Abstract
Context: Scilla scilloides Druce (Liliaceae) is a folk medicine to treat dermal inflammation; however, the medicinal properties of this plant have not been completely established.
Objective: The current study investigates the potent anti-inflammatory effects of S. scilloides bulbs for its traditional usage using lipoxygenase and hyaluronidase as the inflammation model. To gain insight into the active constituents, nine homoisoflavones (1–9) were subsequently tested.
Materials and methods: Lipoxygenase and hyaluronidase inhibition of ethyl acetate extract from the bulbs of this plant within 2000 µg/mL or homoisoflavones within 1000 µM were determined by colorimetric methods. RAW264.7 cells were incubated with 10 or 50 µM homoisoflavones plus lipopolysaccharide (LPS) for 24 h. The culture media were collected and analyzed for determination of the nitric oxide (NO) level by the colorimetric Griess method to measure the extent of inflammation.
Results: The extract exhibited inhibitory effects on lipoxygenase and hyaluronidase activities with IC50 values 31.5 and 169 µg/mL, respectively. Among the nine homoisoflavones tested, four (1 and 3–5) resulted in 79.3–97.9% higher lipoxygenase inhibition than 6.7–32.7% of the others at 500 µM. Calculated IC50 values indicated 5 as the compound responsible for strong lipoxygenase inhibition with 15.8 µM as the IC50 value. In the hyaluronidase assay, all homoisoflavones tested at 1000 µM demonstrated 16.2–58.0% inhibition. Incubating the cells in the presence of all nine homoisoflavones tested at 50 µM significantly suppressed the NO production, downward to 1.5–66.0%, in the LPS-activated macrophage cells as a model.
Discussion and conclusion: These results may indicate a potential role of S. scilloides for anti-inflammatory purposes.
Introduction
Scilla scilloides Druce (Liliaceae) is a perennial herb native to Far Eastern Asia, including the Ussuri basin, Manchuria, Korea, Japan, Ryukyu, Taiwan, and China (Araki, Citation1985; Noda, Citation1976). The bulb part of this plant is garlic-like oval shape and it has been historically used as a foodstuff (Maekawa, Citation1944; Yamaguchi, Citation1959) and in traditional folk medicine as an antidote, blood circulatory activator, and treatment of dermal inflammation such as abscess and dermatitis combustionis (Namba, Citation1993). Recent study further discloses the antimicrobial activity of S. scilloides (Yeo et al., Citation2006) and anticancer activity of the Scilla plants, e.g., S. scilloides and S. peruviana L. (Lee et al., Citation2002; Mikami et al., Citation1994). We have previously separated and isolated nine homoisoflavones and seven other compounds from S. scilloides (Nishida et al., Citation2008). However, the mechanisms underlying the anti-inflammatory properties of the bulbs of S. scilloides and its possible active constituent(s) remain to be completely established.
Inflammation is a response of humans and other mammals to the presence of pathogens and chemical or mechanical injury. In inflammatory responses, several inflammation-related enzymes are known to be involved in generating inflammatory mediators or enhancing tissue damage. Lipoxygenase is a family of key enzymes that produce inflammatory leukotrienes, lipoxins, and hydroxyeicosatetraenoic acids in a number of pathophysiological processes such as asthma, psoriasis, cancer metastasis, and atherosclerosis (Henderson, Citation1994; Lewis et al., Citation1990; Samuelsson, Citation1983). Due to the involvement of this enzyme in various inflammation-related processes, there is a considerable interest in examining whether natural products can inhibit the lipoxygenase pathway in arachidonic cascade (Gleason et al., Citation1986; Ling et al., Citation2003; Komoda et al., Citation2004).
Hyaluronidase is known to be a family of key enzymes involved in human tissue remodeling during allergy and other inflammatory responses (Guerra, Citation1946; Horton et al., Citation1998; Kakegawa, Citation1988; Mio & Stern, Citation2002). Previous reports show that the action of activated hyaluronidase can be involved in the allergic inflammation in line with the histamine release from mast cells (Asada et al., Citation1997; Sawabe et al., Citation1992). Hyaluronidase inhibitory activity has been, therefore, considered a model for screening anti-inflammatory materials and/or compounds from natural sources (Asada et al., Citation1997; Kakegawa et al., Citation1988; Sawabe et al., Citation1992).
In this study, we report the potential role of an extract from the bulbs of S. scilloides on the lipoxygenase and hyaluronidase activities to investigate the mechanisms of this traditional herbal medicine. These two enzymes were used as valid and useful models for the discovery of anti-inflammatory and chemotherapeutic agents. Nine homoisoflavones previously isolated from this plant were further evaluated in both assays. To gain insight into the physiological relevance of homoisoflavones with their potent anti-inflammatory effect, activated mouse macrophage-like cells were incubated in the presence of these compounds.
Materials and methods
Materials
The bulbs of S. scilloides used in this study were originally cultivated by a farmer contracted with Aso Pharmaceutical Co. Ltd. (Kumamoto, Japan), and harvested in August 2005 in Kumamoto Prefecture, Japan. The obtained plant was subsequently identified by Professor Dr. Toshihiro Nohara (Faculty of Pharmaceutical Sciences, Sojo University, Kumamoto Prefecture, Japan) where the voucher specimen (SCK2005) was deposited at the laboratory of Natural Products Chemistry, School of Agriculture, Tokai University, Kumamoto, Japan. This batch (SCK2005, same as the identification reference) has been used for chemical analyses (Nishida et al., Citation2008; Ono et al., Citation2011, Citation2012, Citation2013) and further bioassays. Lipoxygenase type I-B, nordihydroguaiaretic acid (NDGA), hyaluronidase from bovine testis type IV-S, compound 48/80, and lipopolysaccharide (LPS) from Escherichia coli were purchased from Sigma-Aldrich Co. (St. Louis, MO). Linoleic acid, sodium hydroxide (NaOH), methyleneblue trihydrate, hyaluronic acid potassium salt from human umbilical cord, hydrochloric acid (HCl), p-dimethylaminobenzaldehyde (p-DAB), and tannic acid, were products from Nacalai Tesque, Inc. (Kyoto, Japan). All other chemicals were of the highest grade commercially available. RAW 264.7 mouse macrophage-like cells (ECACC91062702, from DS Pharma Biomedical Co. Ltd., Osaka, Japan) were routinely maintained in DMEM culture medium supplemented with 5% fetal bovine serum.
Preparation of the plant extract and isolation of homoisoflavones
Using a same batch of S. scilloides (SCK2005), we have previously separated and isolated nine homoisoflavones and other compounds from the bulbs of this plant by methanol (MeOH) extraction (Nishida et al., Citation2008; Ono et al., Citation2011, Citation2012, Citation2013). All the chemicals previously isolated and nine homoisoflavones (1–9) used in this study are compiled in . Briefly, the crushed fresh bulbs of S. scilloides (18.5 kg) were soaked in MeOH at room temperature for extraction as described previously (Nishida et al., Citation2008). The MeOH extract, obtained as a syrup paste following vacuum evaporation, was subsequently divided into H2O fraction and ethyl acetate (EtOAc) fraction. After the vacuum evaporation, the yield of the EtOAc fraction obtained was 26.4 g and used as the S. scilloides extract for following bioassays. To obtain nine pure homoisoflavones (1–9), the EtOAc extract was successively subjected to silica gel, Chromatorex ODS, and Sephadex LH-20 column chromatography (Amersham Pharmacia Biotech AB, Uppsala, Sweden) as well as further HPLC on ODS and silica gel. Based on their physical and spectral data, compounds 1–9 were confirmed as this plant-specific homoisoflavones. Their chemical structures are shown in .
Table 1. List of the chemicals previously isolated by the authors and used in this studya.
Lipoxygenase inhibition
The lipoxygenase assay was performed based on the decolorization of methyleneblue during the enzymatic reaction (Anthon & Barrett, Citation2003; Suda et al., Citation1995). Linoleic acid solution as a 50 mM stock was first prepared in a volume of 1.0 mL, containing linoleic acid (56 mg), Tween 20 (112 mg), 1 N NaOH (24 µL), and water (811.2 µL), and stored at −20 °C under N2 gas. Lipoxygenase was prepared in 0.1 M phosphate buffer (pH 6.0). The standard assay mixture, in a final volume of 350 µL, contained the sample solution (17.5 µL) at varying concentrations, 0.2 M phosphate buffer (pH 6.0) (140 µL), 25 mM linoleic acid solution (5.6 µL), 250 µM methyleneblue (14 µL), water (102.9 µL), and 212 kU/mL lipoxygenase (70 µL). The reaction was started by the addition of the enzyme, allowed to proceed for 0 min and 30 min at room temperature in a 96-well multiplate. The assay mixture was used for a colorimetric measurement at 660 nm using a grating microplate reader (SH-1000Lab, Corona Electric, Ibaraki, Japan). The lipoxygenase inhibitory activity was expressed as the percentage inhibition:
Hyaluronidase inhibition
The hyaluronidase assay was performed using hyaluronic acid as the substrate. Based on the detection of liberated N-acetyl glucosamine end group, the enzymatic activity as well as inhibition rate was determined by the Morgan–Elson method (Reissig et al., Citation1955) as modified (Aronson & Davidson, Citation1967; Kakegawa et al., Citation1985). Hyaluronidase, compound 48/80, and hyaluronic acid potassium salt were first prepared in 0.1 M acetate buffer (pH 4.0). The standard assay mixture, in a final volume of 30 µL, contained the sample solution (5 µL) at varying concentrations, 0.1 M acetate buffer (pH 4.0) (15 µL), and 2 kU/mL hyaluronidase (10 µL). After a pre-incubation for 20 min at 37 °C, 1 mg/mL compound 48/80 solution (20 µL) was added to activate the enzyme and given another incubation for 20 min. The reaction was started by the addition of 0.8 mg/mL hyaluronic acid (50 µL), allowed to proceed for 40 min at 37 °C, terminated by adding 0.4 N NaOH solution (20 µL) and kept on ice. Sodium borate buffer (pH 9.1) (20 µL) was added into the assay mixture, heated for 3 min at 100 °C and cooled down on ice. p-DAB reagent (600 µL) was subsequently added and incubated for 20 min at 37 °C. p-DAB reagent was prepared as follows: 5 g of p-DAB dissolved in 43.75 mL of glacial acetic acid and 6.25 mL of 10 N HCl was stored at 4 °C as 10× stock solution, and diluted with acetic acid before use. After a brief centrifugation, the supernatant (350 µL) was allowed to a colorimetric measurement at 585 nm using a microplate reader. The hyaluronidase inhibitory activity was expressed as the percentage inhibition:
Nitric oxide (NO) generation assay
For the NO generation assay, RAW264.7 cells seeded in individual wells of a 96-well culture plate at a density of 0.5 × 105 cells in 100 µL per well were preincubated for 3 h, and thereafter incubated with another culture medium (25 µL) containing nine individual homoisoflavones at 10 or 50 µM in the presence of 100 ng/mL LPS. After incubation for 24 h, the culture medium centrifuged was collected and the level of NO therein was measured by the Griess method as described (Marcocci et al., Citation1994; Wang et al., Citation2008). Briefly, the collected culture medium (100 µL) was mixed with the same amount of the Griess reagent in a new 96-well microplate. The level of NO generated therein was determined colorimetrically at 550 nm using a microplate reader.
Statistics
For statistical analysis, the values are expressed as mean ± standard deviation derived from three or four parallel experiments. Data in part were analyzed using statistical add-on software program (Statcel, OMS Co., Saitama, Japan) for Excel 2004 (Microsoft Corp., Redmond, WA). Statistical differences were considered significant at p < 0.001 using Student’s t-test.
Results and discussion
The bulbs of S. scilloides are used as a folk medicine to treat dermal inflammation and skin disorders (Namba, Citation1993). However, the potent anti-inflammatory mechanisms remain to be entirely established. The current study investigates the inhibitory effects of the extract and nine homoisoflavones from S. scilloides in lipoxygenase and hyaluronidase assays.
Lipoxygenase and hyaluronidase inhibitions by S. scilloides extract
We first attempted to investigate whether the extract of the bulbs of S. scilloides can inhibit lipoxygenase activity. As shown in , S. scilloides extract demonstrated concentration-dependent inhibition in the enzymatic assay. Upon this experimental setting, a negative control of S. scilloides extract and NDGA showed 0.0 ± 6.1% and 0.0 ± 4.7% of variances, respectively (data not shown). The calculated half-maximal inhibitory concentration (IC50) of S. scilloides extract was 31.5 µg/mL. The standard sample, NDGA, provided an IC50 value of 3.26 µg/mL. Thus, S. scilloides possesses at least 0.10-fold extent of inhibition capability compared with NDGA, and its active constituent(s) present may contribute to the suppression of lipoxygenase-related inflammation. It is to note that the obtained IC50 of NDGA is comparable with another report with IC50 5.47 µg/mL (Swarnalatha & Satyanarayana, Citation2011). Previous reports have indicated that inhibitors of lipoxygenase can play a suppressive role in acute dermal inflammation (Yamazaki et al., Citation1998) and histamine-induced extravasation in vivo (Giannaras et al., Citation2005).
Figure 2. Effect of concentration of S. scilloides extract in lipoxygenase (A) and hyaluronidase assays (B). Data shown represent mean ± S.D. from four experiments. NDGA in (A) and tannic acid in (B) were used as the standard sample.
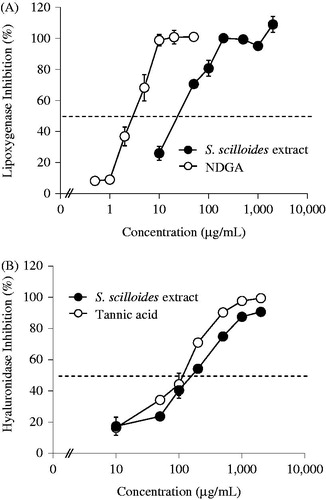
We next investigated whether the bulbs of S. scilloides can inhibit hyaluronidase activity. As shown in , S. scilloides extract also demonstrated a concentration-dependent inhibition in hyaluronidase assay. Upon this experimental setting, a negative control of S. scilloides extract and tannic acid showed 0.0 ± 4.7% and 0.0 ± 2.6% of variances, respectively (data not shown). The calculated IC50 value of S. scilloides extract was 169 µg/mL. The standard sample, tannic acid, provided an IC50 value of 121 µg/mL. Thus, S. scilloides possesses at least 0.72-fold extent of inhibition capability compared with tannic acid, and active constituent(s) present may contribute to the suppression of hyaluronidase-related inflammation. It is to note that the IC50 of tannic acid obtained here is comparable with another report with an IC50 of 129 µg/mL (76.1 µM) (Girish & Kemparaju, Citation2005). Previous studies have reported that patients with burns, septicemia, or shock have rapid increases in circulating hyaluronic acid levels because of the activation of hyaluronidase (Engstrom-Laurent & Hellstrom, Citation1990; Ferrara et al., Citation1991; Onarheim et al., Citation1991a,b). The hyaluronidase inhibition has been demonstrated in other anti-inflammatory drugs such as salicylates (Guerra, Citation1946) and tranilast (Kakegawa et al., Citation1985). S. scilloides may contribute to the suppression of hyaluronidase-related dermal burns and possibly other inflammation-related disorders.
Lipoxygenase and hyaluronidase inhibition by homoisoflavones
As shown in , we have previously isolated and identified nine homoisoflavones and 23 other compounds from the bulbs of S. scillides. Among them, homoisoflavones were first focused on the evaluation in various biological tests for medicinal use of this plant and found to be the antioxidants responsible for 1,1-diphenyl-2-picrylhydrazyl radical scavenging assay (Nishida et al., Citation2013). In view of the anti-inflammatory activity, seven homoisoflavones isolated from the roots and heartwood of Caesalpinia sappan possesses anti-allergic activities upon β-hexosaminidase inhibition system (Yodsaoue et al., Citation2009). To gain insight into the determination of active compounds present in S. scilloides, the extract was further purified by silica gel, Chromatorex ODS, and Sephadex LH-20 column chromatography as well as HPLC on ODS and silica gel to yield nine pure homoisoflavones. Their chemical structures were confirmed by spectroscopic methods according to our previous report (Nishida et al., Citation2008). Nine homoisoflavones were examined in the lipoxygenase assay to evaluate their potent inhibitory effect. A pilot study first revealed four compounds (1 and 3–5) tested at 500 µM showed 79.3–97.7% of high lipoxygenase inhibition while five other compounds (2 and 6–9) resulted in 6.7–32.7% of low inhibition (). On the ranging from 500 to 1000 µM tested, the IC50 values of four homoisoflavones (1 and 3–5) and three (2, 6, and 8) estimated to be out of the range, less than 500 µM and more than 1000 µM, respectively. The IC50 values of 7 and 9 were 927 and 788 µM, respectively. A tetracyclic structure located between B- and C-rings found in 6–9 implies their poor contribution to the structure requirement for the enzyme inhibition. The calculated IC50 value of NDGA was confirmed to be 10.8 µM (3.26 µg/mL) (see ). For comparison with the standard sample, it is, therefore, needed to further determine the IC50 values of four homoisoflavones (1 and 3–5) at lower concentration ranges.
Table 2. Inhibitory effects of nine homoisoflavones (1–9) from S. scilloides in lipoxygenase and hyaluronidase assaysa.
In the case of the hyaluronidase assay, a pilot study demonstrated 16.2–58.0% inhibition when all the compounds were assayed at 1000 µM (). On the ranging from 500 to 1000 µM tested, the IC50 values of seven homoisoflavones (2–4 and 6–9) estimated to be more than 1000 µM, while those of 1 and 5 were 748 and 887 µM, respectively. The calculated IC50 value of tannic acid was confirmed to be 71.1 µM (). Compared with tannic acid, two homoisoflavones (1 and 5) appeared to possess at least 0.08 to 0.10-fold extent of inhibition capability, and other active constituent(s) present in this plant may also contribute to the suppression of hyaluronidase-related inflammation. As shown in , we have previously isolated and identified nine homoisoflavones and 23 other compounds from this plant. It is of note that a pilot study demonstrates three norterpenes possess weak lipoxygenase inhibition and almost no hyaluronidase inhibition activities on the same experimental setting (data not shown). Therefore, it should be emphasized that the screening for these compounds at lower concentrations in various biological assays is another interesting issue to delineate chemically high active constituents from this plant.
To quantitatively evaluate the effect of homoisoflavones in the lipoxygenase assay, four compounds (1 and 3–5) were further tested at different concentrations. As shown in , a concentration-dependent increase of lipoxygenase inhibition was demonstrated in individual homoisoflavones. The calculated IC50 values of four homoisoflavones (1 and 3–5) were 35.8 µM (1), 26.9 µM (3), 286 µM (4), and 15.8 µM (5), respectively. Among four homoisoflavones tested, 5 showed the strongest inhibition in lipoxygenase assay. The IC50 value of NDGA was 9.61 µM. Compared with NDGA, 5 appeared to possess at least 0.60-fold extent of inhibition capability, and other three (1, 3, and 4) also display 0.03 to 0.36-fold inhibition, implying these homoisoflavones may in part play a role in the suppression of lipoxygenase-related inflammation.
Figure 3. Effect of concentration of four homoisoflavones (1 and 3–5) from S. scilloides in lipoxygenase assay. Data shown represent mean ± S.D. from three experiments. NDGA was used as the standard sample.
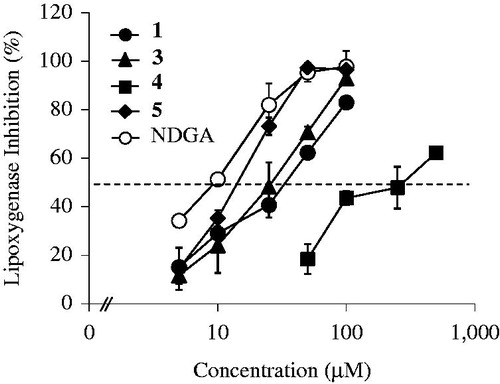
It is interesting to note that there may be some structure requirements of homoisoflavones for selective lipoxygenase inhibition. The catecyl group (3′,4′-dihydroxyphenyl) in the B-ring can confer higher enzyme inhibition than on the 4′-hydroxyphenyl group (see 1, 3 and 5 versus 4), while an O-methylation of the 7-hydroxyl group in the A-ring may not drastically change the inhibition capacity (see 1 versus 3). A double bond at the 3-position in the C-ring plausibly enhanced structural stability, thereby showing 1.69-times stronger inhibition (see 5 versus 3). In addition, the masking of hydroxyl group(s) at the 3′- and/or 4′-positions in the B-ring, implies their poor contribution to the structure requirement for the enzyme inhibition.
Macrophages originated from blood monocytes are thought to be an important defense line. When macrophages are activated by cytokines and/or microbial compounds, excess NO can be generated, thus the NO level reflects the inflammation state of macrophages in vitro and in vivo (Bogdan, Citation2001; Nathan, Citation1992). To gain insight into the physiological relevance of homoisoflavones with their potent anti-inflammatory effect, activated RAW264.7 mouse macrophage-like cells were incubated in the presence of these compounds plus LPS for 24 h. The culture media were collected and analyzed for determination of NO level to measure the extent of inflammation. As shown in , incubating the cells in the presence of six homoisoflavones (1–6) at 10 µM concentration showed 40.5–81.9% of NO production with a significant suppression (p < 0.001) in the LPS-activated macrophage cell model. In the case of 50 µM concentration tested, all nine homoisoflavones (1–9) showed 1.5–66.0% of NO production with a significant suppression (p < 0.001). It is interesting to note that four homoisoflavones (1 and 3–5) seemingly can demonstrate stronger inhibition than the others in accordance with the data obtained in lipoxygenase inhibition assays (see ). Previous reports show that several anti-inflammatory drugs and their candidates can possess inhibitory effect on NO generation from macrophages (Sasaki et al., Citation2007; Wang et al., Citation2008). Whether these homoisoflavones can inhibit the cellular lipoxygenase activity and suppress subsequent NO production in LPS-activated RAW264.7 cells still remains to be clarified.
Figure 4. Inhibitory effect of nine homoisoflavones (1–9) from S. scilloides on the NO production in LPS-stimulated RAW264.7 mouse macrophage-like cells. Cells were incubated in the presence of 10 µM (gray bar) or 50 µM (black bar) of individual compounds plus 100 ng/mL LPS for 24 h. The culture media were collected and analyzed for determination of NO level by the Griess method. Data shown represent mean ± S.D. from four experiments. #Significant differences from untreated cells (control), p < 0.001, and *Significant differences from LPS treated cells, p < 0.001. NO, nitric oxide; LPS, lipopolysaccharide.
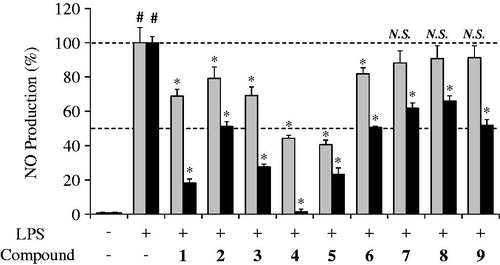
Conclusion
In this report, we demonstrated potential anti-inflammatory effects of the bulbs of S. scilloides and several homoisoflavones using lipoxygenase and hyaluronidase as the inflammation-related enzyme models. Among nine homoisoflavones tested, four (1 and 3–5) were found to possess moderate to high lipoxygenase inhibition. Calculated IC50 values indicated 5 as the compound responsible for strong lipoxygenase inhibition. In addition, all nine homoisoflavones tested at 1000 µM high concentration showed at least 16.2–58.0% hyaluronidase inhibition. Incubating cells in the presence of nine homoisoflavones demonstrated inhibitory effect in the NO production of LPS-activated macrophage cells as an inflammation model, and 4 as the compound responsible for the highest inhibition against the cellular NO production. These results may indicate a potential role of S. scilloides in treatment of dermal inflammation and disorders. In view of traditional usage of this plant, some homoisoflavones and also possibly other constituents found in this plant may be involved in its anti-inflammatory mechanism(s). Extensions of this work are warranted to fully elucidate the beneficial contributions of this plant in suppressing the progression of inflammation.
Declaration of interest
The authors have declared that no competing interest exists. This work was supported in part by Research Fund from the Aso Pharmaceutical Co., Ltd. (Kumamoto, Japan), and Research and Study Program/Project of Tokai University Educational System General Research Organization (Kanagawa, Japan).
References
- Anthon GE, Barret DM. (2003). Thermal inactivation of lipoxygenase and hydroperoxytrienoic acid lyase in tomatoes. Food Chem 81:275–9
- Araki H. (1985). The distribution of diploids and polypoids of the Scilla scilloides complex in Korea. Genetica 66:3–10
- Aronson NN, Davidson EA. (1967). Lysosomal hyaluronidase from rat liver I. Preparation. J Biol Chem 10:437–40
- Asada M, Sugie M, Inoue M, et al. (1997). Inhibitory effect of alginic acids on hyaluronidase and on histamine release from mast cells. Biosci Biotechnol Biochem 61:1030–2
- Bogdan C. (2001). Nitric oxide and the immune response. Nat Immunol 2:907–16
- Engstrom-Laurent A, Hellstrom S. (1990). The role of liver and kidneys in the removal of circulating hyaluronan. An experimental study in the rat. Connect Tissue Res 24:219–24
- Ferrara JJ, Reed RK, Dyess DL, et al. (1991). Increased hyaluronan flux from skin following burn injury. J Surg Res 50:240–4
- Giannaras A, Selig W, Ellis J, Hullinger T. (2005). The effect of a novel, dual function histamine H1 receptor antagonist/5-lipoxygenase enzyme inhibitor on in vivo dermal inflammation and extravasation. Eur J Pharmacol 506:265–71
- Girish KS, Kemparaju K. (2005a). Inhibition of Naja naja venom hyaluronidase by plant-derived bioactive components and polysaccharides. Biochemistry (Mosc) 70:948–52
- Gleason JG, Perchonock CD, Torphy T. (1986). Pulmonary and antiallergy agents. Annu Rep Med Chem 21:73–83
- Guerra F. (1946). Hyaluronidase inhibition by sodium salicylate in rheumatic fever. Science 103:686–7
- Henderson WR Jr. (1994). The role of leukotrienes in inflammation. Ann Intern Med 121:684–97
- Horton MR, McKee CM, Bao C, et al. (1998). Hyaluronan fragments synergize with interferon-gamma to induce the C-X-C chemokines mig and interferon-inducible protein-10 in mouse macrophages. J Biol Chem 273:35088–94
- Kakegawa H, Matsumoto H, Satoh T. (1985). Activation of hyaluronidase by metallic salts and compound 48/80, and inhibitory effect of anti-allergic agents on hyaluronidase. Chem Pharm Bull 33:642–6
- Kakegawa H, Matsumoto H, Satoh T. (1988). Inhibitory effects of hydrangenol derivatives on the activation of hyaluronidase and their antiallergic activities. Planta Med 54:385–9
- Komoda T, Kishi M, Abe N, et al. (2004). Novel lipoxygenase inhibitors, tetrapetalone B, C, and D from Streptomyces sp. Biosci Biotechnol Biochem 68:903–8
- Lee SM, Chun HK, Lee CH, et al. (2002). Eucosterol oligoglycosides isolated from Scilla scilloides and their anti-tumor activity. Chem Pharm Bull 50:1245–9
- Lewis RA, Austen KF, Soberman RJ. (1990). Leukotrienes and other products of the 5-lipoxygenase pathway. N Engl J Med 323:645–55
- Ling S-K, Tanaka T, Kouno I. (2003). Effects of iridoids on lipoxygenase and hyaluronidase activities and their activation by β-glucosidase in the presence of amino acids. Biol Pharm Bull 26:352–6
- Maekawa F. (1944). Prehistoric-naturalized plants to Japan proper. Acta Phytotax Geobot 13:274–9
- Marcocci L, Maguire JJ, Droy-Lefaix MT, Packer L. (1994). The nitric oxide-scavenging properties of Ginkgo biloba extract EGb 761. Biochem Biophys Res Commun 201:748–55
- Mikami Y, Nishino H, Ori K, et al. (1994). Lanosterol oligosaccharides from plants of the subfamily Scilloideae and their antitumor-promoter activity. Chem Pharm Bull 42:327–32
- Mio K, Stern R. (2002). Inhibitors of the hyaluronidases. Matrix Biol 21:31–7
- Namba T. (1993). The Encyclopedia of Wakan-Yaku. Osaka, Japan: Hoikusha
- Nathan C. (1992). Nitric oxide as a secretory product of mammalian cells. FASEB J 6:3051–64
- Nishida Y, Eto M, Ikeda T, et al. (2008). A new homostilbene and two new homoisoflavones from the bulbs of Scilla scilloides. Chem Pharm Bull 56:1022–5
- Nishida Y, Wada K, Toyohisa D, et al. (2013). Homoisoflavones as the antioxidants responsible from bulbs of Scilla scilloides. Nat Prod Res 27:2360–2
- Noda S. (1976). Outline of natural habitats and geographical distributions of Scilla scilloides in East Asia. Bull Cult Nat Sci Osaka Gakuin Univ 2:77–102
- Onarheim H, Missavage AE, Gunther RA, et al. (1991a). Marked increase of plasma hyaluronan after major thermal injury and infusion therapy. J Surg Res 50:259–65
- Onarheim H, Reed RK, Laurent TC. (1991b). Elevated hyaluronan blood concentrations in severely burned patients. Scand J Clin Lab Invest 51:693–7
- Ono M, Toyohisa D, Morishita T, et al. (2011). Three new nortriterpene glycosides and two new triterpene glycosides from the bulbs of Scilla scilloides. Chem Pharm Bull (Tokyo) 59:1348–54
- Ono M, Takatsu Y, Ochiai T, et al. (2012). Two new nortriterpenoid glycosides and a new phenylpropanoid glycoside from the bulbs of Scilla scilloides. Chem Pharm Bull (Tokyo) 60:1314–19
- Ono M, Ochiai T, Yasuda S, et al. (2013). Five new nortriterpenoid glycosides from the bulbs of Scilla scilloides. Chem Pharm Bull (Tokyo) 61:592–8
- Reissig JL, Strominger JL, Leloir LF. (1955). A modified colorimetric method for the estimation of N-acetylamino sugars. J Biol Chem 217:959–66
- Samuelsson B. (1983). Leukotrienes: Mediators of immediate hypersensitivity reactions and inflammation. Science 220:568–75
- Sasaki Y, Hosokawa T, Nagai M, Nagumo S. (2007). In vitro study for inhibition of NO production about constituents of Sappan lignum. Biol Pharm Bull 30:193–6
- Sawabe Y, Nakagomi K, Iwagami S, et al. (1992). Inhibitory effects of pectic substances on activated hyaluronidase and histamine release from mast cells. Biochim Biophys Acta 1137:274–8
- Swarnalatha D, Satyanarayana T. (2011). Anti inflammatory activity of Bauhinia tomentosa (stem bark and roots) and Bauhinia vahlii roots. J Global Trends Pharmaceut Sci 2:108–16
- Suda I, Hajika M, Nishiba Y, et al. (1995). Simple and rapid method for the selective detection of individual lipoxygenase isoenzymes in soybean seeds. J Agric Food Chem 43:742–7
- Wang B-S, Yu HM, Chang LW, et al. (2008). Protective effects of pu-erh tea on LDL oxidation and nitric oxide generation in macrophage cells. LWT – Food Sci Technol 41:1122–32
- Yamaguchi T. (1959). The journey of the Lycoris radiata herb to Japan as a relief plant in a rice lean year. Nat Sci Mus Tokyo 26:147–53
- Yamazaki R, Aiyama R, Matsuzaki T, et al. (1998). Anti-inflammatory effect of YPE-01, a novel diarylheptanoid derivative, on dermal inflammation in mice. Inflamm Res 47:182–6
- Yeo E-J, Kim K-T, Han YS, et al. (2006). Antimicrobial, anti-inflammatory, and anti-oxidative activities of Scilla scilloides (Lindl.) Druce root extract. Food Sci Biotechnol 15:639–42
- Yodsaoue O, Cheenpracha S, Karalai C, et al. (2009). Anti-allergic activity of principles from the roots and heartwood of Caesalpinia sappan on antigen-induced beta-hexosaminidase release. Phytother Res 23:1028–31