm. The experiment was performed in triplicate. Butylated hydroxytoluene (BHT) was used as a control antioxidant standard. IC50 values denote the concentration of the sample that is required to scavenge 50% of DPPH’s free radicals.
Animals
Male Naval Medical Research Institute (NMRI) mice weighing 22 ± 3 g were obtained from the Pasteur Institute of Iran (Amol, Iran). The mice were housed under standard conditions at the university animal facility and were maintained under a controlled 12 h light/dark cycle and temperature (23 ± 1 °C). The animals were acclimatized for 1 week before the study and were given standard food pellets and water ad libitum. All the animals were treated in accordance with the “Care and Use of Laboratory Animals” as prepared by the Mazandaran University of Medical Sciences. The protocol for the study was approved by the Research Committee of Mazandaran University of Medical Sciences, Sari, Iran.
Experimental treatment
For the experiment, animals were divided into six groups (Groups 1–6, n = 5 for each group), which consisted of the following: the negative control group, in which mice received distilled water (10 ml/kg b.w.) via intraperitoneal (i.p.) injection for 7 d; the positive control group, in which mice received a single toxic dose of CP (200 mg/kg b.w., i.p.) in distilled water (10 ml/kg b.w.); and groups 3–6, in which mice were treated with OV at various concentrations (50, 100, 200, and 400 mg/kg b.w. by i.p. injection) in distilled water (10 ml/kg b.w.) every day for 7 consecutive days followed by a single i.p. dose of CP 1 h after the last dose of OV. At 24 h after CP injection, all animals were weighed and sacrificed by an overdose of ether. The lungs were then isolated and washed with chilled 1.15% KCl (pH 7.4). The left lungs were used for the determination of thiobarbituric acid-reactive substance (TBARS) levels, reduced glutathione (GSH) contents, and superoxide dismutase (SOD) activities. The right lungs were fixed in 10% neutral buffered formalin for histopathological examination.
Preparation of lung homogenate
The isolated left lung/body weight ratio was determined for each animal. Homogenization was performed in ice-cold KCl (1.15%, pH 7.4) to yield 10% w/v tissue homogenates (Daba et al., Citation2002), and the biochemical parameters described below were assessed.
Determination of lipid peroxidation
The level of LP in the lung was estimated as TBARS according to Ohkawa et al. (Citation1979). The absorbance was determined spectrophotometrically at 532 nm, and the concentrations were expressed as nmol/g wet tissue.
Determination of reduced glutathione
The level of acid-soluble thiols, mainly GSH, in the lung was assayed colorimetrically, based on its reaction with Ellman’s reagent according to a previously described method (Ellman, Citation1959). The absorbance was measured at 412 nm, and the concentrations were expressed as μmol/g wet tissue.
Determination of superoxide dismutase
The enzymatic activity of SOD was assessed according to Marklund (Citation1985). SOD activity was expressed as U/g wet tissue. One unit of SOD activity is defined as the amount of enzyme that causes 50% inhibition of the auto-oxidation of pyrogallol.
Histopathological analysis
The right lungs were fixed in 10% neutral buffered formalin, sliced transversely, paraffin embedded, and prepared as 5 µm thick sections that were then stained with hematoxylin and eosin (H&E) for light microscopic evaluation. Three factors (thickening of the alveolar septa, pneumocyte hyperplasia, and infiltration by inflammatory cells) were measured using a semi-quantitative method. The level of damage was recorded based on a graded scale of 0–4, in which grade 0 = no damage, 1 = very low level of damage, 2 = mild damage, 3 = moderate damage, and 4 = severe damage. Slides were viewed and photographed using a camera attached to a microscope (Labomed, LX400, Fremont, CA) at 400× magnification in at least three random microscopic fields from each animal by two expert pathologists without the knowledge of the treatment groups.
Statistical analysis
Data are presented as the means ± standard deviation (SD). One-way analysis of variance and Tukey’s honestly significant difference (HSD) test were used for multiple comparison of data. A p value of less than 0.05 was considered to be significant. All measurements were repeated three times.
Results
Free radical-scavenging activity
The scavenging effect of the O. vulgare extract was enhanced with increasing concentration. The maximum inhibition (98.64%) was obtained at a concentration of 0.8 mg/ml. The scavenging effects of the commercial antioxidant BHT were greater than that of the extract at lower concentrations, but the O. vulgare extract had higher antioxidant activity than BHT at higher concentrations ().
Lung lipid peroxidation level
The results of the analysis of lung lipid peroxidation are shown in . A single i.p. injection of CP significantly (p < 0.001) increased the lipid peroxidation level in lung homogenates compared with the negative control group. CP increased the lung TBARS level from 56.32 ± 4.73 for non-treated mice to 174.65 ± 8.48 nmol/g wet tissue. Pretreatment with O. vulgare at various doses elicited significant decreases in the TBARS level compared to CP alone (p < 0.05–p < 0.001). The maximum effect was observed after 7 d pretreatment with the O. vulgare extract at 200 and 400 mg/kg before the CP challenge; under these conditions, the TBARS levels were significantly reduced to 65.71 ± 4.35 and 61.47 ± 3.83 nmol/g wet tissue, respectively (p < 0.001).
Table 1. Effects of CP (200 mg/kg) and/or OV on lung lipid peroxide formation expressed as TBARS, GSH content, and SOD activity.
Lung GSH levels
The GSH level observed in CP-treated animals was significantly decreased compared with the control group (p < 0.001) (). CP decreased the lung GSH level from 0.73 ± 0.12 (negative control group) to 0.18 ± 0.02 μmol/g wet tissue. Pre-treatment with O. vulgare at various doses induced significant decreases in the level of GSH compared with CP alone (p < 0.05–p < 0.001). The maximum effect was observed after 7 d administration of 200 and 400 mg/kg O. vulgare extract before the CP challenge; under these conditions, the GHS content was significantly reduced to 0.66 ± 0.26 and 0.75 ± 0.04 μmol/g wet tissue (p < 0.001).
Lung SOD activity
also shows the SOD activities in the mouse lung homogenates. The SOD activity in lung homogenates from CP-treated mice was significantly (p < 0.001) lower than that in the normal group. CP decreased the lung SOD activity from 81.25 ± 6.74 (negative control group) to 26.48 ± 3.21 U/g wet tissue. In the lung homogenates of O. vulgare-treated mice, the activity of SOD was significantly (p < 0.05–p < 0.001) higher than that in the CP group. The maximum effect was observed with pretreatment of 200 and 400 mg/kg of O. vulgare extract, which significantly increased the SOD activity to 74.61 ± 1.82 and 75.14 ± 2.48 U/g of wet tissue, respectively (p < 0.001).
Histopathological examination of the lungs
Light microscopy photomicrographs of representative histological sections of the lungs 24 h after administration of CP (200 mg/kg) alone compared with the administration of OV extract (400 mg/kg for 7 consecutive days) before CP treatment are shown in . shows a section from the lung of a control mouse showing normal alveolar spaces and alveolar septa lined with normal pneumocytes. shows a section from the lung of a mouse treated with CP showing large distal air spaces filled by lymphocytes, neutrophils and cell debris, hyperplastic pneumocytes and respiratory type epithelium. shows a section from the lung of a mouse pretreated with 400 mg/kg of OV along with CP showing normal alveolar spaces, alveolar septa with focally hyperplastic pneumocytes, and red blood cells (RBC) extravasation, as well as remnants of neutrophilic infiltration and cell debris.
Figure 2. Normal group: a section of the mouse lung tissue showing alveolar septa lined with normal pneumocytes (A, yellow arrow) and normal alveolar spaces (B, red arrow). (Hematoxylin and eosin-stained paraffin sections; H&E × 400.)
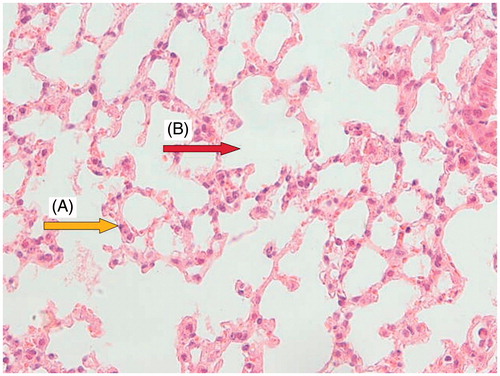
Figure 3. CP (200 mg/kg) group: a section of mouse lung showing hyperplastic pneumocytes (A, yellow arrow), respiratory type epithelium (B, green arrow), large distal air spaces filled by lymphocytes, neutrophils, and cell debris (C, red arrow). (Hematoxylin and eosin-stained paraffin sections; H&E × 400.)
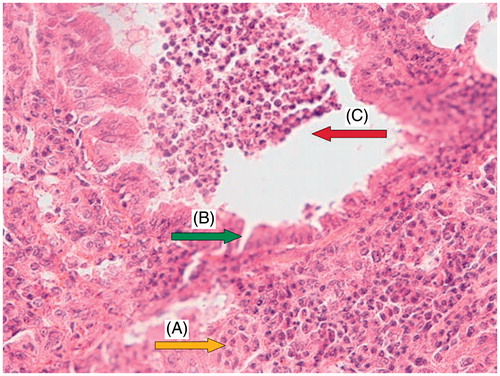
Figure 4. Group pretreated with 400 mg/kg Origanum for 7 d before CP administration: a section of mouse lung tissue showing remnants of neutrophilic infiltration and cell debris (A, green arrow), normal alveolar space (B, red arrow), and alveolar septa showing focally hyperplastic pneumocytes and RBC extravasation (C, yellow arrow). (Hematoxylin and eosin-stained paraffin sections; H&E × 400.)
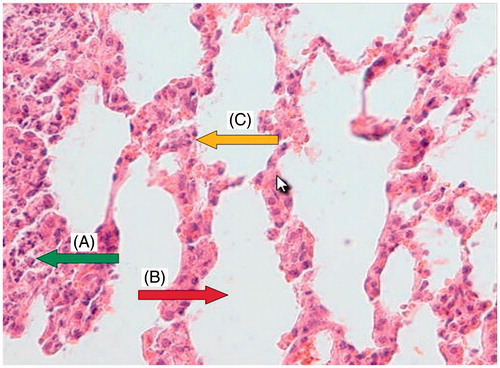
The results of the semi-quantitative histopathological examination of the lung are shown in . Briefly, the grades of damage for all three factors were 0 and 4 for untreated control animals and animals receiving a single dose of CP at 200 mg/kg, respectively, indicating severe damage to the lung tissues 24 h after CP administration. Pretreatment of mice with OV for 7 consecutive days prior to CP treatment resulted in a dose-dependent reduction in the level of lung tissue damage. These results are in agreement with the measurements of the biochemical parameters.
Table 2. The results of semi-quantitative histopathological examination of the lung, showing protection against CP-induced tissue damage by OV at various doses.
Discussion
The adverse and sometimes serious side effects of many potent chemotherapeutic agents constitute a major problem that limits their use. CP, an antineoplastic and immunosuppressive drug, is one of the most effective chemotherapeutic agents used in the treatment of cancers. Injury or damage to normal tissues is the major factor limiting the use of CP. CP treatment also results in the production of ROS, which cause peroxidative damage to vital organs (Patel, Citation1987). Cancer patients usually suffer from CP-induced lung toxicity characterized by hypoxemia, non-cardiogenic pulmonary edema, low lung compliance, and widespread capillary leakage (Malik et al., Citation1996). CP-induced lung injury is evidenced by histological findings including endothelial cell destruction, type I and type II alveolar epithelial cell damage, alveolitis, alveolar edema, hemorrhage, and extensive infiltration by inflammatory cells (Gould & Miller, Citation1975). It is also suggested that the acute effect of CP on rat lungs involves acrolein generation during drug metabolism, severe inflammatory reactions that include accumulation of neutrophils, ROS formation, and increases in lipid peroxidation (Patel, Citation1990). Lipid peroxidation is of great importance in many cellular systems, especially biological membranes, where most oxygen-activating enzymes reside. These enzymes are responsible for oxidative stress and catalyze the formation of ROS. In some cases, lipid peroxidation can overcome the endogenous cellular defense mechanisms, destroying membrane structures are destroyed and affecting organelle function (Jadhav, Citation1995). We previously reported that CP increased TBARS levels and, therefore, the extent of lipid peroxidation in the testis tissue of experimental animals (Chabra et al., Citation2014).
In the present study, the production of MDA, which is an index of lipid peroxidation, was increased significantly in lung homogenates after CP administration. This observation is in accordance with many reports that demonstrated apparent elevations in lung TBARS following the administration of CP (Patel, Citation1987; Stankiewicz et al., Citation2002; Venkatesan & Chandrakasan, Citation1995). These adverse effects of CP on the lung render it important to find a compound capable of protecting healthy lung tissue against the activity of CP metabolites such as acrolein and free radicals. Common treatments aimed at combating lipid peroxidation include the use of synthetic antioxidants with low cost and high stability (Miguel et al., Citation2003); however, such compounds often have negative side effects of their own. Therefore, researchers have focused on identifying compounds derived from plants, which may have greater efficacy and fewer side effects.
In the search for plants as a source of natural antioxidants, certain medicinal plants and fruits have been extensively studied due to their antioxidant activities. Natural products have been shown to be an excellent and reliable source for the development of new drugs (Newman & Cragg, Citation2007). Medicinal plants have potential chemopreventive properties because of their chemical constituents, such as phenolic compounds and flavonoids. The biological benefits of these compounds are generally thought to be a result of their antioxidant and free radical-scavenging properties (Ahmadi et al., Citation2008; Hosseinimehr et al., Citation2010; Tiwari, Citation2001). Chum et al. (Citation2005) found five major phenolic metabolites in oregano: rosmarinic, caffeic, coumaric and protocatechuic acids, and quercetin. These phenolic compounds are rich in hydroxyl groups, which are present at different locations on the phenolic ring. Herbs and aromatic plants, which are widespread and commonly used in the Mediterranean countries, are of commercial interest for their essential oils (Hosseinimehr et al., Citation2011; Parejo et al., Citation2002). The aromatic plant O. vulgare may also act as a potent chemoprotective agent due to its phenolic constituents rich in essential oils, such as thymol and carvacrol. The high antioxidant activity of these compounds appears to be due to the presence of phenolic OH groups, which act as hydrogen donors for the peroxy radicals produced during the first step in lipid oxidation (Roofchaee et al., Citation2011). In a recent study, SOD and GPx activities and MDA levels were measured to determine the antioxidant effects of O. vulgare. The results showed that AFB1 treatment caused decreases in SOD and GPx activities and an increase in the MDA level. The effects of AFB1 on these enzymes decreased after treatment with O. vulgare. The results of this experiment clearly revealed that OV is a potent antioxidant that could reduce oxidative stress and oxidative DNA damage (Ceker et al., Citation2012). Therefore, oregano extract may reduce the side effects and oxidative lung toxicity induced by CP due to its radical-scavenging abilities.
Therefore, we evaluated, for the first time, the chemoprotective effects of O. vulgare extract on oxidative lung toxicity induced by CP in mice. Administration of O. vulgare for 7 consecutive days before CP injection showed a significant decrease in the level of TBARS compared with the CP-treated mice. This activity could be due to the ability of O. vulgare to scavenge the free radicals generated during oxidative stress induced by CP because O. vulgare efficiently inhibited iron-dependent microsomal lipid peroxidation. The iron-reducing effects of oregano extract are thought to be due to its phenolic constituents. Studies have shown that oregano extract is an effective iron reductant and electron donor for peroxidases (Yoshino et al., Citation2006).
The disturbances in the lung antioxidant system observed in response to CP administration were characterized by changes in enzymatic and non-enzymatic antioxidant parameters. After CP injection, the lung SOD, CAT, and glutathione reductase activities were decreased, while glutathione peroxidase activity was increased (Stankiewicz et al., Citation2002; Tannehill & Mehta, Citation1996). The reductions in activity most likely involved damage to the structure of these proteins caused by ROS or aldehydes generated during CP metabolism. Cellular proteins are susceptible to oxidative modification by free radicals, which can have a significant impact on cellular function (Gebicki & Gebicki, Citation1993). Phosphoramide mustard and acrolein, the reactive metabolites of CP, may enhance the toxicity of each other in vivo. Phosphoramide mustard is a potent DNA alkylator, whereas acrolein has been demonstrated to exhibit a high reactivity toward free and protein-bound thiols and can cause depletion of glutathione (GSH). The CP metabolite acrolein and its lipid peroxidation product MDA belong to a class of highly reactive carbonyl compounds that may also modify amino acids causing changes in the structure and functions of enzymes (Haenen et al., Citation1988).
In the present study, administration of CP decreased the GSH content in the lung by approximately 75%. This result could be due to the decreased expression of this antioxidant during bronchial cellular damage (Suddek et al., Citation2012), which is in accordance with other reports demonstrating GSH reduction or depletion following CP challenge in animals (Manda & Bhatia, Citation2003; Patel & Block, Citation1985; Sulkowska et al., Citation2002; Venkatesan & Chandrakasan, Citation1995). However, the administration of O. vulgare for 7 d before CP elicited a significant increase in the level of GSH. The present work also shows that the changes in GSH are accompanied by decreases in the activity of antioxidant enzymes, namely SOD, following CP administration. A single i.p. injection of 200 mg/kg CP decreased the SOD enzyme activity in lung by approximately 67.4%. SOD constitutes an important link in the biological antioxidant defense system because of its ability to convert endogenous cytotoxic superoxide radicals, which are deleterious to polyunsaturated fatty acids and proteins, to H2O2 and molecular oxygen (Marklund, Citation1985). The reduction in the activity of SOD and increase in lipid peroxidation could reflect the adverse effects of CP on the balanced antioxidant system in the lung tissue (Selvakumar et al., Citation2005). A previous study showed that the Origanum extract has superior superoxide radical scavenger activity as compared with SOD activity (Cervato et al., Citation2000). This is in accordance with our study that demonstrated SOD increase following 7 d O. vulgare pretreatment before CP challenge in animals.
Histopathological examination of lung specimens isolated from CP-treated mice showed damage, congestion, and/or edema of the interalveolar septa, neutrophilic and macrophage infiltration, large distal air spaces filled by lymphocytes, neutrophils and cell debris, hyperplastic pneumocytes, and respiratory type epithelium. Sulkowska et al. (Citation2002) demonstrated that congestion and edema may be due to the changes produced by CP in epithelial cell structure as well as alveolocapillary permeability. Treatment with Origanum resulted in minimal lung damage, with no areas of intralobular necrosis and no significant inflammatory infiltration in the lung. In addition, normal alveolar spaces, alveolar septa showing focally hyperplastic pneumocytes and RBC extravasation as well as remnants of neutrophilic infiltration and cell debris were also observed in lung tissues after treatment with the extract. The observed histopathological results parallel the results obtained from the measured biochemical parameters.
In conclusion, Origanum had dose-dependent protective effects on lipid peroxidation induced by CP in lung tissues. Administration of Origanum extract to mice for 7 consecutive days prior to injection of CP rescued the antioxidant enzyme activity of SOD and GSH content in lung tissue. Origanum extract also showed potent radical scavenging properties, as assessed using the DPPH method. Therefore, Origanum may reduce the lung toxicity and pulmonary damage induced by CP in mice through its ability to increase the activity of the antioxidant defense system, scavenge the ROS that induce lipid peroxidation and peroxidative damage, and quench free radicals. Because O. vulgare has been used extensively as an additive agent and herbal medicine for several diseases and is generally regarded as safe, it is a potent candidate therapy for the negative side effects of chemotherapy, particularly lung toxicity.
Declaration of interest
The authors declare no conflict of interest. This study was supported by a grant from the Student Research Committee, Mazandaran University of Medical Sciences, Sari, Iran.
References
- Ahmadi A, Ebrahimzadeh MA, Ahmad-Ashrafi S, et al. (2011). Hepatoprotective, antinociceptive and antioxidant activities of cimetidine, ranitidine and famotidine as histamine H2 receptor antagonists. Fundam Clin Pharmacol 25:72–9
- Ahmadi A, Hosseinimehr SJ, Naghshvar F, et al. (2008). Chemoprotective effects of hesperidin against genotoxicity induced by cyclophosphamide in mice bone marrow cells. Arch Pharm Res 31:794–7
- Arami S, Ahmadi A, Haeri SA. (2013). The radioprotective effects of Origanum vulgare extract against genotoxicity induced by (131)I in human blood lymphocyte. Cancer Biother Radiopharm 28:201–6
- Azadbakht M, Hosseinimehr S, Shokrzadeh M, et al. (2011). Diospyros lotus L. fruit extract protects G6PD-deficient erythrocytes from hemolytic injury in vitro and in vivo: Prevention of favism disorder. Eur Rev Med Pharmacol Sci 15:1270–81
- Bukowski RM. (1996). The need for cytoprotection. Eur J Cancer 32A (Suppl 4):S2–4
- Bunn PA, Carney DN. (1997). Overview of chemotherapy for small cell lung cancer. Semin Oncol 24: S7-69-74
- Ceker S, Agar G, Nardemir G, et al. (2012). Investigation of anti-oxidative and anti-genotoxic effects of Origanum vulgare L. essential oil on human lymphocytes in vitro. J Essent Oil Bear Pl 15:997–1005
- Cervato G, Carabelli M, Gervasio S, et al. (2000). Antioxbdant properties of oregano (Origanum vulgare) leaf extracts. J Food Biochem 24:453–65
- Chabra A, Shokrzadeh M, Naghshvar F, et al. (2014). Melatonin ameliorates oxidative stress and reproductive toxicity induced by cyclophosphamide in male mice. Hum Exp Toxicol. 33:185–95
- Chum S, Vattem D, Lin Y, Shetty K. (2005). Phenolic antioxidants from clonal oregano (Origanum vulgare) with antimicrobial activity against Helicobacter pylori. Process Biochem 40:809–16
- Cooper JA, White DA, Matthay RA. (1986). Drug-induced pulmonary disease. Part 1: Cytotoxic drugs. Am Rev Respir Dis 133:321–40
- Daba MH, Abdel-Aziz AA, Moustafa AM, et al. (2002). Effects of l-carnitine and Ginkgo biloba extract (EG b 761) in experimental bleomycin-induced lung fibrosis. Pharmacol Res 45:461–7
- Das UB, Mallick M, Debnath JM, Ghosh D. (2002). Protective effect of ascorbic acid on cyclophosphamide-induced testicular gametogenic and androgenic disorders in male rats. Asian J Androl 4:201–7
- Di Sotto A, Mazzanti G, Carbone F, et al. (2010). Inhibition by beta-caryophyllene of ethyl methanesulfonate-induced clastogenicity in cultured human lymphocytes. Mutat Res 699:23–8
- Doelman CJ, Bast A. (1990). Oxygen radicals in lung pathology. Free Radic Biol Med 9:381–400
- Ellman GL. (1959). Tissue sulfhydryl groups. Arch Biochem Biophys 82:70–7
- Fraiser LH, Kanekal S, Kehrer JP. (1991). Cyclophosphamide toxicity. Characterising and avoiding the problem. Drugs 42:781–95
- Gebicki S, Gebicki JM. (1993). Formation of peroxides in amino acids and proteins exposed to oxygen free radicals. Biochem J 289:743–9
- Ghosh D, Das UB, Ghosh S, et al. (2002). Testicular gametogenic and steroidogenic activities in cyclophosphamide treated rat: A correlative study with testicular oxidative stress. Drug Chem Toxicol 25:281–92
- Gould VE, Miller J. (1975). Sclerosing alveolitis induced by cyclophosphamide. Ultrastructural observations on alveolar injury and repair. Am J Pathol 81:513–30
- Haenen GR, Vermeulen NP, Tai Tin Tsoi JN, et al. (1988). Activation of the microsomal glutathione-S-transferase and reduction of the glutathione dependent protection against lipid peroxidation by acrolein. Biochem Pharmacol 37:1933–8
- Halici M, Odabasoglu F, Suleyman H, et al. (2005). Effects of water extract of Usnea longissima on antioxidant enzyme activity and mucosal damage caused by indomethacin in rats. Phytomedicine 12:656–62
- Hosseinimehr SJ, Ahmadashrafi S, Naghshvar F, et al. (2010). Chemoprotective effects of Zataria multiflora against genotoxicity induced by cyclophosphamide in mice bone marrow cells. Integr Cancer Ther 9:219–23
- Hosseinimehr SJ, Mahmoudzadeh A, Ahmadi A, et al. (2011). The radioprotective effect of Zataria multiflora against genotoxicity induced by gamma irradiation in human blood lymphocytes. Cancer Biother Radiopharm 26:325–9
- Karimian A, Shokrzadeh M, Ahmadi A. (2013). The potential chemoprotective effects of melatonin against genotoxicity induced by diazinon in human peripheral blood lymphocytes. Toxicol Ind Health. [Epub ahead of print]. doi:10.1177/0748233713500824
- Jadhav SJ, Nimbalkar SS, Kulkarni AD, Madhavi DL. (1995). Lipid oxidation in biological and food systems. In: Madhavi DL, Deshpande SS, Salunkhe DK, ed. Food Antioxidants: Technological, Toxicological and Health Perspectives. Vol. 1. New York: Marcel Dekker Inc., 159–265
- Kim S, Tannock I, Sridhar S, et al. (2012). Chemotherapy-induced infiltrative pneumonitis cases in breast cancer patients. J Oncol Pharm Pract 18:311–15
- Kulisic T, Krisko A, Dragovic-Uzelac V, et al. (2007). The effects of essential oils and aqueous tea infusions of oregano (Origanum vulgare L. spp. hirtum), thyme (Thymus vulgaris L.) and wild thyme (Thymus serpyllum L.) on the copper-induced oxidation of human low-density lipoproteins. Int J Food Sci Nutr 58:87–93
- Lambert RJ, Skandamis PN, Coote PJ, Nychas GJ. (2001). A study of the minimum inhibitory concentration and mode of action of oregano essential oil, thymol and carvacrol. J Appl Microbiol 91:453–62
- Malik SW, Myers JL, DeRemee RA, Specks U. (1996). Lung toxicity associated with cyclophosphamide use. Two distinct patterns. Am J Respir Crit Care Med 154:1851–6
- Manda K, Bhatia AL. (2003). Prophylactic action of melatonin against cyclophosphamide-induced oxidative stress in mice. Cell Biol Toxicol 19:367–72
- Marklund SL. (1985). Superoxide dismutase isoenzymes in tissues and plasma from New Zealand black mice, nude mice and normal BALB/c mice. Mutat Res 148:129–34
- Matsuura H, Chiji H, Asakawa C, et al. (2003). DPPH radical scavengers from dried leaves of oregano (Origanum vulgare). Biosci Biotechnol Biochem 67:2311–16
- Miguel MG, Figueiredo AC, Costa MM, et al. (2003). Effect of the volatile constituents isolated from Thymus albicans, Th. mastichina, Th. carnosus and Thymbra capitata in sunflower oil. Nahrung 47:397–402
- Navarova J, Ujhazy E, Dubovicky M. (1999). Protective effect of the antioxidant stobadine against cyclophosphamide and irradiation induced oxidative stress. Gen Physiol Biophys 18:112–19
- Newman DJ, Cragg GM. (2007). Natural products as sources of new drugs over the last 25 years. J Nat Prod 70:461–77
- Odabasoglu F, Aslan A, Cakir A, et al. (2004). Comparison of antioxidant activity and phenolic content of three lichen species. Phytother Res 18:938–41
- Ohkawa H, Ohishi N, Yagi K. (1979). Assay for lipid peroxides in animal tissues by thiobarbituric acid reaction. Anal Biochem 95:351–8
- Parejo I, Viladomat F, Bastida J, et al. (2002). Comparison between the radical scavenging activity and antioxidant activity of six distilled and nondistilled Mediterranean herbs and aromatic plants. J Agric Food Chem 50:6882–90
- Patel JM. (1987). Stimulation of cyclophosphamide-induced pulmonary microsomal lipid peroxidation by oxygen. Toxicology 45:79–91
- Patel JM. (1990). Metabolism and pulmonary toxicity of cyclophosphamide. Pharmacol Ther 47:137–46
- Patel JM, Block ER. (1985). Cyclophosphamide-induced depression of the antioxidant defense mechanisms of the lung. Exp Lung Res 8:153–65
- Pratheeshkumar P, Kuttan G. (2010). Ameliorative action of Vernonia cinerea L. on cyclophosphamide-induced immunosuppression and oxidative stress in mice. Inflammopharmacology 18:197–207
- Roofchaee A, Irani M, Ebrahimzadeh MA, Akbari MR. (2011). Effect of dietary oregano (Origanum vulgare) essential oil on growth performance, cecal microflora and serum antioxidant activity of broiler chickens. Afr J Biotechnol 10:6177–83
- Russo A, Bonina F, Acquaviva R, et al. (2002). Red Orange extract: Effect on DNA cleavage. J Food Sci 67:2814–18
- Selvakumar E, Prahalathan C, Mythili Y, Varalakshmi P. (2005). Mitigation of oxidative stress in cyclophosphamide-challenged hepatic tissue by dl-α-lipoic acid. Mol Cell Biochem 272:179–85
- Sheibani V, Afarinesh M, Hajializadeh Z. (2011). Evaluation of Origanum vulgare L. ssp. Viridis leaves extract effect on discrimination learning and LTP induction in the CA1 region of the rat hippocampus. Iran J Basic Med Sci 14:177–84
- Sladek NE. (1971). Metabolism of cyclophosphamide by rat hepatic microsomes. Cancer Res 31:901–8
- Stankiewicz A, Skrzydlewska E, Makiela M. (2002). Effects of amifostine on liver oxidative stress caused by cyclophosphamide administration to rats. Drug Metabol Drug Interact 19:67–82
- Suddek GM, Ashry NA, Gameil NM. (2012). Thymoquinone attenuates cyclophosphamide-induced pulmonary injury in rats. Inflammopharmacology 21:427–35
- Sulkowska M, Skrzydlewska E, Sobaniec-Lotowska M, et al. (2002). Effect of cyclophosphamide-induced generation reactive oxygen forms on ultrastructure of the liver and lung. Bull Vet Inst IN Pulway 46:239–46
- Tannehill SP, Mehta MP. (1996). Amifostine and radiation therapy: Past, present, and future. Semin Oncol 23:69–77
- Tiwari AK. (2001). Imbalance in antioxidant defence and human diseases: Multiple approach of natural antioxidants therapy. Curr Sci Banglore 81:1179–87
- Venkatesan N, Chandrakasan G. (1995). Modulation of cyclophosphamide-induced early lung injury by curcumin, an anti-inflammatory antioxidant. Mol Cell Biochem 142:79–87
- Vouldoukis I, Lacan D, Kamate C, et al. (2004). Antioxidant and anti-inflammatory properties of a Cucumis melo LC. extract rich in superoxide dismutase activity. J Ethnopharmacol 94:67–75
- Yoshino K, Higashi N, Koga K. (2006). Antioxidant and antiinflammatory activities of oregano extract. J Health Sci 52:169–73