Abstract
Context: Podophyllotoxin, a pharmaceutically important bioactive compound of Podophyllum sps. (Berberidaceae), is in great demand worldwide as an anticancer and antivirus drug precursor. However, the source of podophyllotoxin is very limited due to the endangered status of the Podophyllum plant.
Objective: The aim of this study was to isolate podophyllotoxin-producing endophytic fungi from Sinopodophyllum hexandrum (Royle) Ying (1979) (Berberidaceae) plants of the Taibai Mountains of China in order to obtain bioactive compounds.
Materials and methods: The strains producing kaempferol and podophyllotoxin were screened by thin-layer chromatography (TLC) analysis. The presence of kaempferol and podophyllotoxin in extracts of these strains was further confirmed by high-performance liquid chromatography (HPLC) and nuclear magnetic resonance (NMR) analyses.
Results: Among six endophytic fungi isolated from the rhizomes of S. hexandrum, one strain was able to produce kaempferol. Another strain, named TW5, was able to produce both kaempferol and podophyllotoxin simultaneously according to the TLC, HPLC, and NMR results. The podophyllotoxin yield of TW5 was calculated to be 49.3 μg/g of mycelial dry weight after 7-d fermentation. Strain TW5 was identified morphologically and phylogenetically to be Mucor fragilis Fresen. (Mucoraceae). These results suggest that the podophyllotoxin-synthesizing ability is obtained by uptaking genes involved in the podophyllotoxin synthesis from the host plant into endophytic fungal genomes.
Conclusion: Our results showed, for the first time, that the endophytic fungus M. fragilis is able to produce simultaneously the same two bioactive metabolites, podophyllotoxin and kaempferol, as its host plant. Furthermore, the relatively high podophyllotoxin yield obtained may improve the industrial production of podophyllotoxin, which may help protect this endangered plant.
Introduction
Podophyllotoxin is a valuable natural product with wide medical and agricultural applications because of its strong antineoplastic and antiviral activities (Canel et al., Citation2000; Guerram et al., Citation2012). It is used as a precursor for the synthesis of many well-known antineoplastic drugs such as etoposide, etopophos, and teniposide (Canel et al., Citation2000). Due to its cellular cytoskeleton disruption and reverse transcriptase inhibition abilities, podophyllotoxin can interfere with viral replication and thus be used to treat viral diseases such as condyloma acuminatum (Wilson, Citation2002). Kaempferol is a flavonoid found in many edible and herbal plants (Calderon-Montano et al., Citation2011). It has a plethora of pharmacological activities including anticancer, antioxidant, and anti-inflammatory activities (Calderon-Montano et al., Citation2011). Thus, the worldwide demand for podophyllotoxin and kaempferol is high and rapidly increasing (Guerram et al., Citation2012).
Podophyllum emodi Wall. (syn. P. hexandrum) and Podophyllum peltatum L. (Berberidaceae) are the major sources of podophyllotoxin (Guerram et al., Citation2012). These plants grow very slowly and require strict growth conditions (Moraes et al., Citation2000). Due to intensive collection, these plants are becoming critically endangered, limiting the supply of podophyllotoxin (Guerram et al., Citation2012). Although much effort has been made, the cultivation of these plants has been unsuccessful and chemical or biosynthesis of podophyllotoxin is commercially unacceptable. Therefore, it is necessary to find alternative sources to satisfy the pharmaceutical demand of podophyllotoxin (Guerram et al., Citation2012).
Endophytic fungi are ubiquitous in vascular plants, and many are able to produce the same bioactive metabolites as their host plant (Chandra, Citation2012; Stierle et al., Citation1995; Strobel et al., Citation2003). Several studies have reported the isolation of podophyllotoxin-producing endophytic fungi from Podophyllum and Juniperus recurva (Cupressaceae) (Eyberger et al., Citation2006; Kour et al., Citation2008; Mohammad et al., Citation2012; Puri et al., Citation2006). However, their podophyllotoxin yields are still commercially unacceptable. Thus, the isolation of novel podophyllotoxin- and kaempferol-producing endophytic fungi would have significant biological and commercial implications by providing sustainable sources of these bioactive compounds and reducing the harvest of the endangered plant. Since Podophyllum has a long history of use in traditional Chinese herbal medicine (Broomhead & Dewick, Citation1990; Giri & Lakshmi Narasu, Citation2000; Jackson & Dewick, Citation1984, Citation1985), the aim of this study was to isolate a new podophyllotoxin- and kaempferol-producing endophytic fungus from a wild collection of Sinopodophyllum hexandrum (Royle) Ying (1979) (syn. P. emodi Wall.) plants in the family Berberidaceae at a high altitude in the Taibai Mountains of China.
Materials and methods
Isolation of endophytic fungi
The intact plant was collected from an altitude of 2600 m in the Taibai Mountains of Shaanxi, China in August 2009. Samples were placed immediately in sterile plastic bags, stored at 4 °C, and used for isolation of endophytic fungi within 48 h after collection. The plant was identified morphologically and phylogenetically to be S. hexandrum by phytology Professor Ming Yue from Northwest University. The plant was deposited at the herbarium in Northwest University, Xi’an, China, under the accession number NWU.2009.08. The plant was cleaned with sterilized water, and its rhizomes were surface-sterilized and cut into 0.5–1.0 cm pieces (Xu et al., Citation2009). Next, the pieces were placed on potato dextrose agar (PDA) plates containing 50 mg/L streptomycin to inhibit bacterial growth. The PDA plates were incubated at 26 ± 2 °C. After endophytic fungal growth, the strains were isolated by routine microbiological methods. The purified strains were numbered and stored at 4 °C.
Strain selection by thin-layer chromatography (TLC)
The endophytic fungi were grown in 500 mL flasks containing 200 mL of fermentation medium (potato, 200 g; glucose, 20 g; and distilled water, 1 L, pH 6.5) and incubated at 26 ± 2 °C for 7 d on a reciprocal shaker at 180 rpm. Fermentation medium without inoculation was used as a negative control. The mycelia collected were frozen at −20 °C for 4 h, crushed by a high-speed tissue triturator, and refluxed in 500 mL of 80% ethanol for 2 h. The ethanol extract was concentrated by a RE252A rotary evaporator (Yarong, Co., Ltd., Shanghai, China) at 60 °C to 10 mL and extracted using an equal volume of chloroform. Chloroform extracts of the strains were spotted on GF254 silica gel plates (Qingdao Haiyang Chemical Co., Ltd., Shandong, China) and run in a chloroform:methanol (9:1, v/v) solvent system. Standard podophyllotoxin (Sigma, St Louis, MO) and kaempferol (NPEC Co., Ltd., Zhejiang, China) were run along with the samples. The plate was observed under ultraviolet (UV) light and visualized by spraying with ethanol:sulfuric acid (1:1, v/v) followed by heating at 110 °C for 5 min. The relative front (Rf) values of each sample were measured and compared with those of standard podophyllotoxin and kaempferol.
Identification of endophytic fungus
The morphological characteristics of endophytic fungal strain TW5 were identified according to Wei (Citation1979). For molecular identification, the total genomic DNA of strain TW5 was extracted by the cetyl trimethylammonium bromide method (Cappiccino, Citation1996) and used for amplification of the 18 S, 5.8 S, and 28 S ribosomal regions using primers ITS1 (5′-TCCGTAGGTGAACCTGCGG-3′) and ITS4 (5′-TCCTCCGCTTATTGATATGC-3′) (White, Citation1990). The amplified products were sequenced at Sangon, Shanghai (China). The DNA sequence of the 578 base product obtained was searched in the ribosomal gene database (http://blast.ncbi.nlm.nih.gov/Blast.cgi?PROGRAM=blastn&PAGE_TYPE=BlastSearch&LINK_LOC=blasthome) by the program BLASTN (Altschul et al., Citation1997). The rRNA gene sequences of strain TW5 and its closest relatives were aligned using the automated tools of the ARB software package (Ludwig et al., Citation2004). The aligned sequences were added to the ARB neighbor-joining tree.
The rRNA gene sequence of strain TW5 was submitted to the Gene Bank under the accession number KC007440. The endophytic fungal strain TW5 was deposited at the China Center for Type Culture Collection, Wuhan, China, under the accession number M 205032.
Determination of growth curves
The endophytic fungal strain TW5 was inoculated into 500-mL flasks containing 200 mL of fermentation medium at a ratio of 1:9 (inoculum:medium). The flasks were incubated at 26 ± 2 °C on a reciprocal shaker at 180 rpm. The mycelia of three flasks were harvested every 24 h. The wet mycelia were dried in an oven at 85 °C. The average dry weight of mycelia was used to draw the growth curve. TLC was used to monitor the podophyllotoxin and kaempferol produced during growth.
Fermentation, extraction, and purification
Strain TW5 was inoculated into 300 flasks (500 mL) containing 200 mL of fermentation medium and incubated at 26 ± 2 °C on a reciprocal shaker at 180 rpm. After 7-d incubation, 60 L of culture liquid containing 4250 g of mycelia (wet weight) was obtained. A sample of 200 g of mycelia was dried and weighed to calculate the water content, and the dry weight of 60 L of culture liquid was calculated accordingly. The chloroform extract from the mycelia of the 60 L culture liquid was prepared as described in the “Strain selection by TLC” section. The chloroform extract was concentrated to 10–15 mL and redissolved in an equal volume of chloroform. The redissolved extract was purified by a glass chromatography column (32 cm × 4 cm) packed with silica gel (200–300 mesh, Qingdao Haiyang Chemical Co., Ltd., Haiyang, China), which was eluted with chloroform:methanol at different ratios (9.5:0.5, 9:1, and 8:2, v/v) and pure methanol, successively. The eluents were analyzed by TLC, and podophyllotoxin- or kaempferol-positive fractions were found in the 9.5:0.5 and 9:1 chloroform:methanol fractions, respectively. The podophyllotoxin-positive fraction was concentrated and further purified by silica gel column chromatography (25 cm × 2 cm; chloroform:methanol, 9.5:0.5, v/v). The podophyllotoxin-positive fraction of the second purification was concentrated and recrystallized from ether to afford 12.5 mg of needle-like crystals. This compound was assigned as compound I. The kaempferol-positive fraction was concentrated, dissolved in 95% ethanol, and filtered; and these steps were repeated several times for further purification. The purified kaempferol-positive fractions were concentrated to provide 11.8 mg of straw-yellow powder, which was assigned as compound II.
High-performance liquid chromatography (HPLC) analysis
Standard podophyllotoxin and compound I were analyzed by an Alliance HPLC system (Waters, Milford, MA) equipped with a UV detector. Analyses were performed on a 5 -μm Sunfire-C18 column (250 mm × 4.6 mm, Waters Alliance, Milford, MA) at 30 °C with a mobile phase of methyl cyanide:water (45:55, v/v), a flow rate of 1.0 mL/min, and a detection wavelength of 290 nm. The concentrated kaempferol-positive fractions and standard kaempferol were analyzed by a Hitachi HPLC system equipped with a UV detector. Analyses were performed on a 10 μm YWC-155 C18 column (250 mm × 4.6 mm, Hitachi, Tokyo, Japan) at 25 °C with a mobile phase of methanol:0.4% phosphoric acid (60:40, v/v), a flow rate of 1.0 mL/min, and a detection wavelength of 360 nm.
Nuclear magnetic resonance (NMR) spectroscopy
The structures of compounds I and II were identified on an INOVA-400 spectrometer (Varian, Palo Alto, CA) (1H-NMR: 400 MHz, 13C-NMR: 100 MHz) at 25 °C. Each sample (10 mg) was dissolved in 0.5 mL of deuterochloroform (CDCl3). Chemical shifts were reported in δ (ppm) relative to tetramethylsilane as the internal standard using CDCl3 as the solvent. For 1H-NMR determination, the pulse sequence was s2pul; the number of scans was 16; the spectrometer frequency was 399.76; and the spectral width was 10 000.0. For 13C-NMR determination, the pulse sequence was s2pul; the number of scans was 1736; the spectrometer frequency was 100.53; and the spectral width was 29 996.2. The coupling constants are given in Hz.
Results
Isolation of endophytic fungi and strain selection by TLC
Six morphologically unique endophytic fungal strains (named as TW1–6) were isolated from the rhizomes of S. hexandrum and cultured for the production of podophyllotoxin and kaempferol. TLC screening of all six strains showed that two of the strains (TW3 and TW5) produced kaempferol (). The mycelial extracts of these strains displayed spots of the same Rf value (0.25) and color (yellow under UV light) as that of standard kaempferol on TLC plates. In addition, strain TW5 also produced podophyllotoxin. By TLC, the mycelial extract of strain TW5 had the same Rf value (0.69) and visualization characteristics (bright purple under UV light and purplish red after ethanol:sulfuric acid spray) as that of standard podophyllotoxin. Therefore, strain TW5 was selected for further studies. The extract of the negative control did not show any evidence of background podophyllotoxin or kaempferol in the medium by TLC analysis. Furthermore, extracts from the culture filtrate collected after mycelium filtration did not show the presence of these compounds (data not shown), indicating that podophyllotoxin and kaempferol were present mainly inside the mycelium.
Table 1. Endophytic fungal strains and their TLC screening results.
Identification of the endophytic fungus of strain TW5
Strain TW5 was identified based on its morphological characteristics and fungal ribotyping. The TW5 colony grew fast with white mycelium spreading on a PDA plate within 5 d (). Microscopic examination showed that the mycelia were fistulous and aseptate. Sporangiophores grew from the mycelium and formed monopodial or sympodial branches with a length of 7509–2160 μm. The rhizoid and stolon were absent. The sporangia were round, hazel, and easily ruptured when they were mature (). The sporangiospores were ellipsoid or limoniform (4.8–7.2 μm × 2.4–3.6 μm) (). Alignment of the rRNA gene sequence obtained from strain TW5 showed 98% homology with the corresponding gene sequence of Mucor fragilis. The phylogenetic relationship of strain TW5 and the podophyllotoxin-producing endophytic fungi isolated in previous reports is illustrated in . Thus, based on its morphological characteristics and ribosomal sequence, strain TW5 was identified to be endophytic fungus M. fragilis Fresen. (Mucoraceae).
Growth curves
The growth of M. fragilis was monitored for up to 12 d, and the greatest biomass was observed on days 6 and 7 (), after which a slight decrease in mycelial dry weight was observed and the stationary phase was reached. The TLC results showed that podophyllotoxin and kaempferol were produced on day 6 onwards (data not shown), which is consistent with previous findings showing that secondary metabolites are often produced around the stationary phase of growth (Griffin, Citation1994).
HPLC analysis of fermentation products
HPLC analyses of the fungal extracts denoted as compounds I and II showed peaks with retention times of 7.2 min and 12.7 min, respectively, which corresponded to standard podophyllotoxin and kaempferol (–). These results are consistent with the TLC strain selection results and further suggest that M. fragilis extract contains podophyllotoxin and kaempferol.
Podophyllotoxin yield determination
The podophyllotoxin yield of M. fragilis was calculated based on the HPLC results. As determined by HPLC, podophyllotoxin accounted for 98.5% of compound I ( and ). In addition, after a 7-d fermentation, 12.5 mg of compound I was produced by 250 g of mycelia (dry weights). Thus, the podophyllotoxin yield of M. fragilis was calculated to be 49.3 μg/g of mycelial dry weight, which was greater than that of previous reports (Kour et al., Citation2008; Mohammad et al., Citation2012).
NMR analysis of fermentation products
The chemical formulas of compounds I and II were identified as C22H22O8 and C15H10O6, respectively, by 1H- and 13C-NMR (– and and ). The 1H-NMR and 13C-NMR spectra of compounds I and II (–) were in good agreement with those of authentic podophyllotoxin (Agrawal & Thakur, Citation1985; Fonseca et al., Citation1980; Jackson & Dewick, Citation1984) and kaempferol (Farzin & Naaman, Citation2003; Wawer & Zielinska, Citation2001), respectively. Based on these results, compounds I and II from M. fragilis were identified as podophyllotoxin and kaempferol, respectively.
Figure 5. Chemical structures of podophyllotoxin (a) and kaempferol (b). Numbers represent the carbon positions.
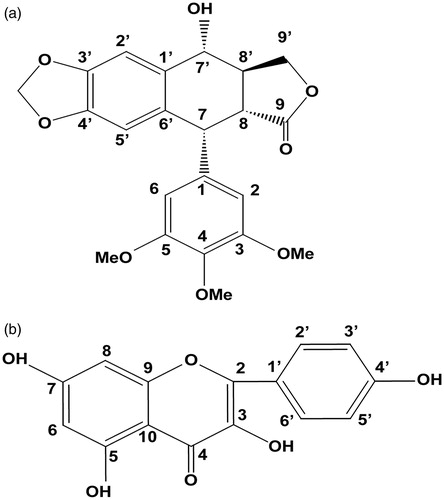
Table 2. 1H- and 13C-NMR characterization of fungal podophyllotoxin.
Table 3. 1H- and 13C-NMR characterization of fungal kaempferol.
Discussion
In this study, the endophytic fungus M. fragilis (strain TW5) was shown to produce podophyllotoxin and kaempferol simultaneously. This report is the first to describe the isolation of an endophytic fungus that produces the same two bioactive metabolites as does its host plant, S. hexandrum. Our results are consistent with those of previous studies that have found that podophyllotoxin-producing endophytic fungi can be isolated from podophyllotoxin-producing plants (Podophyllum species and Juniperus recurva) (Eyberger et al., Citation2006; Kour et al., Citation2008; Mohammad et al., Citation2012; Puri, Citation2006). These studies support the theory of horizontal gene transfer between the host plant and its endophytes due to long periods of co-evolution and adaption (Germaine et al., Citation2004). The results also indicated that podophyllotoxin and kaempferol could be successfully synthesized by endophytic fungus fermentation, providing a new approach for pharmaceutical production.
Various podophyllotoxin-producing endophytic fungi including Trametes hirsute, Fusarium solani, Fusarium oxysporum, and Phialocephala fortinii have been isolated from different podophyllotoxin-producing plants (Eyberger et al., Citation2006; Kour et al., Citation2008; Mohammad et al., Citation2012; Puri et al., Citation2006). In this paper, we report the isolation of M. fragilis from the rhizomes of wild S. hexandrum, which was collected at a high altitude in the Taibai Mountains of China. It was shown to be phylogenetically distinct from previously isolated podophyllotoxin-producing endophytes (). This result indicates that the diversity of podophyllotoxin-producing endophytic fungi is very abundant at the genus level. The rich diversity of podophyllotoxin-producing endophytic fungi and their ability to produce the same multiple bioactive compounds as their host plant suggest that the podophyllotoxin-synthesizing ability is obtained by uptaking genes involved in podophyllotoxin synthesis from the host plant into endophytic fungal genomes (Germaine et al., Citation2004). Thus, endophytic fungi may have the identical or a similar pathway for podophyllotoxin synthesis as their host plants. Since the entire biosynthesis pathway of podophyllotoxin, including key enzyme(s) and the genetic blueprint, has not yet been elucidated (Guerram et al., Citation2012), the isolation of podophyllotoxin-producing endophytic fungi may provide a clue to better understand this pathway through genome sequencing by a high-throughput sequencing technique.
This report is the first to describe that relatively high purity and high yield of needle-like crystals of podophyllotoxin could be obtained via endophytic fungus fermentation. Meanwhile, M. fragilis has the same ability to produce podophyllotoxin and kaempferol as the original strain after more than ten generations of serial cultivation, indicating that this strain has good genetic stability. These results suggest that M. fragilis could be used as a candidate for podophyllotoxin production. It could also be used as a good starting strain for genetic engineering experiments to further improve the production of these bioactive compounds. Thus, the endangered Podophyllum species would be protected due to the reduced harvest of wild populations of the source plant from its natural habitat.
Declaration of interest
All authors declared that there were no conflicts of interest involved in this study.
Acknowledgements
We thank Professor Zhao Changqi and Professor Sun Wenji of Northwest University for their kind help regarding plant collection, identification, and fermentation product analysis. This work was funded by the National Natural Science Foundation of China (Grant no. 41072254) and the Natural Science Basic Research Plan in Shaanxi Province, China (Program no. 2003C107).
References
- Altschul SF, Madden TL, Schaffer AA, et al. (1997). Gapped BLAST and PSI-BLAST: A new generation of protein database search programs. Nucl Acids Res 25:3389–402
- Agrawal PK, Thakur RS. (1985). 13C NMR spectroscopy of lignan and neolignan derivatives. Magn Reson Chem 23:389–418
- Broomhead AJ, Dewick PM. (1990). Tumour-inhibitory aryltetralin lignans in Podophyllum versipelle, Diphylleia cymosa and Diphylleia grayi. Phytochemistry 29:3831–7
- Calderon-Montano JM, Burgos-Moron E, Perez-Guerrero C, Lopez-Lazaro M. (2011). A review on the dietary flavonoid kaempferol. Mini Rev Med Chem 11:298–344
- Canel C, Moraes RM, Dayan FE, Ferreira D. (2000). Podophyllotoxin. Phytochemistry 54:115–20
- Cappiccino JG. (1996). Microbiology: A Laboratory Manual. Redwood City (CA): The Benjamin/Cummings Publishing Company
- Chandra S. (2012). Endophytic fungi: Novel sources of anticancer lead molecules. Appl Microbiol Biotechnol 95:47–59
- Eyberger AL, Dondapati R, Porter JR. (2006). Endophyte fungal isolates from Podophyllum peltatum produce podophyllotoxin. J Nat Prod 69:1121–4
- Farzin H, Naaman K. (2003). Kaempferol from saffron petals. Iran J Pharm Res 2:251–2
- Fonseca SF, Rúveda EA, McChesney JD. (1980). 13C NMR analysis of podophyllotoxin and some of its derivatives. Phytochemistry 19:1527–30
- Germaine K, Keogh E, Garcia-Cabellos G, et al. (2004). Colonisation of poplar trees by gfp expressing bacterial endophytes. FEMS Microbiol Ecol 48:109–18
- Giri A, Lakshmi Narasu M. (2000). Production of podophyllotoxin from Podophyllum hexandrum: A potential natural product for clinically useful anticancer drugs. Cytotechnology 34:17–26
- Griffin DH. (1994). Fungal Physiology. New York, USA: John Wiley and Sons
- Guerram M, Jiang ZZ, Zhang LY. (2012). Podophyllotoxin, a medicinal agent of plant origin: Past, present and future. Chin J Nat Med 10:161–9
- Jackson DE, Dewick PM. (1984). Aryltetralin lignans from Podophyllum hexandrum and Podophyllum peltatum. Phytochemistry 23:1147–52
- Jackson DE, Dewick PM. (1985). Tumour-inhibitory aryltetralin lignans from Podophyllum pleianthum. Phytochemistry 24:2407–9
- Kour A, Shawl A, Rehman S, et al. (2008). Isolation and identification of an endophytic strain of Fusarium oxysporum producing podophyllotoxin from Juniperus recurva. World J Microbiol Biotechnol 24:1115–21
- Ludwig W, Strunk O, Westram R, et al. (2004). ARB: A software environment for sequence data. Nucl Acids Res 32:1363–71
- Mohammad N, Mauji R, Pravej A, Grothaus P. (2012). Fusarium solani, P1, a new endophytic podophyllotoxin-producing fungus from roots of Podophyllum hexandrum. Afr J Microbiol Res 6:2493–9
- Moraes R, Burandt C, Ganzera M, et al. (2000). The American mayapple revisited – Podophyllum peltatum – still a potential cash crop? Econ Bot 54:471–6.
- Puri SC, Nazir A, Chawla R, et al. (2006). The endophytic fungus Trametes hirsuta as a novel alternative source of podophyllotoxin and related aryl tetralin lignans. J Biotechnol 122:494–510
- Stierle A, Strobel G, Stierle D, et al. (1995). The search for a taxol-producing microorganism among the endophytic fungi of the pacific yew, Taxus brevifolia. J Nat Prod 58:1315–24
- Strobel GA. (2003). Endophytes as sources of bioactive products. Microbes Infect 5:535–44
- Wawer I, Zielinska A. (2001). 13C CP/MAS NMR studies of flavonoids. Magn Reson Chem 39:374–80
- Wei J. (1979). The Handbook of Fungi Identification. Shanghai, China: Shanghai Scientific and Technical Publishers
- White TJ. (1990). Fungal Physiology. New York, USA: Academic Press
- Wilson J. (2002). Treatment of genital warts — what's the evidence? Int J STD AIDS 13:216–22.
- Xu LL, Han T, Wu JZ, et al. (2009). Comparative research of chemical constituents, antifungal and antitumor properties of ether extracts of Panax ginseng and its endophytic fungus. Phytomedicine 16:609–16