Abstract
Context: Venous irritation is the most common side effect of intravenous therapy. Although many in vitro models have been developed to evaluate intravenous drug irritation, these models are not widely accepted.
Objectives: The aim of this paper is to determine whether delayed or immediate cytotoxicity better reflects the in vivo venous irritation ranking.
Materials and methods: We compared the endothelial toxicity induced by high-concentrations of amiodarone and diazepam after short-term exposure (20 min) in a human umbilical vein endothelial cell line (EVC304) by using five in vitro models: lactate dehydrogenase (LDH), glucose-6-phosphate dehydrogenase (G6PD), glutathione (GSH), adenosine triphosphate (ATP), and MTT assays.
Results: In the 24-h MTT assay, the IC50 of diazepam and amiodarone was 1.08 and 1.96 mM, respectively. In the 48-h MTT assay, the IC50 of diazepam and amiodarone was 1.114 and 1.128 mM, respectively. In the intracellular LDH and G6PD assays, the EC50 of diazepam was found to be 3.307 and 1.53 mM, while the values of amiodarone were 0.853 and 0.325 mM, respectively. In the intracellular ATP and GSH assays, the EC50 of diazepam was 0.905 and 1.283 mM, while the values of amiodarone were 0.040 and 0.326 mM, respectively.
Conclusion: Both the results of intracellular macromolecule activities and micromolecule concentrations were similar to that observed in in vivo venous irritation studies. However, the delayed cytotoxicity rank from the MTT assay is inconsistent with the in vivo venous irritation rank, suggesting that initial toxicity, but not the delayed toxicity, is related to venous irritation.
Introduction
Although venous irritation is the most common side effect of intravenous therapy, its pathogenesis is not fully understood. The most prevalent opinion is that chemical irritation of the endothelium leads to sterile inflammation. Vascular injury not only causes multi-organ dysfunction but also compromises the normal pharmacokinetics of therapeutic drugs. In areas with increased vascular permeability, drugs may extravasate and accumulate inside the interstitial space. The bioavailability and effectiveness of the drug may decrease, and the local toxicity may increase (Hu et al., Citation2008). Therefore, intravenous drug-induced vascular irritation and injury is an issue of concern because there are no apparent diagnostic markers for either pre-clinical or clinical monitoring (Brott et al., Citation2005).
Various methods have been developed to evaluate the venous irritation caused by intravenous drugs. The classic venous irritation test is an in vivo model that determines the pathology of rabbit ear vein tissue (Hoover et al., Citation1990; Kohno et al., Citation2008). Other in vivo models, such as the rabbit ear temperature model (Ward et al., Citation1991a,Citationb; White & Yalkowsky, Citation1991) and the rat lateral tail vein model (Wang et al., Citation1999), have also been used to assess drug-induced phlebitis. In vitro models, such as the hemolysis test (Husa & Adams, Citation1944) and the intracellular phosphokinase enzyme activity in an L6 muscle cell line (Hoover et al., Citation1990), have been described. However, these in vitro models have not been widely accepted due to their failure to accurately indicate drug-induced venous irritation. In 1990, Hoover and colleagues (Hoover et al., Citation1990) compared in vitro and in vivo models to assess the venous irritation of parenteral drugs and determined that the in vivo model provides a sensitive measure of the potential venous tolerance to parenteral formulations and that the conventional hemolysis test results were inconsistent if the drug formulations contained certain components that may alter the ultraviolet absorption spectrum of hemoglobin (Reed & Yalkowsky, Citation1985, Citation1986; Yalkowsky, Citation1998). The use of non-vessel cells for an in vitro assessment of local tolerance is problematic because the results reflect the general cytotoxicity potential rather than a target-specific response.
In vivo studies provide the most direct and accurate measurements; however, in vitro models, which can be performed rapidly and do not involve animal ethics, have proven to be extremely useful in preformulation studies because these allow early changes to the formulation and thus avoid expensive delays (Hoover et al., Citation1990; Yalkowsky et al., Citation1998). The endothelial lining of blood vessels functions as a barrier between the blood and the interstitial compartments to control and restrict the trans-endothelial flux of fluid and macromolecules (Mehta & Malik, Citation2006). These cells, which are the first cells that are exposed to the drug at the site of injection, have been commonly used to predict the injection site toxicity. Some researchers have focused on the endothelial cell compatibility of intravenous drugs (Robibaro et al., Citation1998; Vorbach et al., Citation2002) and have recommended important clinical medication parameters based on the in vitro toxicity dosage (Robibaro et al., Citation1998). Intracellular depletion assays of adenosine triphosphate (ATP), adenosine diphosphate, guanosine diphosphate, guanosine triphosphate, and lactate dehydrogenase (LDH) (Armbruster et al., Citation2000; Medlicott et al., Citation1998; Vorbach et al., Citation1998, Citation2002) and endothelial cell viability assays (Kruse et al., Citation2007) have also been used. Nevertheless, these studies used varied methodologies and different cell types, exposure times, and quantifiable endpoints. Different experimental designs and assays reflect different pathophysiological implications. Thus, it is uncertain which of the above-mentioned assays are suitable for the prediction of venous irritation.
Amiodarone, which is an antiarrhythmic drug, is commonly administered as a bolus injection followed by a short-term infusion (Ward et al., Citation1991a). Diazepam, which is a benzodiazepine sedative, is incorporated into a stable, submicronized injectable emulsion (Levy et al., Citation1989). Previous non-clinical (White & Yalkowsky, Citation1991) and clinical studies (Hilleman et al., Citation1987; Levy et al., Citation1989) showed that the venous irritation of diazepam is slight, while that of amiodarone is severe. Thus, in this study, we deliberately chose these two drugs, which cause phlebitis in a very different degree, to assess the immediate and delayed cytotoxicity induced by short-term exposure to a high dose of drugs in an established human umbilical vein endothelial cell line (EVC304). The purpose of this paper is to determine whether delayed or immediate cytotoxicity better reflects the in vivo venous irritation ranking.
Materials and methods
Materials
Diazepam and amiodarone injections were purchased from Tianjin Jinyao Amino Acid Co., Ltd. (Tianjin, China) and Sanofi-Aventis Co. (Paris, France), respectively. 3-(4,5-Dimethylthiazol-2-yl)-2,5-diphenyltetrazolium bromide (MTT), glucose-6-phosphate (G6P), diaphorase, phenazine methosulfate (PMS), iodonitrotetrazolium chloride (INT), Triton X-100, and dimethyl sulfoxide (DMSO) were obtained from Sigma-Aldrich (St. Louis, MO). NADP+, NAD+, and bovine serum albumin (BSA) were obtained from Roche Ltd. (Basel, Switzerland). Dulbecco’s modified Eagle’s medium (DMEM), ethylenediaminetetraacetic acid (EDTA), fetal calf serum (FCS), and trypsin were purchased from Gibco BRL (Grand Island, NY). The 96-well and 6-well plates were purchased from Corning Incorporated (One Riverfront Plaza, NY). All the other reagents were of analytical grade.
Cell cultures and treatments
EVC304 is a unique, spontaneously transformed human umbilical vein endothelial cell line that displays a specific repertoire of surface molecules (Mutin et al., Citation1997). This cell line was kindly provided by Prof. Qiujun Lv of the Academy of Military Medical Sciences (Beijing, China). The cells were grown in DMEM supplemented with 10% heat-inactivated FCS and 1% penicillin–streptomycin solution in a humidified incubator with 5% CO2 at 37 °C.
For the MTT assay, the cells were cultured in 96-well plates (3–5 × 104 cells/well) overnight. For the intracellular LDH, ATP, glucose-6-phosphate dehydrogenase (G6PD), and glutathione (GSH) assays, cells were cultured in six-well plates (0.5–1 × 106 cells/well) and allowed to attach for 24 h before treatment. The cells were then washed with Hank’s buffer and treated with diazepam or amiodarone for 20 min at 37 °C.
MTT assay
The cells were treated for 20 min with diazepam or amiodarone, and the possibility of delayed toxicity was evaluated 24 h and 48 h after the removal of the drugs. After treatment, the cells were washed with Hank’s buffer, and the culture medium was replaced with new DMEM without drugs. The cells were cultured for 21 h and 45 h and then incubated with 20 μl MTT (0.5 mg/ml) for 3 h. The medium was aspirated, and 200 μl of DMSO was added to each well to dissolve the formazan crystal. The absorbance at 540 nm was then measured.
Intracellular LDH assay
The intracellular LDH activity was measured as previously described with some modifications (Decker & Lohmann-Matthes, Citation1988). After treatment, the cells were incubated with 0.2% Triton X-100 at 37 °C for 30 min with shaking (800 rpm). The cell lysates were collected and centrifuged at 4000 × g for 15 min at 4 °C, and the supernatant was recovered. The supernatant (70 μl) was transferred to microtiter wells, and 20 μl of the lithium lactate solution (36 mg/ml in 10 mM Tris buffer, pH 8.5) and then 20 μl of INT solution (2 mg/ml in PBS, pH 7.2) was added to each of the wells. The enzymatic reaction was initiated by the addition of 20 μl of a solution containing NAD+ and diaphorase (3 mg/ml NAD+, 13.5 U/ml diaphorase, 0.03% BSA, and 1.2% sucrose in PBS, pH 7.2), and the mixture was incubated at room temperature for 20 min. The absorbance at 492 nm was then measured.
Intracellular G6PD assay
The intracellular G6PD activity was determined using a previously described method (Quillen et al., Citation2006) with some modifications. Dulbecco’s phosphate-buffered saline (D-PBS) was prepared according to Freshney (Citation1983). After incubation, the cells were treated with the primary assay mixture [0.125 mM NADP and 0.05% (w/v) Triton X-100]. The plates were incubated for 30 min at 37 °C with shaking (800 rpm). The supernatant was transferred to a microcentrifuge tube and centrifuged at 4000 × g for 10 min. The supernatant was dispensed at 150 μl/well in a 96-well plate. About 50 μl of the secondary assay mixture (0.333 M Tris, pH 7.8; 0.2% Triton X-100; 0.02 mM PMS; 1.25 mM INT; 0.125 mM NADP and 4.2 mM G6P) was added to each well. The plate contents were mixed for 30 s on a plate shaker and incubated at 37 °C for 10 min. The absorbance was measured at 492 nm.
Intracellular ATP assay
The intracellular ATP content was determined using the ATP chemiluminescence assay kit (Vigorous, Beijing, China). After incubation, the cells were lysed with 0.5 M HClO4, and the reaction was neutralized with 1 M K2CO3. The cell lysates were collected and centrifuged at 10 000 × g for 15 min at 4 °C. Then, 10 μl of the supernatant of each sample was used for the assay according to the kit specifications.
Intracellular GSH assay
The intracellular GSH concentration was measured according to a previously reported method (Hissin & Hilf, Citation1976) with some modifications. After treatment, the cells were incubated for 2 min with 0.5 M HClO4, and the reaction was neutralized with 1 M K2CO3. The cell lysates were collected and centrifuged at 10 000 × g for 15 min at 4 °C, and the supernatant was recovered. The supernatant (10 μl) was transferred to a 96-well solid black flat-bottom plate, and 180 μl of PBS (pH 7.2) and 10 μl of ο-phthalaldehyde (1 mg/ml in methanol) were added to each well. The fluorescence was measured using a fluorescence microplate reader (Thermo Scientific, Vantaa, Finland) with an excitation wavelength of 355 nm and an emission wavelength of 405 nm.
Statistics and data analysis
A one-way ANOVA was used to determine the statistical significance between different groups. A Student t-test was used when only two groups were compared. The IC50 (50% inhibition concentration) and EC50 (50% depletion concentration) values were calculated from the concentration–response curves through a graphic method to determine the significant right-ward shift. Data represent the mean ± SD of at least three independent experiments, each experiment was performed in triplicate. Differences with p < 0.05 were considered statistically significant.
Results
Effects on cell viability
Cell cytotoxicity assays are the most common in vitro bioassay methods that are used to predict the toxicity of substances in various tissues. These assays demonstrate the degree of damage caused by a drug. Compared with the control group, diazepam and amiodarone markedly decreased the number of viable cells in a concentration-dependent manner (). In the 24 h assay, the IC50 values of diazepam and amiodarone were 1.08 ± 0.025 mM and 1.96 ± 0.030 mM, respectively. In the 48-h MTT assay, the IC50 values of diazepam and amiodarone are 1.114 ± 0.016 mM and 1.128 ± 0.003 mM, respectively.
Figure 1. Delayed toxicity of diazepam and amiodarone on the viability of EVC304 cells. The cells were exposed to a high concentration of the drugs for a period of 20 min. The drugs were removed, and the delayed toxicity was evaluated at 24 h (A) and 48 h (B). *p < 0.05 and **p < 0.01 compared with the normal control group.
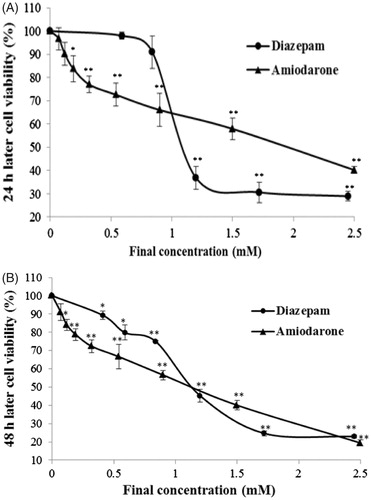
Effects on LDH
Most cells contain LDH, and the loss of membrane integrity due to lethal injury can be assessed by monitoring the concentration of intracellular or extracellular LDH (Koizumi et al., Citation1996). Compared with the normal control group, diazepam and amiodarone markedly decreased the intracellular LDH concentration in a concentration-dependent manner (). The concentration–response curve of diazepam was significantly right-shifted compared with that obtained for amiodarone (p < 0.01). Diazepam did not lead to a significant decrease in the LDH levels at a dosage of 1.01 mM, whereas amiodarone decreased the LDH concentration significantly at a dosage of 0.32 mM. The EC50 values of diazepam and amiodarone were found to be 3.307 ± 0.040 mM and 0.853 ± 0.031 mM, respectively.
Effects on G6PD
The G6PD enzyme, which is abundant in the endothelial cell cytoplasm (Quillen et al., Citation2006), produces NADPH and plays a key role in the regulation of cellular redox (Lee et al., Citation2011). G6PD was recently shown to regulate growth in nucleated cells (Gao et al., Citation2009). A previous study showed that the G6PD status affected the cellular responses to external stimuli (Cheng et al., Citation2000). G6PD can be stabilized in an aqueous solution by the addition of NADP+ and one of its substrates and then assayed using the secondary assay mixture. In this assay, 4.2 mM G6P, as the substrate, was added to the mixture, and the NADP+ concentration was adjusted to stabilize the enzyme. Compared with the normal control group, diazepam and amiodarone markedly decreased the intracellular G6PD concentration in a concentration-dependent manner. As shown in , the concentration–response curve of diazepam was significantly right-shifted compared with that obtained for amiodarone (p < 0.01). G6PD was not depleted with the addition of 0.69 mM diazepam, whereas 0.32 mM amiodarone significantly decreased the G6PD concentration. The EC50 values of diazepam and amiodarone were found to be 1.53 ± 0.003 mM and 0.325 ± 0.023 mM, respectively.
Effects on ATP
ATP is a major metabolite of living cells and is rapidly lost in injured cells (Imamura et al., Citation2009). The endothelial metabolism was examined by determining the intracellular ATP concentration of the treated cells. Exposure to diazepam or amiodarone for 20 min induced a concentration-dependent decrease in the cellular ATP content of the treated cells (). The concentration–response curve of diazepam was significantly right-shifted compared with that obtained for amiodarone (p < 0.01). Exposure to 0.48 mM diazepam did not significantly affect the intracellular ATP concentration, whereas 26 μM amiodarone leads to a significant decrease in the intracellular ATP level. The EC50 values of diazepam and amiodarone were found to be 0.905 ± 0.015 mM and 40.30 ± 1.527 μM, respectively.
Effects on GSH
GSH, a cysteine-containing tripeptide of glutamate, cysteine, and glycine, is the most abundant endogenous non-protein thiol in cells (Fang et al., Citation2002; Parcell, Citation2002). This tripeptide plays important roles in the detoxification of xenobiotic compounds and as an antioxidant of ROS and free radicals (Masella et al., Citation2005). A shortage of intracellular GSH usually causes a cell to be at risk for oxidative damage (Nagai et al., Citation2002). Therefore, the maintenance of intracellular GSH equilibrium is essential for cellular function and survival. Based on the concentration–response curves (), both diazepam (at a concentration ranging from 0.84 to 3.50 mM) and amiodarone (at a concentration ranging from 0.12 to 1.50 mM) decrease the intracellular GSH concentration. The intracellular GSH concentration–curve of diazepam was significantly right-shifted compared with that obtained for amiodarone (p < 0.01). The EC50 values of diazepam and amiodarone were found to be 1.283 ± 0.021 mM and 0.326 ± 0.015 mM, respectively.
Discussion
In this study, EVC304 cells were exposed to diazepam or amiodarone injection at high concentrations for 20 min (Armbruster et al., Citation2000; Robibaro et al., Citation1998; Vorbach et al., Citation1999), and the initial injury (LDH, G6PD, ATP, and GSH assays) and delayed toxicity (MTT assays) were examined. High concentrations of the drugs were used because many injectable drugs may be used clinically at higher concentrations than their aqueous solubility limits (Yalkowsky et al., Citation1998). To minimize interference from the test compounds, we measured intracellular components instead of extracellular factors. In a previous study (Robibaro et al., Citation1998), the levels of extracellular prostacyclin I2 and thromboxane A2, which are secreted by endothelial cells were measured. However, functional secretion assays are usually applicable to normal cells with an integral membrane, whereas short-term exposure to high concentrations of a drug may impair the cell membrane. Thus, it was reasonable to measure intracellular factors instead of extracellular secretions.
After short-term exposure to a high concentration of the tested drugs, the intracellular levels of both macromolecule (LDH and G6PD) and micromolecule (GSH and ATP) components were variably decreased, and this decreases may be attributed to the leakage of intracellular factors through an impaired plasmalemma. The level of cytomembrane damage, which was indirectly measured by the reduction in the levels of intracellular factors, was similar to the level of venous irritation () (Ward et al., Citation1991a; White & Yalkowsky, Citation1991). Our results also illustrate how the drug dosage may vary between different in vitro assays. For instance, the EC50 values of amiodarone determined through intracellular assays measuring ATP and LDH were 0.040 and 0.853 mM, respectively. There is a 21.3-fold difference in the drug EC50 values found between these two assays. Thus, it is not accurate to recommend a clinical dosage based on a single in vitro assay. In fact, the evaluation of the cytomembrane integrity through a macromolecule or micromolecule assay may lead to an erroneous conclusion. The reduction in the intracellular enzyme activity may result from cytoplasmic leakage or enzyme denaturation (Paolucci-Jeanjean et al., Citation2001). Therefore, based on the effects of amiodarone on G6PD, LDH, and GSH, the significant depletion of intracellular ATP may be due to other mechanisms in addition to cytoplasmic leakage. The simultaneous determination of micromolecule concentrations and enzyme activities to evaluate the venous irritation caused by different drugs may be more accurate than to the determination obtained using only one assay. The simultaneous reduction in the levels of both micromolecules and macromolecules after short-term drug exposure is likely attributable to cytoplasmic leakage through an impaired plasmalemma.
Figure 6. Comparison of the EC50 values of diazepam and amiodarone determined through the ATP, G6PD, GSH, and LDH assays. **p < 0.01 compared with the diazepam group.
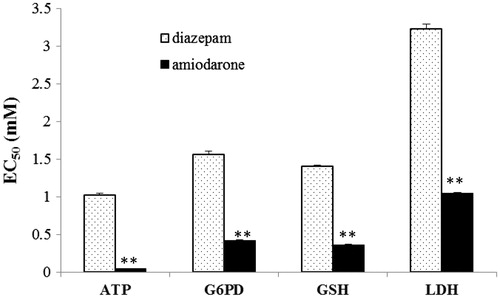
MTT assay quantifies mitochondrial activity by measuring the formation of a dark blue formazan product formed by the reduction of the tetrazolium ring of MTT. The reduction of MTT is thought to mainly occur in the mitochondria through the action of succinate dehydrogenase, therefore, providing a measure of cell viability. Although the method was previously used for evaluating the venous irritation of macrolides on endothelial cells (Millrose et al., Citation2009), our results indicate that the MTT assay is not an effective method. After short-term exposure, endothelial cellular content was significantly reduced but the change of mitochondrial activity was undetected, indicating the plasma membrane breakdown appears to precede the respiratory impairment. The inconsistency between the delayed cytotoxicity and the extent of damage of plasmalemma suggests that the intracellular mitochondrial function may have been impaired by the drug accumulated in the cells. Therefore, it is important to choose suitable assay methods depending on the cell damage mode. Our study can help to find the appropriate ones for evaluating the degree of venous irritation.
Conclusions
In conclusion, this study demonstrates that venous irritation is related to initial toxicity rather than delayed toxicity after short-term exposure of EVC304 to amiodarone or diazepam. Because the initial toxicity of endothelial cells may represent cell damage by venous irritation, we used the endothelial cell line EVC304 in our study. The depletion of endothelium-associated molecules is also indicative of acute cellular damage. Further studies focusing on the direct relationships between in vitro models and in vivo assays will be necessary for the establishment of a vascular endothelial cell model for the evaluation of venous irritation.
Declaration of interest
The authors declare that there are no conflicts of interest. This work was supported by the National Natural Science Foundation of China (Nos. 81274163 and 81173645) and National S&T Major Project and Scientific Researchers Aiding Enterprise Item (Nos. 2012ZX09301-002-001 and 2009ZX09301-003) from the Ministry of Science and Technology of the People’s Republic of China.
References
- Armbruster C, Robibaro B, Griesmacher A, Vorbach H. (2000). Endothelial cell compatibility of trovafloxacin and levofloxacin for intravenous use. J Antimicrob Chemother 45:533–5
- Brott D, Gould S, Jones H, et al. (2005). Biomarker of drug-induced vascular injury. Toxicol Appl Pharmacol 207:441–5
- Cheng ML, Ho HY, Liang CM, et al. (2000). Cellular glucose-6-phosphate dehydrogenase (G6PD) status modulates the effects of nitric oxide (NO) on human foreskin fibroblasts. FEBS Lett 475:257–62
- Decker T, Lohmann-Matthes ML. (1988). A quick and simple method for the quantitation of lactate dehydrogenase release in measurements of cellular cytotoxicity and tumor necrosis factor (TNF) activity. J Immunol Methods 115:61–9
- Fang YZ, Yang S, Wu G. (2002). Free radicals, antioxidants and nutrition. Nutrition 18:872–9
- Freshney RI. (1983). Culture of Animal Cells. New York, USA: Alan R. Liss, Inc
- Gao LP, Cheng ML, Chou HJ, et al. (2009). Ineffective GSH regeneration enhances G6PD-knockdown hep G2 cell sensitivity to diamide-induced oxidative damage. Free Radic Biol Med 47:529–35
- Hilleman DE, Hansen JM, Mohiuddin SM. (1987). Amiodarone-induced infusion phlebitis. Clin Pharm 6:364–67
- Hissin PJ, Hilf R. (1976). A fluorimetric method for determination of oxidized and reduced glutathione in tissues. Anal Biochem 74:214–26
- Hoover DM, Gardner JB, Timmerman TL, et al. (1990). Comparison of in vitro and in vivo models to assess venous irritation of parenteral antibiotics. Fundam Appl Toxicol 4:589–97
- Hu G, Place AT, Minshall RD. (2008). Regulation of endothelial permeability by Src kinase signaling: Vascular leakage versus transcellular transport of drugs and macromolecules. Chem Biol Interact 171:177–89
- Husa WJ, Adams JR. (1944). Isotonic solutions II. The permeability of red corpuscles to various substances. J Am Pharm Assoc Sci Ed 33:329–32
- Imamura H, Nhat KP, Togawa H, et al. (2009). Visualization of ATP levels inside single living cells with fluorescence resonance energy transfer-based genetically encoded indicators. Proc Natl Acad Sci USA 106:15651–6
- Kohno E, Murase S, Nishikata M, et al. (2008). Methods of preventing vinorelbine-induced phlebitis: An experimental study in rabbits. Int J Med Sci 5:218–23
- Koizumi T, Shirakura H, Kumagai H, et al. (1996). Mechanism of cadmium-induced cytotoxicity in rat hepatocytes: Cadmium-induced active oxygen-related permeability changes of the plasma membrane. Toxicology 114:125–34
- Kruse M, Kilic B, Flick B, Stahlmann R. (2007). Effect of quinupristin/dalfopristin on 3T3 and Eahy926 cells in vitro in comparison to other antimicrobial agents with the potential to induce infusion phlebitis. Arch Toxicol 81:447–52
- Lee JW, Choi AH, Ham M, et al. (2011). G6PD up-regulation promotes pancreatic β-cell dysfunction. Endocrinology 152:793–803
- Levy MY, Langerman L, Gottschalk-Sabag S, Benita S. (1989). Side-effect evaluation of a new diazepam formulation: Venous sequel reduction following intravenous (i.v.) injection of a diazepam emulsion in rabbits. Pharm Res 6:510–16
- Masella R, Di Benedetto R, Varì R, et al. (2005). Novel mechanisms of natural antioxidant compounds in biological systems: Involvement of glutathione and glutathione-related enzymes. J Nutr Biochem 16:577–86
- Medlicott NJ, Foster KA, Audus KL, et al. (1998). Comparison of the effects of potential parenteral vehicles for poorly water soluble anticancer drugs (organic cosolvents and cyclodextrin solutions) on cultured endothelial cells (HUV-EC). J Pharm Sci 87:1138–43
- Mehta D, Malik AB. (2006). Signaling mechanisms regulating endothelial permeability. Physiol Rev 86:279–367
- Millrose M, Kruse M, Flick B, Stahlmann R. (2009). Effects of macrolides on proinflammatory epitopes on endothelial cells in vitro. Arch Toxicol 83:469–76
- Mutin M, Dignat-George F, Sampol J. (1997). Immunologic phenotype of cultured endothelial cells: Quantitative analysis of cell surface molecules. Tissue Antigens 50:449–58
- Nagai H, Matsumaru K, Feng G, Kaplowitz N. (2002). Reduced glutathione depletion causes necrosis and sensitization to tumor necrosis factor-alpha-induced apoptosis in cultured mouse hepatocytes. Hepatology 36:55–64
- Paolucci-Jeanjean D, Belleville MP, Rios GM. (2001). A comprehensive study of the loss of enzyme activity in a continuous membrane reactor-application to starch hydrolysis. J Chem Technol Biotechnol 76:273–8
- Parcell S. (2002). Sulfur in human nutrition and applications in medicine. Altern Med Rev 7:22–44
- Quillen EE, Haslam GC, Samra HS, et al. (2006). Ectoadenylate kinase and plasma membrane ATP synthase activities of human vascular endothelial cells. J Biol Chem 281:20728–37
- Reed KW, Yalkowsky SH. (1985). Lysis of human red blood cells in the presence of various cosolvents. J Parenter Sci Technol 39:64–9
- Reed KW, Yalkowsky SH. (1986). Lysis of human red blood cells in the presence of various cosolvents. II. The effect of differing NaCl concentrations. J Parenter Sci Technol 40:88–94
- Robibaro B, Vorbach H, Weigel G, et al. (1998). Endothelial cell compatibility of glycopeptide antibiotics for intravenous use. J Antimicrob Chemother 41:297–300
- Wang Y, Mesfin GM, Rodríguez CA, et al. (1999). Venous irritation, pharmacokinetics, and tissue distribution of tirilazad in rats following intravenous administration of a novel supersaturated submicron lipid emulsion. Pharm Res 16:930–8
- Ward GH, Nolan PE Jr, White M, Yalkowsky SH. (1991a). Studies in phlebitis. II. Early detection of Amiodarone-induced phlebitis in a rabbit model. Pharm Res 8:801–3
- Ward GH, Nolan PE Jr, Chawla M, Yalkowsky SH. (1991b). Studies in phlebitis: Detection and quantitation using a thermographic camera. Pharm Res 8:76–9
- White M, Yalkowsky SH. (1991). Studies in phlebitis. III. Evaluation of diazapam and phenytoin. Pharm Res 8:1341–2
- Yalkowsky SH. (1998). Solubility and Solubilization of Drugs. Washington, USA: American Chemical Society
- Yalkowsky SH, Krzyzaniak JF, Ward GH. (1998). Formulation-related problems associated with intravenous drug delivery. J Pharm Sci 87:787–96
- Vorbach H, Weigel G, Robibaro B, et al. (1998). Endothelial cell compatibility of clarithromycin for intravenous use. Clin Biochem 31:653–6
- Vorbach H, Robibaro B, Armbruster C, et al. (1999). Endothelial cell compatibility of clindamycin, gentamicin, ceftriaxone and teicoplanin in Bier's arterial arrest. J Antimicrob Chemother 44:275–7
- Vorbach H, Armbruster C, Robibaro B, et al. (2002). Endothelial cell compatibility of azithromycin and erythromycin. J Antimicrob Chemother 49:407–9