Abstract
Context: Marine cyanobacteria offer considerable potential to isolate new antimalarials to meet a pressing need of our times.
Objective: To explore the antiplasmodial properties of marine cyanobacteria.
Materials and methods: Cyanobacterial samples collected from the coastal regions of Tamil Nadu were identified using light microscopy, and the strains were cultivated in ASN-III medium. Organic extracts (0–100 µg mL−1) of 25 in vitro mass-cultivated cyanobacteria, prepared using methanol: chloroform mixture (1:1 v/v) were evaluated for their antiplasmodial activity against chloroquine-sensitive and -resistant strains of Plasmodium falciparum by fluorescence-based SYBR Green I assay where chloroquine was used as a control. To detect the toxic effects of cyanobacterial extracts against red blood cells, the invasion, maturation, and growth rate of malarial parasites in cyanobacterial extracts pre-treated versus untreated erythrocytes were quantified microscopically. Mammalian cell line (HeLa) was used to determine cyanobacterial extract toxicity using the MTT assay.
Results: The extracts of Lyngbya aestuarii Liebm. ex Gomont CNP 1005 (C12) Oscillatoria boryana BDU 91451 (C22) and Oscillatoria boryana Bory ex Gomont BDU 141071 (C18) showed promising antiplasmodial activity (IC50 = 18, 18, and 51 μg mL−1 respectively) against Pf3D7. Pretreatment of red blood cells with IC100 of C12, C18, and C22 (40, 100, and 40 µgmL−1, respectively) did not significantly influence the invasion, maturation, and growth rate of malarial parasites in comparison with untreated RBC controls suggesting a lack of toxicity to host cells. MTT assay based IC50 (>200 μg mL−1) of these extracts against HeLa cell line also indicates their high selectivity against the malaria parasite.
Discussion and conclusion: These exploratory studies suggest the possibilities of development of new antimalarial compounds from marine cyanobacteria.
Introduction
Exploring organisms for their pharmacological properties is expected to provide new pharmacophores against diseases (Newman & Cragg, Citation2007). Marine organisms need to be explored for their biological activities as several reports indicate them to possess antimicrobial, anticancer, and antitumor activities (Fattorusso & Taglialatela-Scafati, Citation2009). From a perspective of new drug discovery, marine cyanobacteria are particularly fascinating due to the ease of their availability (Nunnery et al., Citation2010) and amenability of most strains to grow in culture conditions.
Cyanobacteria are ancient diverse organisms common in aquatic and terrestrial environments. They are photosynthetic like eukaryotic algae and higher plants, but their cellular organizations are akin to bacteria (Vincent, Citation2009). Cyanobacteria are extremely rich in secondary metabolites exhibiting various biological activities. More than 800 secondary metabolites from marine cyanobacteria including linear and cyclic peptides, linear and cyclic depsipeptides, lipopeptides, lipodepsipeptides, ribosomal peptides, and fatty acid amides (Costa et al., Citation2012) have been reported for their promising clinical activities such as antimicrobial, anticancer, antitumor, anti-inflammatory, and antimicrotubule (Nagarajan et al., Citation2012; Yadav et al., Citation2011). Several toxins including hepatotoxins, neurotoxins, cytotoxins, dermatotoxins, and irritant toxins have been isolated from the genera of Microcystis, Anabaena, Nostoc, Oscillatoria, Cylindrospermum, Cylindrospermopsis, Aphanizomenon, and Nodularia (Tan, Citation2007; Yadav et al., Citation2011). These toxins have been used as inhibitors of several enzymes (Yadav et al., Citation2011), protein synthesis (Froscio et al., Citation2003), and eukaryotic voltage-gated sodium channels (Tan, Citation2007).
More interestingly, marine Moorea producens (earlier known as Lyngbya majuscula Harvey in Hooker ex Gomont) has been found to exhibit potent antimalarial activity against Plasmodium falciparum and a number of antimalarial compounds such as lagunamides (Tripathi et al., Citation2010a), dragomabin (McPhail et al., Citation2007), and malyngolide (Gutiérrez et al., Citation2010) have been isolated from it. Likewise, antimalarial screening of 18 cyanobacterial strains showed that the methylene dichloride extract of Phormidium ectocarpi inhibited the malaria parasite (IC50 = 2.1 µg mL−1) (Papendorf et al., Citation1998). Similarly, crude extracts and secondary metabolites of several marine cyanobacteria have been found to be inhibiting malaria parasites in the sub-micromolar range (). World Health Organization has reported 214 million cases of malaria throughout the world including 655 000 deaths in 2011 (World Malaria Report, Citation2011). Furthermore, the rapid spread of resistance against antimalarial drugs marks the urgency for discovery of new molecular scaffolds against malaria (Burrows et al., Citation2011; Dondorp et al., Citation2000; Noedl et al., Citation2008; White, Citation2010). In this study, we have isolated and identified several cyanobacterial species from coastal regions of Tamil Nadu, India, to explore their potential antiplasmodial activity. The primary criterion for selection of cyanobacterial species in our work was the ability to grow them under laboratory conditions. Although cyanobacterial species have been studied for antiplasmodial activity, this is the first report of the antiplasmodial action of extracts of cyanobacterial species from the various coastal regions of Tamil Nadu. Further, it has been confirmed that the antiplasmodial action of the extracts is specific to the parasite since the extracts tested were found to have no significant toxicity against either the red blood cells or the HeLa cells in culture.
Table 1. Antimalarial properties of marine cyanobacterial secondary metabolites.
Materials and methods
Sample collection
Cyanobacterial samples were collected in September 2008, October 2009, and December 2010 from the coastal regions of Tamil Nadu including Mandapam (9°16′55″N, 79°8′45″E), Dhanushkodi (9°14′55″N, 79°19′0″E), Thondi (9°46′35″N, 79°0′28″E), and Kanyakumari (8°04′41″N, 77°32′28″E). Furthermore, cyanobacterial samples from mangrove plants {Avicennia marina (Forssk.) Vierh., Aegiceras corniculatum (L.) Blanco, and Rhizophora apiculata Blume} were collected by scrapings from Pichavaram (11°25′47″N, 79°47′29″E) and Muthupettai (10°23′54″N, 79°30′34″E) (). Solid substrata, such as mollusc shells, sand, pieces of macroalgae, small rocks, fragments of coral reefs, sponges, boats, mangrove roots, objects that appeared blue-green in color, and sea water samples, were also collected (). Live samples were transferred into polypropylene bags containing sea water collected from the same site and transported immediately to the laboratory.
Table 2. Morphological features of cyanobacterial species and their antiplasmodial activity against Pf3D7.
Isolation and identification of cyanobacteria
Collected cyanobacteria were observed under a microscope and identified by their morphological features. In each case, a cyanobacterial sample of 1 g was suspended in 5 mL of ASN III medium and homogenized thoroughly using tissue homogenizer for 1 min at 27 ± 2 °C. Homogenized samples (100 µL) were transferred into 0.9% agar-containing ASN III medium petriplates and spread uniformly. The plates were kept in an illuminated culture rack with a light intensity of 16.2–20.25 μmol photons m−2 s−1and maintained at 27 ± 2 °C. The plates were observed regularly under a microscope at 10× magnification. Finally, a single strain was isolated by continuous subculturing in fresh ASN III media-containing petriplates. To avoid eukaryotic algal and fungal contamination, the cyanobacterial culture was treated with cycloheximide (100 µg mL−1) and amphotericin B (300 µg mL−1) for 2 d with intermittent shaking. The genus of isolated species was identified and photographs were taken by using binocular microscope inverted epifluorescence Model XTS, Via della Meccanica, Italy, at 100× magnification (Desikachari, Citation1959). In addition, three more cyanobacterial cultures (C18, C22, and C25) were obtained from the Germplasm of the National Facility for Marine Cyanobacteria (NFMC), Bharathidasan University, India, and mass-cultivated for extraction.
Phylogenetic analysis of selected cyanobacteria
Isolation of genomic DNA
The DNA isolation method from plant developed by Doyle and Doyle (Citation1987) was followed with certain modifications. Approximately, 1 g cells of Lyngbya aestuarii CNP 1005 were washed and centrifuged the content at 14 000 rpm for 10 min. The pellet was washed twice with sterile Cetyl trimethylammonium bromide (CTAB) buffer (10% 1 M Tris, pH 8.0; 4% 0.5 M EDTA; 28% 5 M NaCl; 2% CTAB) and homogenized by using a glass homogenizer. The homogenate was freeze thawed three times. Then the suspension was centrifuged at 10 000 rpm for 10 min. The supernatant was removed and subsequently 700 µL of CTAB was added and incubated at 65 °C for 90 min. After cooling, 700 µL of CHCl3 was added and mixed well by upside down shaking. The tubes were spun at 10 000 rpm for 10 min; the aqueous phase was transferred to a fresh tube and added with 900 µL of 100% ethanol. Tubes were again centrifuged and the pellet (DNA) was washed with 70% ethanol.
Amplification of 16S rRNA gene by PCR
The 16S rRNA gene was amplified by using polymerase chain reaction (PCR) from the isolated DNA of cyanobacteria using specific primers CYA106F (5′ CGG ACG GGT GAG TAA CGC GTG A 3′) and CYA781 (R)b (5′ GAC TAC TGG GGT ATC TAA TCC CAT T 3′), as previously described by Nübel et al. (Citation1997). The PCR volumes were kept 30 μL containing 2 μL (≈20 ng) of DNA, 16 µL of PCR mixture 2× Master Mix (Prime), 1 μL of each primer (10 pmolmL−1), and 10 μL of Milli-Q water. The PCR were performed in a My gene thermo cycler (Applied Biosystems, Foster City, CA) as follows: initial denaturation for 4 min at 94 °C, followed by 35 cycles run under the following programme: 1 min at 94 °C for denaturation, 1 min at 50 °C for annealing, 1.3 min at 72 °C for elongation, and final elongation for 7 min at 72 °C.
Phylogenetic analysis of cyanobacterial 16S rRNA gene
PCR products were confirmed after run in 1.2% agarose gel, purified using RPC purification kit (Real Biotech Corporation (RPC), Bangiao City, Taiwan) and sequenced with reverse and forward primers. The 16S rRNA gene sequences were analyzed using the BLAST system (Altschul et al., 1990) (http://www.ncbi.nlm.nih.gov/BLAST/) to identify their closest relatives. The gene sequence was deposited in the public domain GenBank database. Multiple alignments were then created with reference to the selected GenBank sequences using the ClustalW multiple alignment algorithm. Alignment positions, at which one or more sequences had gaps or ambiguities, were omitted from the analysis. The evolutionary history was inferred by the neighbor joining method using the bootstrap method of phylogeny with 1500 replications employing MEGA 5.0 (Tamura et al., Citation2007). All positions contained gaps and missing data were eliminated.
Mass cultivation of cyanobacteria and extract preparation
Marine cyanobacterial strains were cultivated separately in ASN III medium. Cyanobacteria were grown as static cultures at 27 ± 2 °C in conical flasks (1 L × 20) with each flask containing 500 mL of ASN III medium under a light intensity of 36.45 μmol photons m−2 s−1 with a light/dark (14/10 h) cycle (Rippka et al., Citation1979). Late-log phase cells were harvested by centrifugation and samples were repeatedly washed with distilled water to remove salts and the biomass was air dried at 27 ± 2 °C. The cyanobacterial extracts were obtained by homogenization using methanol–chloroform (1:1 v/v) from 10% (w/v) of cyanobacterial dry biomass. The homogenates were taken in flasks stoppered with cotton plugs and kept in a shaking incubator at 25 °C for 24 h. The extract was centrifuged at 10 000 rpm for 10 min to collect the supernatant, which was subsequently evaporated to dryness and stored at −20 °C.
Measurement of in vitro antiplasmodial activity of marine cyanobacterial extracts
The in vitro antiplasmodial activities of marine cyanobacterial extracts were measured against erythrocytic stages of chloroquine (CQ) sensitive (Pf3D7) and CQ resistant (PfINDO) strains of P. falciparum. Parasite strains were cultivated by the method of Trager and Jensen (Citation1976), with minor modifications. Parasite cultures were maintained in fresh O+ human erythrocytes at 4% hematocrit in complete medium (RPMI1640 with 0.2% sodium bicarbonate, 0.5% Albumax, 45 mg L−1 hypoxanthine, and 50 mg L−1 gentamicin) at 37 °C under reduced O2 (gas mixture contains 5% O2, 5% CO2, and 90% N2). Stock solutions of CQ were prepared in water (Milli-Q grade) and marine cyanobacterial extracts were dissolved in dimethyl sulfoxide (DMSO) (25 mg mL−1). All stocks were then diluted with complete culture medium to achieve the required concentrations. In all cases, the final concentration of DMSO was 0.4%, which was found to be nontoxic to the parasite. Extracts were then placed in triplicate wells of 96-well flat-bottom tissue culture grade plates with extract concentrations ranging between 0 and 100 μg mL−1 in a final well volume of 100 μL for primary screening. Chloroquine [100 nM (for CQ sensitive Pf3D7) and 1000 nM (for CQ resistant PfINDO Strain)] was used as a positive control in all experiments. Parasite culture was synchronized at ring stage with 5% sorbitol (Lambros & Vanderberg, Citation1979). Synchronized culture was aliquoted to drug containing 96-well plates at 2% hematocrit and 1% parasitemia. After 48 h of incubation under standard culture conditions, the plates were harvested and read by the SYBR Green I fluorescence-based method (Smilkstein et al., Citation2004) using a 96-well fluorescence plate reader (Victor, Perkin-Elmer, Morrisville, NC), with excitation and emission wavelengths of 497 and 520 nm, respectively. The fluorescence readings were plotted against drug concentration, and a horizontal line was drawn from the 50% value on the growth axis till it intersected with the growth inhibition curve. Then a vertical line was drawn from the point of intersection and projected on the concentration axis to obtain IC50 values as described earlier (Sharma et al., Citation2012).
Measurement of in vitro cytotoxic activity of marine cyanobacterial extracts against mammalian cell line
Mammalian cell line (HeLa) was used to determine cyanobacterial extract toxicity by using the MTT assay for cell viability as described by Mosmann (Citation1983). HeLa cells were cultured in complete RPMI (cRPMI) containing 10% fetal bovine serum, 0.2% sodium bicarbonate, and 50 μg mL−1 gentamicin. Briefly, cells (104 cells/200 μL well) were seeded into 96-well flat-bottom tissue-culture plates in complete culture medium. Extracts (ranging between 0 and 200 μg mL−1) were added after overnight seeding and incubated for 24 h in a humidified atmosphere at 37 °C and 5% CO2. DMSO (final concentration 10%) was added as a positive control. An aliquot of a stock solution of 3-(4,5-dimethylthiazol-2-yl)-2,5-diphenyltetrazolium bromide (MTT) (5 mg mL−1 in 1× phosphate-buffered saline) was added at 20 μL per well and incubated for another 3 h. After the plate was spun at 1500 rpm for 5 min, the supernatant was removed and 100 μL of the stop agent DMSO was added to the well to dissolve the formazan crystals. Formation of formazan, an index of growth, was read at 570 nm by 96-well plate reader (Versa Max, Molecular Devices, Sunnyvale, CA), and IC50 values were determined by analysis of dose–response curves as indicated earlier. The therapeutic index was calculated as IC50 (mammalian cell)/IC50 (Pf 3D7).
Detection of toxic effects of potent antiplasmodial cyanobacterial extracts against host red blood cells
To detect the toxicity effects of cyanobacterial extracts against red blood cells, the invasion, maturation, and growth rate of malarial parasites in cyanobacterial extracts pre-treated versus untreated erythrocytes were quantified microscopically. Briefly, the uninfected human red blood cells were pre-treated with IC100 values of C12, C18, and C22 (40, 100, and 40 µg mL−1, respectively) for 48 h at 37 °C. The treated cells were washed twice with incomplete RPMI and the washed RBCs were used for culture of P. falciparum. In order to find if the extract treated RBCs were affected or remained unaffected by the treatment, it was necessary to obtain a parasite enriched culture to cause infection in the extract treated red blood cells versus untreated control cells. Hence, we decided to separate the late trophozoite stage parasite infected RBCs from ring infected and uninfected RBCs. For this, the culture was centrifuged (1600 rpm, 5 min, 27 °C) and the pellet was used to prepare 20% cell suspension in incomplete RPMI. The resulting cell suspension was then overlaid with an equal volume of Percoll solution (60% v/v in incomplete RPMI) and the sample was centrifuged (2000 rpm, 20 min, 4 °C). The interphase (trophozoite enriched) was aspirated out, carefully pipetted into a microfuge tube and cells harvested by centrifugation (1600 rpm, 5 min). The resulting pellet was washed using incomplete RPMI. The collected sample was adjusted to 1% parasitemia and 2% hematocrit by using cyanobacterial extracts treated or untreated RBC and transferred to 96-well plates (100 µL/well). The plates were incubated at normal culture conditions for 72 h. Thin blood smears were prepared from replicate wells of both control and test samples at 24, 48, and 72 h. Further the Giemsa stained blood smears were analyzed microscopically to check the invasion rate (at 24 h), maturation rate (at 48 h), and growth rate (at 72 h). Approximately 3000 cells were counted for each sample by using Autocount software (Monash University, Clayton, VIC, Australia) (Ma et al., Citation2010) and the percentage of invasion, maturation, and growth were calculated by comparison with untreated control. This method has enabled us to find if the action of extracts is specific to the parasite or is an indirect effect via toxicity to the host red blood cells.
Results
As a result of our survey, 23 filamentous and two unicellular strains of cyanobacteria belonging to Chroococcaceae, Oscillatoriaceae, Nostocaceae, Scytonemataceae, and Rivulariaceae families were primarily identified by microscopic observation. Based on the morphological characters, cyanobacteria were classified under the genera Aphanocapsa, Synechococcus, Anabaena, Calothrix, Lyngbya, Oscillatoria, Phormidium, Spirulina, Geitlerinema, and Pseudoscytonema ( and ).
Figure 2. Micrographs of cyanobacterial isolates at 100×. Twenty-five different strains of cyanobacteria were isolated from various coastal regions of Tamil Nadu. The monocultures of all isolated cyanobacteria were examined under light microscope (Binocular microscope inverted epifluorescence Model XTS, Italy) at the same magnification; the scale bar 50 µm applies to all panels and the genera of cyanobacteria were identified following Desikachari (Citation1959) manual. C12 (Lyngbya aestuarii CNP 1005) and C22 O. boryana BDU 91451) showed promising antiplasmodial activity (IC50 = 18 µg mL−1) against Pf3D7. indicates the names of genera for the strains in the figure.
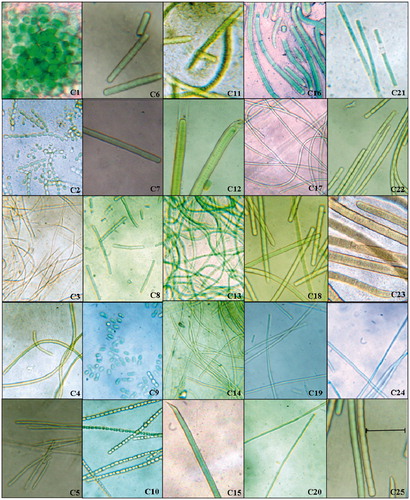
Organic extracts of the above 25 cyanobacteria were screened for their antiplasmodial activity against P. falciparum 3D7 ( and ). The classification of inactive, moderately active, and active is based on antiplasmodial potency shown by different samples. Thus in recent literature, an IC50 value of 41.88 μg mL−1 (Inbaneson & Ravikumar, Citation2011) and IC50 49.63 μg mL−1 (Ravikumar et al., Citation2011a) have been classified as moderately active while IC50 5.06 and 16.69 μg mL−1 (Ravikumar et al., Citation2011b) and IC50 6 and 26 μg mL−1 (McPhail et al., Citation2007) have been classified as promising or good. In our study, we have justifiably classified IC50 of 18 μg mL−1 {L. aestuarii CNP 1005 (C12), O. boryana BDU 91451 (C22)} as active. Anabaena constricta CNP 5007 (C10), O. boryana BDU 141071 (C18), Aphanocapsa littoralis (CNP 2022), O. salina (BDU 141792), Spirulina subsalsa (CNP 1020), Anabaena spp. (CNP 2061 and 5007), and Phormidium spp. (CNP 1009, 2012, and 7010) which exhibited IC50 of 35 μg mL−1–100 μg mL−1 were considered as moderately active while all other extracts with IC50 >100 μg mL−1 were considered as inactive. Furthermore, the extracts of L. aestuarii (CNP 1005) and O. boryana (BDU 91451) showed promising resistance indices (1.9–3.4) against CQ resistance PfINDO strain (). The cytotoxicity results revealed that the potent antiplasmodial extracts of L. aestuarii CNP 1005 () and O. boryana BDU 91451 were relatively nontoxic to mammalian cell line HeLa (IC50 > 200 μg mL−1), with selectivity index of >11.1.
Figure 3. Antiplasmodial activity of extracts of the marine cyanobacterial species. Concentration-dependent inhibition of blood-stage Plasmodium falciparum growth by cyanobacterial crude extracts as measured by SYBR Green fluorescence assay. Extract numbers and the corresponding color codes are indicated in the strip on the right. Standard deviation bars at each data point have been calculated from triplicate observations.
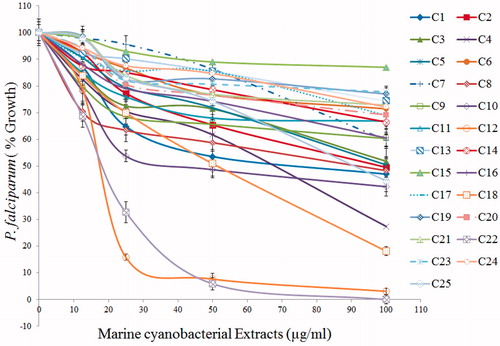
Table 3. Antiplasmodial potency, resistance and selectivity indices of potent cyanobacterial extracts.
The possible toxicity of the three cyanobacterial extracts (C12, C18, and C22) against host red blood cells was analyzed by microscopic comparison of invasion, maturation, and growth rate of malarial parasites in IC100 pre-treated versus untreated RBCs. No hemolysis was observed in treated RBCs. Moreover, the C12 and C22 pre-treated RBCs failed to show any significant reduction of invasion, maturation, and growth rate of malaria parasites in comparison with untreated RBC controls while the C18 treated RBCs showed slight reduction in invasion (17%), maturation (17%), and growth (21%) of malarial parasites (). These results suggest that the C12 and the C22 cyanobacterial extracts were not toxic to host RBCs and they inhibited the growth of malaria parasites through direct action against P. falciparum.
Figure 4. Pretreatment of host red blood cells with antiplasmodial extracts to find if their action is against host red blood cells. The aim of the experiment was to judge if the extracts are selectively toxic to the malaria parasite or that they are toxic to host red blood cells leading to indirect antiplasmodial toxicity. Uninfected human red blood cells were incubated (48 h, 37 °C) with extracts of C12, C18, and C22 each at its antiplasmodial IC100. Results shown above indicate that there was no significant effect of pre-exposure on invasion, maturation, or growth of the malaria parasite suggesting the selective action of extracts on Plasmodium falciparum with no significant toxicity against host red blood cells.
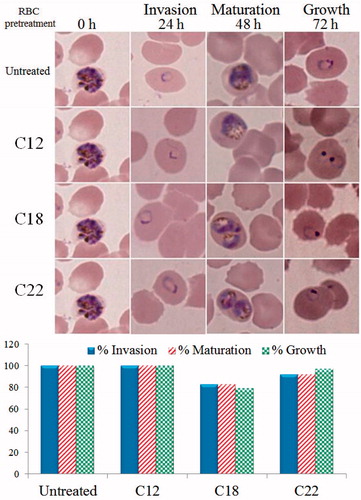
Microscopic identification of certain cyanobacterial genera including Lyngbya, Oscillatoria, Phormidium, Nostoc, and Anabaena is difficult. Hence, identification through 16S rRNA gene sequencing was resorted to find out the genus and species for C12 which was found to exhibit the most promising antiplasmodial activity. The phylogenetic analysis () identified C12 as L. aestuarii (CNP 1005) (). The PCR amplification of the 16S sequence from L. aestuarii (CNP 1005) () gave 591 bp of DNA (), which was blasted using BLAST system. The DNA obtained from this cyanobacterium showed sequence similarity with the DNA of another cyanobacterium (accession number AJ621838) and the sequence was deposited in the GenBank database (accession number JX519572).
Figure 5. Morphology and molecular identification of Lyngbya aestuarii CNP 1005, the strain showing the most potent antiplasmodial action in the present studies. (A) Microscopic image of L. aestuarii CNP 1005, (B) amplification of L. aestuarii 16S rRNA, (C) amplified 16S rRNA sequence using forward primer, and (D) phylogenetic tree.
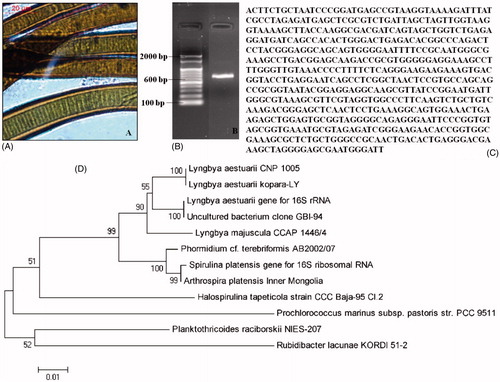
Discussion
Marine cyanobacteria are promising sources for potent antimalarial compounds as well as other pharmacological properties. Some representative secondary metabolites of marine cyanobacterial organisms and their biological activities including antimalarial activities have been listed in and . Their widespread availability and vast biodiversity of species offers the possibility of identifying metabolites occupying rich chemical diversity space. The ability to grow marine cyanobacterial organisms under laboratory conditions ensures consistency of results, not influenced by variations arising from collections made from different places and at different times. Furthermore, the in vitro grown cultures can be scaled up to obtain sufficient biomass for harvesting of promising lead compounds. Previous reports have indicated potent antiprotozoal activities of several marine cyanobacteria. For instance, viridamide A, an alkynoic lipopeptide, isolated from Oscillatoria nigro-viridis showed impressive activities against P. falciparum (IC50 = 5.8 μM), Leishmania donovani (IC50 = 1.37 μM), and Trypanosoma cruzi (IC50 = 1.0 μM) (Simmons et al., Citation2008).
Table 4. Biological activities of compounds produced by marine cyanobacteria.
Linington et al. (Citation2007) isolated two active antimalarial peptides named venturamides A and B (IC50 = 8.2 and 5.6 μM, respectively) from another Oscillatoria sp. from coastal regions of Panama. Likewise, crude organic extracts of Lyngbya majuscula from different sites of coastal regions of Panama exhibited promising antiplasmodial activities (IC50 = 6 and 26 μg mL−1) against CQ-resistant P. falciparum (McPhail et al., Citation2007). It is interesting that, in our study, the organic extract of in vitro grown L. aestuarii CNP 1005 has shown promising antiplasmodial activity against both CQ-sensitive (IC50 = 18 μg mL−1) and resistant strains (IC50 = 34 μg mL−1) of P. falciparum. McPhail et al. (Citation2007) have previously reported the occurrence of carmabin A, dragomabin, and dragonamide A in Lyngbya majuscula. The antiplasmodial IC50s of these molecules against CQ-resistant P. falciparum W2 strain were reported to be 1.4, 21.0, and 10.7 μM, respectively, while their cytotoxicity IC50 against Vero cell line were 9.8, 182.3, and 67.8 μM, respectively (McPhail et al., Citation2007). Another alkynoic lipopeptide, jamaicamide B, isolated from L. majuscula collected from Hector's Bay, Jamaica, exhibited antimalarial activity (IC50 = 18.4 μM) along with high cytotoxicity (IC50 = 16.2 μM) against Vero cells (Edwards et al., Citation2004).
The antimalarial activities of cyclodepsipeptides of Lyngbya majuscula origin namely lagunamide A (IC50 0.19 μM), lagunamide B (IC50 = 0.91 μM) (Tripathi et al., Citation2010a), and malyngolide dimer (Gutiérrez et al., Citation2010) (IC50 19 μM) against P. falciparum appear to reinforce the possibilities of finding new antimalarial pharmacophores from L. aestuarii CNP 1005 (IC50 = 18 μg mL−1) isolated from coastal regions of Tamil Nadu. To the best of our knowledge, there are no antimalarial studies from the heterocystous Anabaena sp. while, in this study, Anabaena constricta CNP 5007 from coastal region of Tamil Nadu has been found to inhibit CQ-sensitive Pf3D7 (IC50 = 35 μg mL−1). The nontoxic effects of Anabaena constricta CNP 5007 against HeLa cell line (IC50 = 200 μg mL−1) indicates the promise of isolation of safe antimalarials from this source. Although strong antimalarial activities of calothrixin A and calothrixin B (IC50 58 and 180 nM, respectively) (Rickards et al., Citation1999) from Calothrix sp. have been reported, in the present study, Calothrix brevissima CNP 2000 (IC50 > 100 μg mL−1) failed to inhibit the growth of malaria parasite. In view of potent antimalarial activities of hierridin B (IC50 = 5.2 μg mL−1) from P. ectocarpi (Papendorf et al., Citation1998), the exploration of antiplasmodial activity in extracts of Phormidium sp. from coastal regions of Tamil Nadu assumes great significance. It is interesting to note that three strains of Phormidium sp. (CNP 7010, CNP 1009, and CNP 2012) exhibited moderate antiplasmodial activity (IC50 = 93–100 μg mL−1) against P. falciparum. Furthermore, the moderate antiplasmodial activities of Aphanocapsa littoralis CNP 2022 (IC50 = 74 μg mL−1) and Spirulina subsalsa CNP 1020 (IC50 = 68 μg mL−1) also appear to hold promise for the possibilities of isolating potent antimalarial compounds.
In view of the fact that marine cyanobacterial organisms are well known for producing toxins, it was important to find if the antiplasmodial activity observed by us was specifically against the parasite or due to host cell toxicity. Hence, we tested the toxicity of extracts against HeLa cells, on one hand, and on extract treated and washed red blood cells, on the other hand. Both these assays indicated that the extracts were selective in their action against the parasite.
Conclusion
The present studies have revealed the possibilities of new antimalarial compounds from marine cyanobacteria. The preliminary antiplasmodial screening of different strains of marine cyanobacteria obtained from coastal regions of Tamil Nadu showed promising antiplasmodial activities against P. falciparum with good therapeutic indices. L. aestuarii CNP 1005 and O. boryana BDU 91451 showed strong antiplasmodial activity against P. falciparum 3D7 (IC50 = 18 μg mL−1) with good resistance indices of 1.9 and 3.4, respectively. These two extracts were selective in their action against the parasite. Our ability to grow both these cyanobacterial species in the laboratory augurs well for the isolation of active compounds in substantial amounts.
Declaration of interest
We are grateful to the funding agency, Defence Research and Development Organization (Sanction no. DLS/81/48222/LSRB-144/BTB/2008) for the financial support to carry out this research work. We thank the Indian Council of Medical Research, New Delhi for fellowship to M. Dinesh.
Acknowledgements
We thank X. Su and the MR4 for providing chloroquine-resistant INDO strain of P. falciparum.
References
- Altschul SF, Gish W, Miller W, et al. (1990). Basic local alignment search tool. J Mol Biol 215:403–10
- Balunas MJ, Linington RG, Tidgewell K, et al. (2010). Dragonamide E, a modified linear lipopeptide from Lyngbya majuscula with antileishmanial activity. J Nat Prod 73:60–6
- Burrows JN, Chibale K, Wells TN. (2011). The state of the art in antimalarial drug discovery and development. Curr Top Med Chem 11:1226–54
- Chang TT, More SV, Lu I, et al. (2011). Isomalyngamide A, A-1 and their analogs suppress cancer cell migration in vitro. Eur J Med Chem 46:3810–19
- Clark BR, Engene N, Teasdale ME, et al. (2008). Natural products chemistry and taxonomy of the marine cyanobacterium Blennothrix cantharidosmum. J Nat Prod 71:1530–7
- Costa M, Costa-Rodrigues J, Fernandes MH, et al. (2012). Marine cyanobacteria compounds with anticancer properties: A review on the implication of apoptosis. Mar Drugs 10:2181–207
- Desikachari TV. (1959). Cyanophyta. ICAR Monographs on Algae. New Delhi: Indian Council of Agricultural Research
- Doyle JJ, Doyle JL. (1987). A rapid DNA isolation procedure for small quantities of fresh leaf tissue. Phytochem Bull 19:11–5
- Dondorp AM, Nosten F, Yi P, et al. (2000). Artemisinin resistance in Plasmodium falciparum malaria. N Engl J Med 361:455–67
- Edwards DJ, Marquez BL, Nogle LM, et al. (2004). Structure and biosynthesis of the jamaicamides, new mixed polyketide peptide neurotoxins from the marine cyanobacterium Lyngbya majuscula. Chem Biol 11:817–33
- Fattorusso E, Taglialatela-Scafati O. (2009). Marine antimalarials. Mar Drugs 7:130–52
- Froscio SM, Humpage AR, Burcham PC, Falconer IR. (2003). Cylindrospermopsin induced protein synthesis inhibition and its dissociation from acute toxicity in mouse hepatocytes. Environ Toxicol 18:243–51
- Gunasekera SP, Owle CS, Montaser R, et al. (2011). Malyngamide 3 and cocosamides A and B from the marine cyanobacterium Lyngbya majuscula from Cocos Lagoon, Guam. J Nat Prod 74:871–6
- Gutiérrez M, Tidgewell K, Capson TL, et al. (2010). Malyngolide dimer, a bioactive symmetric cyclodepside from the panamanian marine cyanobacterium, Lyngbya majuscula. J Nat Prod 73:709–11
- Inbaneson SJ, Ravikumar S. (2011). In vitro antiplasmodial activity of marine sponge Hyattella intestinalis associated bacteria against Plasmodium falciparum. Asian Pac J Trop Biomed 1:S100–4
- Iwasaki A, Teruya T, Suenaga K. (2010). Isolation and structure of koshikalide, a 14-membered macrolide from the marine cyanobacterium Lyngbya sp. Tetrahedron Lett 51:959–60
- Lambros C, Vanderberg JP. (1979). Synchronization of Plasmodium falciparum erythrocytic stages in culture. J Parasitol 65:418–20
- Linington RG, González J, Urena L, et al. (2007). Venturamides A and B: Antimalarial constituents of the panamanian marine cyanobacterium Oscillatoria sp. J Nat Prod 70:397–401
- Linington RG, Clark BR, Trimble EE, et al. (2009). Antimalarial peptides from marine cyanobacteria: Isolation and structural elucidation of gallinamide A. J Nat Prod 72:14–7
- Ma C, Harrison P, Wang L, Coppel RL. (2010). Automated estimation of parasitaemia of Plasmodium yoelii infected mice by digital image analysis of Giemsa-stained thin blood smears. Malar J 9:1–9
- Malloy KL, Villa FA, Engene N, et al. (2011). Malyngamide2, an oxidized lipopeptide with nitric oxide inhibiting activity from a Papua New Guinea marine cyanobacterium. J Nat Prod 74:95–8
- Maruthanayagam V, Nagarajan M, Sundararaman M. (2013). Cytotoxicity assessment of cultivable marine cyanobacterial extracts in Artemia salina (brine shrimp) larvae and cancer cell lines. Toxin Rev 32:1–9
- McPhail KL, Correa J, Linington RG, et al. (2007). Antimalarial linear lipopeptides from a panamanian strain of the marine cyanobacterium, Lyngbya majuscula. J Nat Prod 70:984–8
- Montaser R, Abboud K, Paul VJ, Luesch H. (2011). Pitiprolamide, a proline-rich dolastatin16 analogue from the marine cyanobacterium Lyngbya majuscula from Guam. J Nat Prod 74:109–12
- Mosmann T. (1983). Rapid colorimetric assay for cellular growth and survival: Application to proliferation and cytotoxicity assays. J Immunol Methods 65:55–63
- Nagarajan M, Maruthanayagam V, Sundararaman M. (2012). A review of pharmacological and toxicological potentials of marine cyanobacterial metabolites. J Appl Toxicol 32:153–85
- Newman DJ, Cragg GM. (2007). Natural products as sources of new drugs over the last 25 years. J Nat Prod 70:461–7
- Noedl H, Se Y, Socheat D, Fukuda MM. (2008). Evidence of artemisinin-resistant malaria in Western Cambodia. N Engl J Med 359:2619–20
- Nübel U, Garcia-Pichel F, Muyzer G. (1997). PCR primers to amplify 16S rRNA genes from cyanobacteria. Appl Environ Microbiol 63:3327–32
- Nunnery JK, Mevers E, Gerwick WH. (2010). Biologically active secondary metabolites from marine cyanobacteria. Curr Opin Biotechnol 21:787–93
- Papendorf O, Koenic GM, Wright AD. (1998). Hierridin b and 2,4-dimethoxy-6-heptadecyl-phenol, secondary metabolites from the cyanobacterium Phormidium ectocarpi with antimalarial activity. Phytochemistry 49:2383–6
- Ravikumar S, Inbaneson SJ, Suganthi P, et al. (2011a). Mangrove plants as a source of lead compounds for the development of new antiplasmodial drugs from South East coast of India. Parasitol Res 108:1405–10
- Ravikumar S, Inbaneson SJ, Suganthi P, et al. (2011b). In vitro antiplasmodial activity of ethanolic extracts of seaweed macroalgae against Plasmodium falciparum. Parasitol Res 108:1411–6
- Rickards RW, Rothschild JM, Willis AC, et al. (1999). Calothrixins A and B, novel pentacyclic metabolites from Calothrix cyanobacteria with potent activity against malaria parasites and human cancer cells. Tetrahedron 55:13513–20
- Rippka R, Deruelles J, Waterbury JB, et al. (1979). Generic assignments, strain histories and properties of pure cultures of cyanobacteria. J Gen Microbiol 111:1–61
- Simmons TL, Engene N, Urena LD, et al. (2008). Viridamides A and B, lipodepsipeptides with antiprotozoal activity from the marine cyanobacterium, Oscillatoria nigro-viridis. J Nat Prod 71:1544–50
- Sharma N, Mohanakrishnan D, Shard A, et al. (2012). Stilbene-chalcone hybrids: Design, synthesis, and evaluation as a new class of antimalarial scaffolds that trigger cell death through stage specific apoptosis. J Med Chem 12:297–311
- Smilkstein M, Sriwilaijaro N, Kelly JX, et al. (2004). Simple and inexpensive fluorescence-based technique for high-throughput antimalarial drug screening. Antimicrob Agents Chemother 48:1803–6
- Stolze SC, Deu E, Kaschani F, et al. (2012). The antimalarial natural product symplostatin 4 is a nanomolar inhibitor of the food vacuole falcipains. Chem Biol 19:1546–55
- Tamura K, Dudley J, Nei M, Kumar S. (2007). MEGA4: Molecular Evolutionary Genetics Analysis (MEGA) software version 4.0. Mol Biol Evol 24:1596–9
- Tan LT. (2007). Bioactive natural products from marine cyanobacteria for drug discovery. Phytochemistry 68:954–79
- Thornburg CC, Thimmaiah M, Shaala LA, et al. (2011). Cyclic depsipeptides, grassypeptolides D and E and ibuepidemethoxylyngbyastatin 3, from a Red Sea Leptolyngbya cyanobacterium. J Nat Prod 74:1677–85
- Trager W, Jensen JB. (1976). Human malaria parasites in continuous culture. Science 193:673–5
- Tripathi A, Puddick J, Prinsep MR, et al. (2010a). Lagunamides A and B: Cytotoxic and antimalarial cyclodepsipeptides from the marine cyanobacterium, Lyngbya majuscula. J Nat Prod 73:1810–4
- Tripathi A, Puddick J, Prinsep MR, et al. (2010b). Hantupeptins B and C, cytotoxic cyclodepsipeptides from the marine cyanobacterium Lyngbya majuscula. Phytochemistry 71:307–11
- Tripathi A, Puddick J, Prinsep MR, et al. (2011). Lagunamide C, a cytotoxic cyclodepsipeptide from the marine cyanobacterium, Lyngbya majuscula. Phytochemistry 72:2369–75
- Vincent WF. (2009). Cyanobacteria. In: Likens GE, ed. Encyclopedia of Inland Water. Vol. 3. Oxford: Elsevier, 226–32
- White NJ. (2010). Artemisinin resistance: The clock is ticking. Lancet 376:2051–2
- World Malaria Report (2011) World Health Organization. Available from: http://www.who.int/malaria/publications/atoz/9789241564403/en/index.html [last accessed 6 Dec 2013]
- Yadav S, Sinha RP, Tyagi MB, Kumar A. (2011). Cyanobacterial secondary metabolites. Int J Pharm Bio Sci 2:144–67