Abstract
Context: The application of bleomycin is limited due to its side effects including lung toxicity. Silymarin is a flavonoid complex isolated from milk thistle [Silybum marianum L. (Asteraceae)] which has been identified as an antioxidant and anti-inflammatory compound.
Objective: This study evaluates the effect of silymarin on oxidative and inflammatory parameters in the lungs of mice exposed to bleomycin.
Materials and methods: BALB/c mice were divided into four groups of control, bleomycin (1.5 U/kg), bleomycin plus silymarin (50 and 100 mg/kg). After bleomycin administration, mice received 10 d intraperitoneal silymarin treatment. On 10th day, blood and lung samples were collected for measurement of oxidative and inflammatory factors.
Results: Silymarin led to a decrease in lung lipid peroxidation (0.19 and 0.17 nmol/mg protein) in bleomycin-injected animals. Glutathione-S-transferase (GST) which was inhibited by bleomycin (32.4 nmol/min/mg protein) induced by higher dose of silymarin (41 nmol/min/mg protein). Silymarin caused an elevation in glutathione (GSH): 2.6 and 3.1 µmol/g lung compare with bleomycin-injected animals 1.8 µmol/g lung. Catalase (CAT) was increased due to high dose of silymarin (65.7 µmol/min/ml protein) compare with bleomycin treated-mice. Myeloperoxidase (MPO) which was induced due to bleomycin (p < 0.05) reduced again by high dose of silymarin (0.51 U/min/mg protein). Bleomycin led to an increase in TNF-α and interleukin-6 (IL-6) (7.9 and 11.8 pg/ml). These parameters were reduced by silymarin (p < 0.05).
Conclusions: Silymarin attenuated bleomycin induced-pulmonary toxicity. This protective effect may be due to the ability of silymarin in keeping oxidant–antioxidant balance and regulating of inflammatory mediator release.
Introduction
Oxidative stress consists of cellular and molecular abnormalities caused by overproduction of reactive oxygen species (ROS) as well as reduction in antioxidant defense (Faner et al., Citation2012; Jones, Citation2006). This process plays a key role in expansion of fibrosis in different organs such as lungs. Lungs are also outstandingly susceptible for oxidative damage as they are exposed to the highest level of oxygen. The origins of oxidative stress, which are able to induce pulmonary fibrosis, are exposure to toxicant and drugs such as tobacco, asbestos, silica, and bleomycin (Cheresh et al., Citation2012).
Bleomycin, a glycopeptide antibiotic extracted from Streptomyces verticillus, is commonly used as an adjuvant in chemotherapy protocols to control some human cancers such as germ cell tumor and Hodgkin’s or non-Hodgkin’s malignant lymphoma (Bayer et al., Citation1992; Levi et al., Citation1993). However, the therapeutic application of this anticancer drug is restricted because of its lung toxicity (Martin et al., Citation2005). Bleomycin-lung injury, which is mediated by ROS, eventually leads to pulmonary fibrosis (Mata et al., Citation2003). Bleomycin binds to DNA and in a reaction, which is mediated by molecular oxygen and ferrous ion, causes cleavage in backbone (Burger et al., Citation1981).
In addition to oxidative stress, inflammation is also evident in bleomycin pulmonary damage. Studies have demonstrated that cytokines including interleukin (IL)-1, IL-7, IL-8, IL-10, IL-12, tumor necrosis factor-α (TNF-α), and transforming growth factor-β (TGF-β) effectively take part in collagen deposition over the pulmonary fibrosis (Larki-Harchegani et al., Citation2013).
Silymarin is a mixture of flavonolignans derived from milk thistle [Silybum marianum L. (Asteraceae)] which has antioxidative and anti-inflammatory properties. Silymarin can scavenge free radicals, like hydroxyl, superoxide and hydrogen peroxide, reduces lipid peroxidation, and enhances SOD activity (Hogan et al., Citation2007; Kiruthiga et al., Citation2007). There is evidence that silymarin has protective properties against some toxicants such as halothane, thioacetamide, galactosamine, paladin, and carbon tetrachloride (Mukarram Shah et al., Citation2011). The role of silymarin in a variety of disorders in various organs like CNS, prostate, kidneys, and lung has been established (Gazak et al., Citation2007). Studies on humans and animals have confirmed that the natural antioxidant has no significant adverse effects (Oliveira et al., 2001).
In light of above knowledge, the present study evaluates the protective activity of silymarin following oxidative stress and inflammation induced by bleomycin in mice.
Materials and methods
Animals and groups
Pathogen-free BALB/c mice (20–25 g) were purchased from Pasteur Institute, Tehran, Iran. The mice were kept in temperature-controlled room at 25 °C and on a standard diet and water. All experimental procedures were approved by the Medical Ethics Committee of Tehran Medical University. Twenty-eight mice were randomly divided into four groups (n = 7) including control (intratracheal saline), bleomycin (intratracheal bleomycin), bleomycin plus silymarin (50 and 100 mg/kg b.w).
After anaesthetization, a single dose of bleomycin (1.5 U/kg in normal saline) was administered intratracheally to mice. In silymarin groups, silymarin (Sigma, St. Louis, MO) was dispersed in normal saline +0.5% w/v methylcellulose and following the bleomycin administration, treated intraperitoneally for 10 consecutive days.
Sample collection
The entire animal group was slightly anesthetized on day 10 after bleomycin administration, blood was drawn by cardiac puncture and serum sample was immediately separated. Lung tissue was also quickly removed and processed for homogenization and fractionation.
Lung lipid peroxidation assay
Formation of thiobarbituric acid-reactive substances (TBARS) was measured in lung homogenates (20% w/v) by the method described by Buege and Aust (Citation1978). Briefly, the tissue was homogenized in 100 mM potassium phosphate buffer (pH 7.4) and the homogenate was centrifuged for 5 min at 3000 g. One milliliter of supernatant was mixed with 2 ml of the TBA–TCA–HCl reagent (Thiobarbituric acid 0.37%, 0.25 N HCl and 15% TCA) and vortexed thoroughly. The samples were kept in a boiling water bath for 15 min and then allowed to cool at room temperature. The samples were then centrifuged at 3000 g for 10 min and the absorbance of supernatant was measured at 535 nm by a spectrophotometer. The MDA levels was determined from the extinction coefficient at 535 nm (ε = 1.56 × 105 M−1 cm−1).
Lung reduced glutathione (GSH) assay
The level of lung GSH was measured according to the method of Sedlak and Lindsay (Citation1968). In short, tissue samples were homogenized in 0.02 M EDTA, added an equal volume of 10% TCA to the homogenate, vortexed for 15 min, and centrifuged at 10 000 rpm for 10 min at 4 °C to obtain protein free supernatant. About 0.5 ml of Ellman's reagent (19.8 mg of DTNB in 100 ml of 0.1% sodium nitrate) and 3.0 ml of phosphate buffer (0.2 M, pH 8.0) were added to 1.0 ml of supernatant and the absorbance was measured spectrophotometrically at 412 nm.
Measurement of lung glutathione-S-transferase (GST) activity
GST activity was assayed according to the method of Habig and Jakoby (Citation1981). In this assay 1-chloro-2,4-dinitrobenzene (CDNB) was used as the substrate. The change in absorbance was monitored spectrophotometrically at 340 nm for 3 min. The enzymatic activity was expressed as units/(min mg proteins) using a molar extinction coefficient of 9600 M−1 cm−1.
Measurement of catalase (CAT) activity
CAT activity was determined using a kit (Cayman, Ann Arbor, MI) according to the manufacturer’s instructions. The absorbance was measured at 540 nm and the standard curve was used to calculate the results.
Measurement of lung myeloperoxidase (MPO) activity
In MPO activity, assay of 4-aminoantipyrine/phenol solution was used as the MPO substrate. The absorbance at 510 nm was recorded using a UV–visible spectrophotometer. One unit of enzyme activity was defined as the amount causing degradation of 1 μmol hydrogen peroxide in 1 min at 37 °C. The enzyme activity was expressed as U/g tissue (Wei & Frenkel, Citation1993).
Measurement of serum cytokines (TNF-α and IL-6)
The level of cytokines TNF-α and IL-6 in serum was determined by enzyme-linked immunosorbent assay (ELISA) kit (Abcam, Cambridge, UK) according to manufacturers’ instruction.
Statistical analysis
The experimental results are presented as mean ± standard deviation (SD). Following the assurance of normal distribution of data, a one-way analysis of variance (ANOVA) with the Tukey post hoc test was done in SPSS (17.0) (SPSS Inc., Chicago, IL) (p values < 0.05).
Results
The influence of silymarin treatment on lung lipid peroxidation, formation of thiobarbituric acid reacting substances (TBARS), revealed that the levels of TBARS were elevated in bleomycin-injected mice (∼92%). Silymarin treatment to bleomycin-administered mice resulted in a significant decrease in TBARS (p < 0.05).
As shown in , lung GSH was depleted due to bleomycin administration (83%); while, silymarin treatment leads to a significant increase in pulmonary GSH content (p < 0.05). Our results showed that GSH almost reached to normalcy in rats undergoing high dose silymarin treatment.
Table 1. Effects of silymarin on pulmonary oxidative parameters following bleomycin exposure.
A significant reduction in GST activity was occurred following bleomycin treatment, compared with control mice (48%), whereas high dose of silymarin treatment led to a significant induction in enzyme activity (p < 0.05). Although low dose of silymarin caused an increase in GST activity it was not significant.
Changes in serum CAT activity showed that enzyme activity, which was inhibited due to bleomycin (∼92%), was induced again following a high dose of silymarin injection ().
MPO activity is used as an index for neutrophil infiltration. As shown in , the MPO activity was induced significantly via bleomycin administration in compare with controls (∼70%). However, enzyme activity was suppressed by the higher dose of silymarin (p < 0.05).
Figure 1. Effect of silymarin on pulmonary MPO activity in bleomycin-exposed mice. BLM, bleomycin. MPO activity is expressed as U min/mg of protein. Results are mean ± SD for 7 mice/group. ap < 0.05 versus control group. bp < 0.05 versus BLM group.
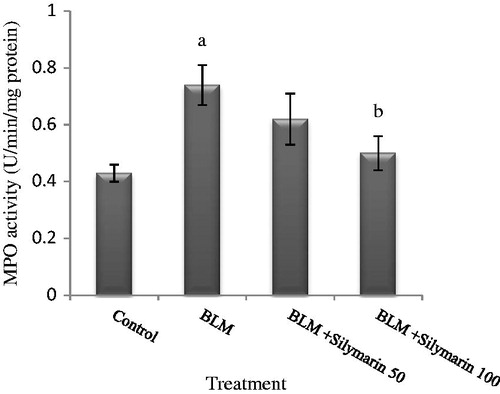
The data obtained from cytokines revealed that a dramatic increase in serum TNF-α level occurred following bleomycin exposure (). Our results show that silymarin administration at a low dose failed to reduce this inflammatory factor (p > 0.05), while treatment of bleomycin-injected rats with high dose of silymarin resulted in a significant depletion of serum TNF-α (∼60%).
Figure 2. Effects of silymarin in serum tumor necrosis factor-alpha (TNF-α) level in animal exposed to bleomycin. BLM, bleomycin. Results are mean ± SD for 7 mice/group. ap < 0.05 versus control group. bp < 0.05 versus BLM group.
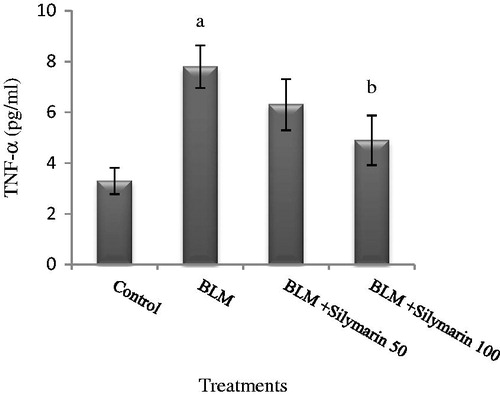
It was found that serum IL-6 was elevated significantly due to bleomycin comparing with the control group (p < 0.05). However, IL-6 decreased in mice subjected to silymarin treatment ().
Discussion
The lung injury following bleomycin administration was defined as changes in some parameters such as a significant elevation in lung lipid peroxidation, MPO activity, TNF-α and IL-6 levels, depletion in GSH content and GST and CAT suppression.
Overproduction of reactive oxygen species as well as decrease in antioxidant capability of the cell leads to tissue oxidative damage. Lipid peroxidation along with the changes in radical scavengers is evidence of oxidative stress occurrence (Khan, Citation2006).
The generation of free radicals led to lipid peroxidation and production of by-products such as MDA which is a marker of lipid peroxidation (Janero, Citation1990; Marnett, Citation1999). Bleomycin exposure caused a significant elevation in pulmonary MDA level compared with control mice which demonstrates improved lipid peroxidation in mice. Our results are in accordance with other studies that have reported lipid peroxidation by exposure to bleomycin (Kara et al., Citation2010; Sener et al., Citation2007; Yildirim et al., Citation2005). Sener et al. (Citation2007) demonstrated that bleomycin lung injury was accompanied by a significant depletion in pulmonary GSH level as well as GST and glutathione peroxidase activity. In this study, they found that administration of resveratrol, an antioxidant agent, can ameliorate oxidative injury and fibrosis due to bleomycin.
GSH (γ-glutamylcysteinylglycine) is an endogenous non-enzyme antioxidant, which is responsible for scavenging of free radicals and preserves the redox status. In addition, GSH plays a central role in catalytic cycles of antioxidant enzymes, such as glutathione peroxidase, glutathione reductase (GR), and GST (Townsend et al., Citation2003). The data obtained from GSH levels following bleomycin treatment showed that bleomycin depleted pulmonary content of GSH (). Depletion in GSH content following oxidative stress can be due to suppression of the enzymes, which participate in GSH synthesis and/or GR activity. Some studies have indicated that loss of GSH will straightly influence the activity of the GR, which is responsible for generation of GSH from GSSG (Tobwala et al., Citation2013).
GST is another antioxidant enzyme, which contributes in cellular defense against ROS generation during oxidative stress of different tissues including lung (Day, Citation2008; Quinlan et al., Citation1994). As indicated in , a significant suppression occurs in GST activity of mice subjected to bleomycin exposure (48%).
In addition to GST, we observed that CAT activity was inhibited due to bleomycin administration. CAT is a free radical scavenging enzyme, which is expressed in various tissues such as lung alveolar epithelial cell and inflammatory cells. This enzyme can reduce H2O2-mediated activation of fibroblasts in idiopathic pulmonary fibrosis (Kliment & Oury, Citation2010). Moreover, it has been shown that CAT administration to asbestos-exposed mice inhibits pulmonary fibrosis through reducing H2O2 generation in Rac 1-activated inflammatory cells (Murthy et al., Citation2009).
The most important sources of TNF-α production are activated monocytes, fibroblasts, and endothelial cells. TNF-α and IL-6, which are the pro-inflammatory factors, are increased by bleomycin administration ( and ). It was also revealed that TNF-α expression increases in lung of mice after bleomycin treatment (Piguet et al., Citation1989). The role of TNF-α during fibrosis process was further established when it was specified that anti-TNF-α noticeably inhibited bleomycin-induced pulmonary fibrosis by down-regulation of pro-fibrotic factors like TGF-β and MCP-1 (Chen et al., Citation2007). MPO, which is frequently found in neutrophil granulocytes, release in response of different stimulatory factors (Kettle et al., Citation1997). We observed prominent lung MPO activity in bleomycin treated animal, demonstrating enhanced PMN activity.
The protective effects of silymarin have been demonstrated against some compounds such as thioacetamide, halothane, and carbon tetrachloride (Mukarram Shah et al., Citation2011). There is strong evidence that silymarin can accomplish its protective actions through various mechanisms like antifibrotic, anti-inflammatory, and antioxidant activity (Chen et al., Citation2009; Nazemian et al., Citation2010; Singh & Agarwal, Citation2004; Yang et al., Citation2003).
In this study, we showed that treatment of bleomycin-administered animals with silymarin causes a significant reverse in bleomycin-induced pulmonary injury. Silymarin treatment resulted in decrease in lung MDA, which is an end product of lipid peroxidation in bleomycin-administered mice. Free radical generation via cell membrane damage can leads to lipid peroxidation product elevation. Silymarin can modulate lipid peroxidation by regulating membrane permeability and increasing the stability of membrane integrity (Pandey & Sahni, Citation2011).
Besides, silymarin administration efficiently restored the depleted levels of GSH in animals subjected bleomycin injection. Silymarin as a natural antioxidant can compensate the cellular glutathione content by preserving the GSH homeostasis (Pradeep et al., Citation2007).
The antioxidant enzymes including CAT and GST which was initially suppressed by bleomycin, induced again due to silymarin treatment. These data corroborate the previously detected pulmonary GSH reduction and lipid peroxidation. Our findings about antioxidant enzymes are in consonant with a prior study which reported that silymarin induce the activity of CAT and superoxide dismutase (Soto et al., Citation2003).
As evidenced in this study, silymarin depressed the elevated pro-inflammatory cytokines in response to bleomycin. It has been demonstrated that silymarin modulates the inflammatory reactions through up-regulation of anti-inflammatory cytokines, like IL-10, IL-12 as well as suppression of leukotrien formation, which is a potent inflammatory mediator (Meeran et al., Citation2006; Schumann et al., Citation2003).
Increased pulmonary MPO activity as the results of bleomycin was effectively inhibited via silymarin treatment. The depression of cytokine release by silymarin is related to infiltration of neutrophil suppression, since MPO activity diminishes in lung tissue.
The reversal of oxidative and inflammatory parameters suggests that the protective mechanism of silymarin involves the inhibition of inflammatory and oxidative mediators.
In conclusion, the present study demonstrates that silymarin attenuated blepmycin-induced pulmonary injury. Although the exact mechanism underlying these actions is unclear, this effect can be attributed to the antioxidant along with anti-inflammatory properties of silymarin. Future studies should focus on molecular mechanisms involved in the modulatory action of silymarin on bleomycin pulmonary damage.
Declaration of interest
The authors report no declarations of interest. The authors alone are responsible for the content and writing of the paper.
References
- Bayer RA, Gaynor ER, Fisher RI. (1992). Bleomycin in non-Hodgkin’s lymphoma. Semin Oncol 19:46–52
- Buege JA, Aust SD. (1978). Microsomal lipid peroxidation. Methods Enzymol 52:302–10
- Burger RM, Peisach J, Horwitz SB. (1981). Activated bleomycin. A transient complex of drug, iron, and oxygen that degrades DNA. J Biol Chem 256:11636–44
- Chen CH, Huang TS, Wong CH, et al. (2009). Synergistic anticancer effect of baicalein and silymarin on human hepatoma HepG2 Cells. Food Chem Toxicol 47:638–44
- Chen K, Wei Y, Sharp GC, Braley-Mullen H. (2007). Decreasing TNF-α results in less fibrosis and earlier resolution of granulomatous experimental autoimmune thyroiditis. J Leukoc Biol 81:306–14
- Cheresh P, Kim SJ, Tulasiram S, Kamp DW. (2012). Oxidative stress and pulmonary fibrosis. Biochim Biophys Acta 1832:1028–40
- Day BJ. (2008). Antioxidants as potential therapeutics for lung fibrosis. Antioxid Redox Signal 10:355–70
- Faner R, Rojas M, Macnee W, Agusti A. (2012). Abnormal lung aging in chronic obstructive pulmonary disease and idiopathic pulmonary fibrosis. Am J Respir Crit Care Med 186:306–13
- Gazak R, Walterova D, Kren V. (2007). Silybin and silymarin – New and emerging applications in medicine. Curr Med Chem 14:315–38
- Habig WH, Jakoby WB. (1981). Assays for differentiation of glutathione S-transferases. Methods Enzymol 77:398–405
- Hogan FS, Krishnegowda NK, Mikhailova M, Kahlenberg MS. (2007). Flavonoid, silibinin, inhibits proliferation and promotes cell-cycle arrest of human colon cancer. J Surg Res 143:58–65
- Janero DR. (1990). Malondialdehyde and thiobarbituric acid-reactivity as diagnostic indices of lipid peroxidation and peroxidative tissue injury. Free Radic Biol Med 9:515–40
- Jones DP. (2006). Extracellular redox state: Refining the definition of oxidative stress in aging. Rejuvenation Res 9:169–81
- Kara H, Karatas F, Tug T, et al. (2010). Protective effect of octreotide on intra-tracheal bleomycin-induced oxidative damage in rats. Exp Toxicol Pathol 62:235–41
- Kettle AJ, Van Dalen CJ, Winterbourn CC. (1997). Peroxynitrite and myeloperoxidase leave the same footprint in protein nitration. Redox Rep 3:257–8
- Khan SM. (2006). Protective effect of black tea extract on the levels of lipid peroxidation and antioxidant enzymes in liver of mice with pesticideinduced liver injury. Cell Biochem Funct 24:327–32
- Kiruthiga PV, Shafreen RB, Pandian SK, et al. (2007). Protective effect of silymarin on erythrocyte hemolysate against benzo(a)pyrene and exogenous reactive oxygen species (HO) induced oxidative stress. Chemosphere 68:1511–18
- Kliment CR, Oury TD. (2010). Oxidative stress, extracellular matrix targets, and idiopathic pulmonary fibrosis. Free Radic Biol Med 49:707–17
- Larki-Harchegani A, Hemmati AA, Arzi A, et al. (2013). Evaluation of the effects of caffeic acid phenethyl ester on prostaglandin E2 and two key cytokines involved in bleomycin-induced pulmonary fibrosis. Iran J Basic Med Sci 16:850–7
- Levi JA, Raghavan D, Harvey V, et al. (1993). The importance of bleomycin in combination chemotherapy for good-prognosis germ cell carcinoma. Australasian germ cell trial group. J Clin Oncol 11:1300–5
- Marnett LJ. (1999). Lipid peroxidation-DNA damage by malondialdehyde. Mutat Res 424:83–95
- Martin WG, Ristow KM, Habermann TM, et al. (2005). Bleomycin pulmonary toxicity has a negative impact on the outcome of patients with Hodgkin’s lymphoma. J Clin Oncol 23:7614–20
- Mata M, Ruız A, Cerda M, et al. (2003). Oral N-acetylcysteine reduces bleomycin-induced lung damage and mucin Muc5ac expression in rats. Eur Respir J 22:900–5
- Meeran SM, Katiyar S, Elmets CA, Katiyar SK. (2006). Silymarin inhibits UV radiation-induced immunosuppression through augmentation of interleukin-12 in mice. Mol Cancer Ther 5:1660–8
- Mukarram Shah SM, Khan FA, Hassan Shah SM, et al. (2011). Evaluation of phytochemicals and antimicrobial activity of white and blue capitulum and whole plant of Silybum Marianum. World Appl Sci J 12:1139–44
- Murthy S, Adamcakova-Dodd A, Perry SS, et al. (2009). Modulation of reactive oxygen species by Rac1 or catalase prevents asbestos-induced pulmonary fibrosis. Am J Physiol Lung Cell Mol Physiol 297:846–55
- Nazemian F, Karimi G, Moatamedi M, et al. (2010). Effect of silymarin administration on TNF-α serum concentration in peritoneal dialysis patients. Phytother Res 24:1654–7
- Oliveira C, Lopasso FP, Laurindo F, et al. (2001). Protection against liver ischemiareperfusion injury in rats by silymarin or verapamil. Transplant Proc 33:3010–14
- Pandey G, Sahni YP. (2011). A review on hepatoprotective activity of silymarin. IJRAP 2:75–9
- Piguet PF, Collart MA, Grau GE, et al. (1989). Tumor necrosis factor/cachectin plays a key role in bleomycin-induced pneumopathy and fibrosis. J Exp Med 170:655–63
- Pradeep K, Mohan CVR, Gobianand K, Karthikeyan S. (2007). Silymarin modulates the oxidant–antioxidant imbalance during diethylnitrosamine induced oxidative stress in rats. Eur J Pharmacol 560:110–16
- Quinlan T, Spivack S, Mossman BT. (1994). Regulation of antioxidant enzymes in lung after oxidant injury. Environ Health Perspect 2:79–87
- Schumann J, Prockl J, Kiemer AK, et al. (2003). Silibinin protects mice from T cell-dependent liver injury. J Hepatol 39:333–40
- Sedlak J, Lindsay RH. (1968). Estimation of total protein bound and non-protein sulfhydryl groups in tissue with Ellman’s reagent. Anal Biochem 25:192–205
- Sener G, Topaloglu N, Sehirli AO, et al. (2007). Resveratrol alleviates bleomycin-induced lung injury in rats. Pulm Pharmacol Ther 20:642–9
- Singh RP, Agarwal R. (2004). Prostate cancer prevention by silibinin. Curr Cancer Drug Targets 4:1–11
- Soto C, Recoba R, Barron H, et al. (2003). Silymarin increases antioxidant enzymes in alloxan-induced diabetes in rat pancreas. Comp Biochem Physiol C Toxicol Pharmacol 136:205–12
- Tobwala S, Fan W, Stoeger T, Ercal N. (2013). N-Acetylcysteine amide, a thiol antioxidant, prevents bleomycin-induced toxicity in human alveolar basal epithelial cells (A549). Free Radic Res 47:740–9
- Townsend, DM, Tew KD, Tapiero H. (2003). The importance of glutathione in human disease. Biomed Pharmacother 57:145–55
- Wei H, Frenkel K. (1993). Relationship of oxidative events and DNA oxidation in Sencar mice to in vivo promoting activity of phorbol ester-type tumor promoters. Carcinogenesis 14:1195–201
- Yang SH, Lin JK, Chen WS, Chiu JH. (2003). Antiangiogenic effect of silymarin on colon cancer LoVo cell line. J Surg Res 113:133–8
- Yildirim Z, Kotuk M, Iraz M, et al. (2005). Attenuation of bleomycin-induced lung fibrosis by oral sulfhydryl containing antioxidants in rats: Erdosteine and N-acetylcysteine. Pulm Pharmacol Ther 18:367–73