Abstract
Context: Cactus pear (Opuntia ficus-indica (L.) Mill. (Cactaceae)) is a medicinal plant widely used to treat diabetes.
Objective: This work investigates the hypoglycemic and antihyperglycemic effect of cactus pear seed oil (CPSO), its mechanism of action, and any toxic effects.
Materials and methods: The hypoglycemic effect of CPSO was evaluated in groups of six healthy Wistar rats given 1 or 2 ml kg−1 orally and compared with groups receiving glibenclamide (2 mg kg−1) or water. Glycemia was determined after 30, 60, 120, 240, and 360 min. The antihyperglycemic effect of CPSO was determined in healthy rats and in streptozotocin-induced diabetic rats (STZ); normal rats received 0.8 ml kg−1 CPSO, while diabetic rats received 1 ml kg−1 CPSO, their controls received water or 2 mg kg−1 glibenclamide. For the antihyperglycemic effect evaluation, all the animals were fasted for 16 h before treatment and received glucose orally at 1 g kg−1 30 min after treatment; blood was taken after 30, 90, 150, and 210 min. Intestinal glucose absorption was estimated in rat jejunum perfused with a solution containing 5.55 mmol l−1 glucose. Acute toxicity was determined in albino mice that received oral or intraperitoneal doses of 1, 3, or 5 ml kg−1 CPSO.
Results: CPSO (p.o.) decreased postprandial hyperglycemia (60 min after glucose loading), 40.33% and 16.01%, in healthy and STZ-diabetic glucose-loaded rats, respectively. CPSO, also, significantly decreased intestinal glucose absorption by 25.42%. No adverse effects were seen in mice administered CPSO at up to 5 ml kg−1.
Conclusion: CPSO is antihyperglycemic. The effect can be explained partly by inhibition of intestinal glucose absorption.
Introduction
The International Diabetes Federation (IDF) estimated that 382 million people have diabetes in 2013; by 2035 this will rise to 592 million (IDF, Citation2013). Diabetes mellitus is a complicated metabolic disorder resulting from heterogeneous factors. Impaired glucose metabolism is the most known diabetes’ symptom, which is due to a lack of insulin secretion, impaired insulin activity or both (Dinneen, Citation2006). Generally, metabolic impairment caused by diabetes induces harmful complications, such as blindness, limb amputation, and finally death (Marles & Farnsworth, Citation1995), which are exacerbated by glucotoxicity due to a permanently elevated blood glucose level (Bensellam et al., Citation2012; Rosenthal, Citation2007). Improving glucose tolerance may ameliorate the patient’s condition and delay or prevent complications, and preventing post-prandial hyperglycemia can improve glucose tolerance. Not only common hypoglycemic agents but also the quality of the diet can inhibit post-prandial hyperglycemia in both diabetic and normal people. Therefore, functional foods, such as plants extracts, administered before meals can have a beneficial effect on hyperglycemia. Vegetable oils can improve cellular glucose tolerance and thus decrease blood glucose level (Foster et al., Citation2009).
Cactus pear [Opuntia ficus-indica (L.) Mill. (Cactaceae)], Barbary fig or prickly pear is endemic to Mexico (Griffith, Citation2004) and is popular throughout the Mediterranean area and Morocco. The fresh fruits are widely consumed, and parts are used as functional foods, therapeutics, and animal food (Feugang et al., Citation2006). Many studies (Deters et al., Citation2012; Feugang et al., Citation2010; Kim et al., Citation2012; Tesoriere et al., Citation2004; Zou et al., Citation2005) have shown that cactus pears can reduce oxidative stress and may prevent cancer.
Cactus pear seed oil (CPSO) is an organic extract that contains fatty acids, represented by linoleic acid, a major polyunsaturated fatty acid, oleic acid, the dominant monounsaturated fatty acid, and palmitic acid, the major saturated fatty acid (Ennouri et al., Citation2005; Ramadan & Morsel, Citation2003; Sawaya & Khan, Citation1982). CPSO supplementation of the diet of normal rats decreased blood glucose, increased liver and muscle glycogen, and decreased low-density lipoprotein cholesterol (Ennouri et al., Citation2006a, Citation2007). Furthermore, O. ficus-indica is reputed to be antidiabetic (El-Hilaly et al., Citation2003; Marles & Farnsworth, Citation1995; Tahraoui et al., Citation2007). Both cladodes extract and stemfruit combinations lowered plasma sugar in a healthy rat model (Butterweck et al., Citation2011), and cactus pear polysaccharides were beneficial in streptozotocin (STZ)-induced diabetic rats (Liu et al., Citation2010). Seed powder and seed oil from this plant reduced plasma glucose levels, improved the hepatic and plasma lipid profile and reduced cholesterolemia and hepatic and muscular glycogen in healthy rats treated subchronically (Ennouri et al., Citation2006a,Citationb, Citation2007).
The present work investigates the hypoglycemic and antihyperglycemic effects of CPSO, its possible mechanism of action, and any toxic effects in normal and diabetic rats.
Materials and methods
Animals
Healthy adult Wistar rats of each sex weighing 170–230 g were housed in polypropylene cages at the animal house of the Faculty of Sciences, Mohammed I University, Oujda, Morocco. The animals were maintained in environmental conditions and fed standard diet and water ad libitum.
All animals were cared for in compliance with the Guide for the Care and Use of Laboratory Animals, US National Institutes of Health (Citation2012).
Acute toxicity
Oral and intraperitoneal acute toxicities were determined in albino mice weighing 20–30 g. For each route, four groups of six mice (three males and three females) received increasing doses of CPSO (1, 3, and 5 ml kg−1) or distilled water (10 ml kg−1). After treatment, the animals were observed daily for 14 d to assess behavioral and autonomic toxic effects.
Hypoglycemic and antihyperglycemic effects
Experimental diabetes was induced by a single intraperitoneal injection of 60 mg kg−1 streptozotocin (STZ, Sigma-Aldrich, Hamburg, Germany) freshly dissolved in citrate buffer (0.1 mol L−1 phosphate, 1 mol L−1 citrate, pH 4.5). Animals with a fasting blood glucose level >1.5 g L−1 on day 7 after injection were considered diabetic (Wu & Huan, Citation2008) and were included in the study.
Hypoglycemic and antihyperglycemic effects were assessed in normal and STZ-induced diabetic rats fasted for 16 h with free access to water.
For determination of the hypoglycemic effects of CPSO, blood was withdrawn from the tail vein under light ether anesthesia and centrifuged in a hematocrit centrifuge (Hermle Z 230H, HERMLE, Gosheim, Germany) for 10 min; the plasma was used to determine glucose levels by the glucose oxidase–peroxidase method using a commercial kit (Glucose, Biosystems, Barcelona, Spain). In this experiment, normal rats were divided in four groups of six rats each, which received by oral gavage CPSO (Argan Oil Company, Casablanca, Morocco) at 1 or 2 ml kg−1, glibenclamide (purchased from a local pharmacy as Benclamide 5 mg) at 2 mg kg−1 or distilled water (10 ml kg−1). Blood glucose was estimated at 30, 60, 120, 240, and 360 min after treatment (Bellahcen et al., Citation2012).
For determination of the antihyperglycemic effects of CPSO, healthy and STZ-induced diabetic rats were divided into three groups of six rats each. Normal rats received CPSO by oral gavage at 0.8 ml kg−1, diabetic rats received CPSO at 1.0 ml kg−1, healthy and diabetic rats received glibenclamide at 2 mg kg−1, and healthy and diabetic controls received distilled water at 10 ml kg−1. All the animals were orally loaded with glucose (1 g kg−1) 30 min after treatments. Blood was sampled by tail vein incision 30, 90, 150, and 210 min after treatment.
Intestinal perfusion
Rats were fasted for 36 h, then anaesthetized by intramuscular injection of sodium pentobarbital (50 mg kg−1) and placed on a homeothermic pad to maintain the body temperature at 37 °C. Laparotomy was performed by a midline incision through an abdominal muscle, and a jejunum segment measuring approximately 10 cm was catheterized with polyethylene tubes at both proximal and distal ends. After catheterization, the incision was sutured and the surgical area covered with cotton imbibed with saline (0.9% NaCl) to prevent dehydration. A solution of 126.1 mmol L−1 NaCl, 2.70 mmol L−1 KCl, 0.4 mmol L−1 NaH2PO4·2H2O, 1.04 mmol L−1 MgCl2·6H2O, 7.14 mmol L−1 NaHCO3, 6.93 mmol L−1 CaCl2, and 5.55 mmol L−1 glucose (pH 7.5) was perfused through the intestinal lumen with a peristaltic pump (Thermo Fisher Scientific Inc., Waltham, MA) at a constant flow rate (0.53 ml min−1) and temperature (37 °C) for 10 min to clean out any residual material and to allow intestinal adaptation; the main perfusion was carried out for 1 h. An aliquot of the perfusate was collected for glucose determination (Bnouham et al., Citation2003), the length of the perfused segment was measured, and the rats were euthanized. Intestinal glucose absorption was estimated in mg 10 cm−1 h−1.
Four groups of rats were used: one received the perfusion solution with added CPSO (1 ml kg−1); one received the solution with added emulsified CPSO (0.36% DMSO, 1% Tween 20); one received the solution with added acarbose (3 mg kg−1), a standard inhibitor of d-glucose luminal absorption (Hirsh et al., Citation1997); and one group rat served as the control, receiving the solution alone.
Statistical analysis
The results are expressed as means ± standard errors of the means (SEMs). Student's t-test was used, and differences were considered statistically significant at p < 0.05.
Results
Acute toxicity
After oral or intraperitoneal administration of CPSO at a dose of 1, 3, or 5 ml kg−1, no mortality was seen, and there were no behavioral or autonomic effects throughout the 14-d period of observation.
Hypoglycemic effect
Oral administration of CPSO at 1 or 2 ml kg−1 did not significantly affect the fasting blood glucose level of healthy rats, although glibenclamide induced a significant (p ≤ 0.001) decrease in blood glucose level (0.60 ± 0.05 g L−1) at 360 min after its administration ().
Table 1. Changes in blood glucose level variation during 6 h after oral administration of cactus pear seed oil (CPSO) at 1 or 2 ml kg−1 and of glibenclamide at 2 mg kg−1 in healthy rats.
Antihyperglycemic effect
Oral administration of CPSO at 0.8 ml kg−1 to healthy rats significantly inhibited (p ≤ 0.001) the hyperglycemia that follows glucose loading, 1.35 ± 0.14 g L−1 at 90 min for treated rats and 2.27 ± 016 g L−1 for a normal control group. Furthermore, the area under the curve (AUCglucose) was significantly smaller (286.3 ± 24.18 g L−1 min−1) than that of normal controls (390.1 ± 22.59 g L−1 min−1) (). In the STZ-diabetic rats, oral administration of CPSO improved the glucose tolerance in the STZ-diabetic treated group (3.56 ± 0.20 g L−1) at 90 min, compared with the diabetic control group (4.24 ± 0.14 g L−1), and the AUCglucose of CPSO-treated diabetic rats (789 ± 15.79 g L−1 min−1) was significantly smaller than that of diabetic controls (798.9 ± 15.79 g L−1 min−1) ().
In situ single-pass intestinal perfusion
The absorption of d-glucose by the perfused intestinal jejunum segment is shown in . Perfusion of emulsified CPSO significantly decreased (p ≤ 0.05) glucose absorption through the perfused jejunum segment (8.44 ± 0.92 mg 10 cm−1 h−1) compared with normal control group (11.32 ± 0.97 mg 10 cm−1 h−1).
Figure 3. Effect of cactus pear seed oil (CPSO) on free d-glucose absorption assessed by in situ single-pass intestinal perfusion. Data are means ± SEM (n = 6). *p ≤ 0.05; **p ≤ 0.001 compared with normal controls.
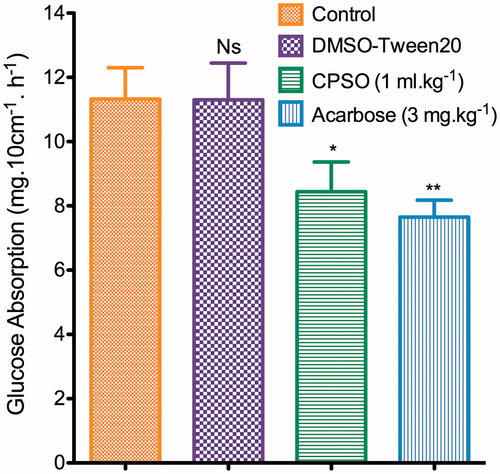
Acarbose (3 mg kg−1) induced a greater inhibitory effect (p ≤ 0.001).
Discussion
The results show that oral administration of CPSO can improve glucose tolerance by decreasing postprandial hyperglycemia in healthy glucose-loaded rats and STZ-induced diabetic glucose-loaded rats, but it has no effect on the basal glucose level of healthy rats fasted overnight. Also, CPSO partially decreased d-glucose intestinal absorption. The result of the acute oral toxicity test shows that the product is safe at doses up to 5 ml kg−1.
It has been reported that the lipid fraction of O. ficus-indica seed oil contains polyunsaturated fatty acids (linoleic acid) and monounsaturated fatty acids (oleic acid), and the unsaponifiable fraction contains liposoluble vitamins (tocopherols, vitamin K1), sterols, and carotenoids (β-carotene) (Ennouri et al., Citation2005; Mannoubi et al., Citation2009; Oguzhan et al., Citation2006; Ramadan & Morsel, Citation2003). The omega-3 fatty acids increase the insulin secretion stimulated by d-glucose by contact with Langerhans islet beta cells (Oguzhan et al., Citation2006). In addition, polyunsaturated fatty acids enhance cell membrane fluidity and GLUT4 transporter expression (Manco et al., Citation2004). Moreover, polyunsaturated fatty acids improve insulin-induced glucose uptake on insulin-sensitive cells (3T3-L1 adipocytes) by increasing GLUT1 and GLUT4 plasma membrane density (Nugent et al., Citation2001). This mechanism may be responsible for enhanced glucose-induced insulin secretion. An amplified insulin reaction might be involved in the rapid inhibition of postprandial hyperglycemia by CPSO.
It has been reported that antioxidants not only affect the plasma stability of unsaturated fatty acids but also improve the insulin sensitivity of L6 muscle cells (Vinayaga Moorthi et al., Citation2006). The β-carotene plasma level is inversely correlated to the fasting plasma glucose level, and carotenoids are involved in diabetes control (Abahusain et al., Citation1999). All these consideration lead us to suggest that the acute postprandial glucose-lowering action of CPSO can be explained by increased intra-pancreatic glucose-induced insulin release from β-cells, extrapancreatic enhancement of glucose uptake by insulin sensitive tissues, or both. This extrapancreatic effect of CPSO may be due to the presence of fatty acids of various degrees of unsaturation (Barra et al., Citation1995). It is known that fatty acids disturb the absorption function of enteric cells when they are present in the luminal space.
Conclusion
The present study confirms that CPSO has an anti-postprandial hyperglycemic effect in normal and STZ-induced diabetic rats. This effect should prevent the complications of glucotoxicity and is due, in part, to inhibition of intestinal d-glucose absorption. Further in vitro tests and subchronic and chronic assays are required to confirm this acute effect on glucose metabolism and to assess the effect of CPSO in primary or secondary prevention of diabetes mellitus of both types.
Declaration of interest
The authors declare that there is no conflict of interest and they are alone responsible of the content of this article. This work was supported by grants from CNRST, Morocco [Project URAC-40] and from Belgium [Program 3, CUD Project].
Acknowledgements
The authors are thankful to Badraoui Mustapha and Ramdaoui Karim for technical help and animal breeding. The authors are thankful to Pr. Elizabeth Heseltine (editor for WHO and various other international organizations and national institutes in United Kingdom), for English correction.
References
- Abahusain MA, Wright J, Dickerson JW, De Vol EB. (1999). Retinol, alpha-tocopherol and carotenoids in diabetes. Eur J Clin Nutr 53:630–5
- Barra IG, Escobar M, Guerra MV. (1995). Influence of intake of vegetables oils on the in vitro intestinal absorption of glucose in golden hamster. Arch Latinoam Nutr 45:290–4
- Bellahcen S, Mekhfi H, Ziyyat A, et al. (2012). Prevention of chemically induced diabetes mellitus in experimental animals by virgin argan oil. Phytother Res 26:180–5
- Bensellam M, Laybutt DR, Jonas JC. (2012). The molecular mechanisms of pancreatic beta-cell glucotoxicity: Recent findings and future research directions. Mol Cell Endocrinol 364:1–27
- Bnouham M, Merhfour FZ, Ziyyat A, et al. (2003). Antihyperglycemic activity of the aqueous extract of Urtica dioica. Fitoterapia 74:677–81
- Butterweck V, Semlin L, Feistel B, et al. (2011). Comparative evaluation of two different Opuntia ficus-indica extracts for blood sugar lowering effects in rats. Phytother Res 25:370–5
- Deters AM, Meyer U, Stintzing FC. (2012). Time-dependent bioactivity of preparations from cactus pear (Opuntia ficus indica) and ice plant (Mesembryanthemum crystallinum) on human skin fibroblasts and keratinocytes. J Ethnopharmacol 142:438–44
- Dinneen SF. (2006). What is diabetes? Medicine 34:45–6
- El-Hilaly J, Hmammouchi M, Lyoussi B. (2003). Ethnobotanical studies and economic evaluation of medicinal plants in Taounate province (Northern Morocco). J Ethnopharmacol 86:149–58
- Ennouri M, Evelyne B, Laurence M, Hamadi A. (2005). Fatty acid composition and rheological behaviour of prickly pear seed oils. Food Chem 93:431–7
- Ennouri M, Fetoui H, Bourret E, et al. (2006a). Evaluation of some biological parameters of Opuntia ficus indica. 1. Influence of a seed oil supplemented diet on rats. Bioresour Technol 97:1382–6
- Ennouri M, Fetoui H, Bourret E, et al. (2006b). Evaluation of some biological parameters of Opuntia ficus indica. 2. Influence of seed supplemented diet on rats. Bioresour Technol 97:2136–40
- Ennouri M, Fetoui H, Hammami M, et al. (2007). Effects of diet supplementation with cactus pear seeds and oil on serum and liver lipid parameters in rats. Food Chem 101:248–53
- Feugang JM, Konarski P, Zou D, et al. (2006). Nutritional and medicinal use of Cactus pear (Opuntia spp.) cladodes and fruits. Front Biosci 11:2574–89
- Feugang JM, Ye F, Zhang DY, et al. (2010). Cactus pear extracts induce reactive oxygen species production and apoptosis in ovarian cancer cells. Nutr Cancer 62:692–9
- Foster R, Williamson CS, Lunn J. (2009). Briefing Paper: Culinary oils and their health effects. Nutr Bull 34:4–47
- Griffith MP. (2004). The origins of an important cactus crop, Opuntia ficus-indica (Cactaceae): New molecular evidence. Am J Bot 91:1915–21
- Hirsh AJ, Yao SY, Young JD, Cheeseman CI. (1997). Inhibition of glucose absorption in the rat jejunum: A novel action of alpha-d-glucosidase inhibitors. Gastroenterology 113:205–11
- International Diabetes Federation. (2013). IDF Diabetes Atlas. 5th ed. Brussels: International Diabetes Federation. Available from: www.idf.org/diabetesatlas [last accessed Nov 2013]
- Kim SH, Jeon BJ, Kim DH, et al. (2012). Prickly pear cactus (Opuntia ficus indica var. saboten) protects against stress-induced acute gastric lesions in rats. J Med Food 15:968–73
- Liu HG, Liang QY, Meng HL, Huang HX. (2010). Hypoglycemic effect of extracts of cactus pear fruit polysaccharide in rats. Zhong Yao Cai 33:240–2
- Manco M, Calvani M, Mingrone G. (2004). Effects of dietary fatty acids on insulin sensitivity and secretion. Diabetes Obes Metab 6:402–13
- Mannoubi I, Barrek S, Skanji T, et al. (2009). Characterization of Opuntia ficus indica seed oil from Tunisia. Chem Nat Comp 45:616–20
- Marles RJ, Farnsworth NR. (1995). Antidiabetic plants and their active constituents. Phytomedicine 2:137–89
- National Institutes of Health (NIH). (2012). Guide for the Care and Use of Laboratory Animals. Publication 5–23, revised 2012. Available from: http://grants.nih.gov/grants/olaw/Guiding_Principles_2012.pdf [last accessed 30 Dec 2012]
- Nugent C, Prins JB, Whitehead JP, et al. (2001). Arachidonic acid stimulates glucose uptake in 3T3-L1 adipocytes by increasing GLUT1 and GLUT4 levels at the plasma membrane. Evidence for involvement of lipoxygenase metabolites and peroxisome proliferator-activated receptor gamma. J Biol Chem 276:9149–57
- Oguzhan B, Zhang Y, Louchami K, et al. (2006). Pancreatic islet function in omega 3 fatty acid-depleted rats – Glucose metabolism and nutrient-stimulated insulin release. Endocrine 29:457–66
- Ramadan MF, Morsel MT. (2003). Oil cactus pear (Opuntia ficus-indica L.). Food Chem 82:339–45
- Rosenthal MJ. (2007). Diabetes. In: Editor-in-Chief: James EB, ed. Encyclopedia of Gerontology, 2nd ed. New York: Elsevier, 409–17
- Sawaya WN, Khan P. (1982). Chemical characterization of prickly pear seed oil, Opuntia ficus-indica. J Food Sci 47:2060–1
- Tahraoui A, El-Hilaly J, Israili ZH, Lyoussi B. (2007). Ethnopharmacological survey of plants used in the traditional treatment of hypertension and diabetes in South-Eastern Morocco (Errachidia province). J Ethnopharmacol 110:105–17
- Tesoriere L, Butera D, Pintaudi AM, et al. (2004). Supplementation with cactus pear (Opuntia ficus-indica) fruit decreases oxidative stress in healthy humans: A comparative study with vitamin C. Am J Clin Nutr 80:391–5
- Vinayaga Moorthi R, Bobby Z, Selvaraj N, Sridhar MG. (2006). Vitamin E protects the insulin sensitivity and redox balance in rat L6 muscle cells exposed to oxidative stress. Clin Chim Acta 367:132–6
- Wu KK, Huan Y. (2008). Streptozotocin-induced diabetic models in mice and rats. Curr Protoc Pharmacol 40:1–14
- Zou DM, Brewer M, Garcia F, et al. (2005). Cactus pear: A natural product in cancer chemoprevention. Nutr J 4:25–37