Abstract
Context: The herbal composition Gyeongshingangjeehwan 18 (GGEx18) extracted from Rheum palmatum L. (Polygonaceae), Laminaria japonica Aresch (Laminariaceae), and Ephedra sinica Stapf (Ephedraceae) is traditionally used as an anti-obesity drug by local clinics in Korea.
Objective: This study investigates the effects of GGEx18 on visceral obesity and insulin resistance and determines the molecular mechanisms involved in this process.
Materials and methods: After C57BL/6J mice were fed a high-fat diet supplemented with GGEx18 (125, 250, and 500 mg/kg) for 8 weeks and 3T3-L1 adipocytes were treated with GGEx18 (0.1, 1, and 10 μg/ml); variables and determinants of visceral obesity and insulin resistance were measured using in vivo and in vitro approaches.
Results: Administration of GGEx18 to obese mice decreased visceral adipose tissue weight with an ED50 value of 232 mg/kg. 3T3-L1 adipocytes treated with GGEx18 showed a reduction in lipid accumulation with an ED50 value of 0.7 µg/ml. GGEx18 significantly increased the expression of fatty acid oxidation genes, including adiponectin, AMPKs, PPARα and its target enzymes, and CPT-1, in both mesenteric adipose tissues and 3T3-L1 cells. However, GGEx18 treatment decreased the mRNA levels of adipocyte marker genes such as PPARγ, aP2, TNFα, and leptin. GGEx18 normalized hyperglycemia and hyperinsulinemia in obese mice. Blood glucose levels of GGEx18-treated mice were significantly reduced during oral glucose tolerance tests compared with obese controls.
Discussion and conclusion: These results suggest that GGEx18 may treat visceral obesity and visceral obesity-related insulin resistance by upregulating the visceral adipose expression of fatty acid oxidative genes.
Introduction
Increased visceral adipose tissue mass is closely associated with insulin resistance (Jensen, Citation2006; Kissebah, Citation1997). Human and animal studies demonstrate that visceral obesity due to adipocyte hypertrophy leads to insulin resistance and diabetes, whereas restoration to normal adipose tissue levels alleviates the insulin-resistant state (Brunzell & Hokanson, Citation1999; Murphy et al., Citation1997; Spiegelman & Flier, Citation1996; Wickelgren, Citation1998). Hypertrophic adipocytes of obese, insulin-resistant rodents overexpress leptin, TNFα, and adipocyte fatty acid-binding protein (aP2), which are involved in insulin resistance pathogenesis (Kralisch & Fasshauer, Citation2013; Okuno et al., Citation1998). Thus, the conversion of hypertrophic adipocytes into small adipocytes appears to play an important role in the alleviation of both insulin resistance and diabetes (de Souza et al., Citation2001; Okuno et al., Citation1998).
Adiponectin is a protein hormone that modulates several metabolic processes, including glucose metabolism and fatty acid oxidation (Díez & Iglesias, Citation2003). Adiponectin is secreted exclusively from adipose tissue (Nedvídková et al., Citation2005) and its levels are inversely correlated with body fat (Ukkola & Santaniemi, Citation2002). Adiponectin activates the downstream target AMP-activated protein kinase (AMPK), a serine/threonine kinase that plays an important role in energy metabolism at both the cellular and whole-organism levels (Hardie, 2008; Kemp et al., Citation1999; Padmalayam & Suto, Citation2013). AMPK activation induces the expression of peroxisome proliferator-activated receptor α (PPARα) and carnitine palmitoyltransferase I (CPT-1), which increase fatty acid oxidation and improve insulin sensitivity (Bijland et al., Citation2013; Long & Zierath, Citation2006; Schreurs et al., Citation2010). Targeting any of these fatty acid oxidation regulatory molecules could provide a treatment for obesity and obesity-related metabolic diseases.
Gyeongshingangjeehwan 18 (GGEx18) is an herbal drug composed of three medicinal plants: Rheum palmatum L. (Polygonaceae), Laminaria japonica Aresch (Laminariaceae), and Ephedra sinica Stapf (Ephedraceae). It is currently being used as an anti-obesity drug by local clinics in Korea. These three herbs are reported to have anti-obesity, antidiabetes, and lipid-reducing effects (Boozer et al., Citation2001; Huang et al., Citation2010; Xue et al., Citation2010; You et al., Citation2009; Zheng et al., Citation2010), supporting the belief that GGEx18 may reduce obesity and related metabolic disorders. Our previous study showed that GGEx18 has increased anti-obesity effects and safety when compared with the individual herbs (Yoon et al., Citation2010). We also found that GGEx18 decreased obesity and hepatic steatosis in a high-fat diet-induced obesity mouse model (Shin & Yoon, Citation2012; Shin et al., Citation2012). Therefore, we hypothesized that GGEx18 can effectively regulate visceral adipose tissue mass and insulin resistance.
The objectives of the present study were to determine whether GGEx18 stimulates visceral adipose expression of fatty acid oxidation genes and to examine the subsequent effects of GGEx18 on visceral obesity and insulin resistance. Our data demonstrate that GGEx18 treatment increased mRNA expression of fatty acid oxidation enzymes in visceral adipose tissue. This leads to the alleviation of visceral obesity and insulin resistance without demonstrating toxic effects.
Materials and methods
GGEx18 preparation
GGEx18 was prepared from food-grade aqueous extracts of the three herbs: L. japonica, E. sinica, and R. palmatum (Hwalim, Busan, Korea). The composition of GGEx18 was described previously (Shin et al., Citation2012). The percent composition of each ingredient is equivalent to the formula used to treat human patients. Voucher specimens for E. sinica (FOS-05-04), L. japonica (FOS-05-05), and R. palmatum (FOS-05-06) were deposited in the Department of Formula Sciences, Dongeui University, Busan, South Korea. Briefly, the three dried herbs were weighed, combined, and boiled in distilled water for 22 h at 95 °C. The aqueous extracts were then filtered and freeze-dried under vacuum to produce GGE × 18. The preparation of GGEx18 was accomplished in 27.74% overall yield.
Animal study
Eight-week-old, male wild-type C57BL/6J mice (n = 8/group) were purchased from Central Lab Animal (Seoul, Korea) and randomly divided into five groups. Mice were fed a low-fat diet (normal group; 13% kcal fat, CJ, Incheon, Korea), a high-fat diet (control group; 45% kcal fat, Research Diets, New Brunswick, NJ), or the high-fat diet supplemented with one of the three GGEx18 doses (125, 250, or 500 mg/kg/d) for 8 weeks. At the end of the study, the mice were sacrificed under diethyl ether anesthesia. The visceral adipose tissues were harvested, weighed, snap-frozen in liquid nitrogen, and stored at −80 °C. Additional sections of visceral adipose tissues were prepared for histological analyses.
Serum glucose and insulin levels were measured using an Accu-Chek Performa System (Roche, Mannheim, Germany) and an insulin radioimmunoassay kit (Linco, St. Charles, MO), respectively. Glucose tolerance tests were carried out on all five test groups. Glucose (2 g/kg body weight) was administered orally to the mice. Blood glucose levels were measured using the Accu-Chek Performa System at the indicated time intervals. All animal experiments were approved by the Institutional Animal Care and Use Committee of Dongeui University and followed National Research Council Guidelines.
Induction of preadipocyte differentiation
Mouse 3T3-L1 cells (ATCC) were cultured in 6-well plates with DMEM containing 10% bovine calf serum (Invitrogen, Carlsbad, CA) and grown to 100% confluency. The cells were incubated two additional days followed by medium replacement with MDI induction medium (day 0) containing 0.5 mM 1-methyl-3-isobutyl-xanthine, 1 μM dexamethasone, and 1 μg/ml insulin in DMEM with 10% fetal bovine serum (FBS) (Invitrogen, Carlsbad, CA). The cultures were incubated for two additional days to induce adipocyte differentiation. Thereafter, cells were cultured in DMEM with 10% FBS for the rest of the differentiation process. Drug treatments were administered from day 0 to day 2 only, and the medium was changed every other day. Cells were treated with varying concentrations of GGEx18 (0.1, 1, or 10 μg/ml), 10 μM fenofibrate, or 10 μM Wy14,643. GGEx18 was dissolved in distilled water. Fenofibrate and Wy14,643 were dissolved in ethanol. Cells were stained at day 8 with Oil-red O and photographed. For quantitative analysis, Oil-red O was eluted with isopropanol for 10 min and the optical density was measured at 570 nm.
Histological analysis
Visceral adipose tissue was fixed in 10% phosphate-buffered formalin for 1 d and processed in a routine manner for paraffin sectioning. Tissue sections (5 μm) were cut and stained with hematoxylin and eosin (HE) for microscopic examination. To quantify adipocyte size, the HE-stained sections were analyzed using the Image-Pro Plus analysis system (Media Cybernetics, Bethesda, MD).
Reverse transcription-polymerase chain reaction (RT-PCR)
Total cellular RNA from the visceral adipose tissues and differentiated 3T3-L1 adipocytes was prepared using Trizol reagent (Invitrogen). Total RNA (2 µg) was reverse-transcribed into cDNA using Moloney murine leukemia virus reverse transcriptase (MMLV-RT; Promega, Madison, WI) and an appropriate antisense primer. Briefly, the RNA was denatured for 5 min at 72 °C and immediately placed on ice for 5 min. Denatured RNA was mixed with MMLV-RT, MMLV-RT buffer, and dNTPs, followed by a 1 h incubation at 42 °C. Synthesized cDNA fragments were PCR amplified in a MJ Research Thermocycler (Waltham, MA). The PCR primers used for gene expression analysis are listed in . The cDNA was mixed with PCR primers, Taq DNA polymerase (Nanohelix, Daejeon, Korea), and dNTPs. The PCR reaction consisted of 30 cycles of denaturation for 1 min at 94 °C, annealing for 1 min at 52–58 °C, and elongation for 1 min at 72 °C. The PCR products were analyzed by electrophoresis on a 1% agarose gel. PCR products were quantified from the agarose gels using the Syngene GeneGenius Gel Documentation System (Syngene, Cambridge, UK). Relative expression levels are presented as a ratio of target gene cDNA versus β-actin cDNA.
Table 1. PCR primers and conditions.
Statistical analysis
All values are expressed as the mean ± standard deviation (SD). Statistical analysis was performed by ANOVA followed by either post hoc Tukey’s multiple comparison test or Dunnett’s test.
Results
Visceral adipose tissue mass and adipocyte size in high-fat diet-induced obese mice
To determine whether GGEx18 regulates visceral obesity in mice fed a high-fat diet, we measured the visceral adipose tissue weight and adipocyte size. High-fat diet-induced, obese control mice had a higher visceral adipose tissue mass (452%) than normal, lean mice fed on a low-fat diet (). In contrast, administration of GGEx18 to obese mice decreased visceral adipose tissue weight with an ED50 value of 232 mg/kg. GGEx18 significantly reduced the visceral adipose tissue weight of control mice by 46 and 24% at doses of 250 and 500 mg/kg/d, respectively, when compared with control mice. Similarly, histological analysis showed that the visceral adipocytes were significantly smaller in GGEx18-treated mice than in untreated control mice (). GGEx18 significantly decreased visceral adipocyte size by 35 and 18% at doses of 250 and 500 mg/kg/d, respectively, when compared with control mice. In addition, toxicological analysis revealed that the 8-week treatment with GGEx18 did not affect serum levels of glutamic oxaloacetic transaminase and glutamic pyruvic transaminase, and that it did not induce liver and heart lesions (data not shown).
Figure 1. Visceral adipose tissue weight and histology of visceral adipocytes. Adult male C57BL/6J mice were fed a low-fat diet (normal), a high-fat diet (control), or the high-fat diet supplemented with 125, 250, or 500 mg/kg/d GGEx18 for 8 weeks. (A) At the end of study, visceral adipose tissue weights were measured. (B) The size of adipocytes in a fixed area (1 000 000 µm2) was measured. All values are expressed as the mean ± SD. #p < 0.05 compared with the normal group, *p < 0.05 compared with the obese control group. (C) Representative hematoxylin and eosin-stained sections (5 µm thick) of mesenteric adipose tissue.
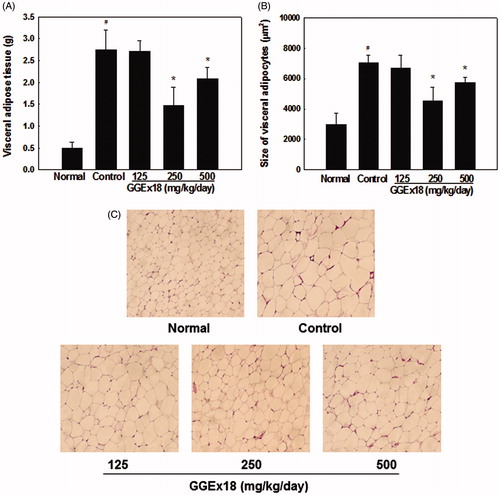
Lipid accumulation in 3T3-L1 adipocytes
The development of obesity is closely associated with adipogenesis including cytoplasmic lipid accumulation. To test whether GGEx18 could reduce lipid accumulation, we treated differentiated 3T3-L1 adipocytes with GGEx18. Differentiated 3T3-L1 adipocytes (control) showed lipid accumulation as expected, demonstrated by an increase in Oil red O staining compared with non-differentiated cells (ND) (). However, 3T3-L1 adipocytes treated with GGEx18 showed a reduction in lipid accumulation with an ED50 value of 0.7 µg/ml. GGEx18 decreased accumulation of lipid droplets in a dose-dependent manner by 41, 54, and 70% at 0.1, 1, and 10 μg/ml concentrations, respectively, when compared with the control. Treatment with the PPARα activators fenofibrate and Wy14,643 also decreased triglyceride droplets in 3T3-L1 adipocytes by 62 and 74%, respectively. These results suggest that, similar to PPARα activators, GGEx18 suppresses lipid accumulation in adipocytes.
Figure 2. Lipid accumulation in 3T3-L1 cells. (A) 3T3-L1 preadipocytes (ND) were differentiated into mature adipocytes (control). Differentiated control cells were treated with GGEx18, fenofibrate (FF) or Wy14,643 (Wy). Shown are representative Oil red O-stained cells. (B) Quantitative analysis of triglyceride contents. All values are expressed as the mean ± SD. #p < 0.05 compared with the ND group, *p < 0.05 compared with the control group.
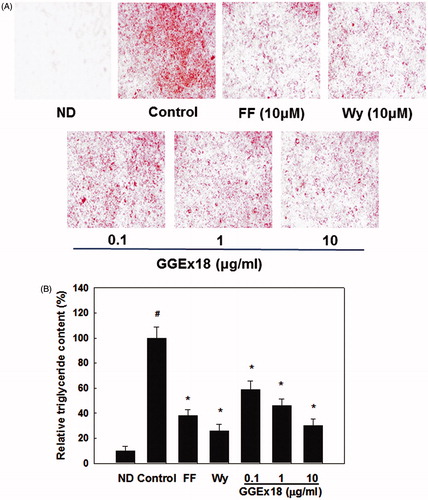
Fatty acid oxidation gene expression in mesenteric adipose tissue and differentiated 3T3-L1 adipocytes
We hypothesized that GGEx18 reduces visceral adipose tissue mass and adipocyte size by changing the expression of fatty acid oxidation genes in high-fat diet-fed mice. We first measured the mRNA levels of adiponectin, the α1 and α2 catalytic subunits of AMPK, and the AMPK target gene acetyl-CoA carboxylase (ACC), which produces malonyl-CoA to inhibit CPT-1. Obese control mice had lower mRNA levels of adiponectin, AMPKα1, and AMPKα2 than lean normal mice. However, mice treated with 250 mg/kg/d of GGEx18 showed that adiponectin, AMPKα1, and AMPKα2 mRNA levels were significantly elevated by 83, 152, and 153%, respectively, compared with controls (). In contrast, GGEx18 treatment decreased ACC expression by 61%.
Figure 3. The mRNA expression levels of genes involved in fatty acid oxidation in visceral adipose tissue of obese mice. (A) Adult male C57BL/6J mice were fed a low-fat diet (normal), a high-fat diet (control), or the high-fat diet supplemented with 250 mg/kg/d GGEx18 for 8 weeks. Total cellular RNA was extracted from visceral adipose tissue and mRNA levels were measured using RT-PCR. All values are expressed as the mean ± SD of relative density units using β-actin as a reference. #p < 0.05 compared with the normal group, *p < 0.05 compared with the obese control group. (B) Representative RT-PCR bands from one of the three independent experiments are shown.
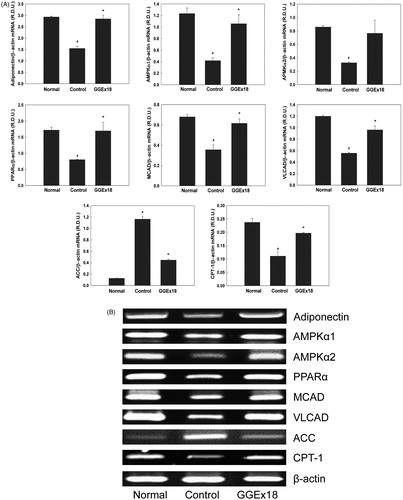
Next, we measured visceral adipose tissue mRNA levels of CPT-1, PPARα, and the PPARα target genes medium chain acyl-CoA dehydrogenase (MCAD) and very long chain acyl-CoA dehydrogenase (VLCAD). Treatment of obese mice with 250 mg/kg/d of GGEx18 increased the mRNA levels of CPT-1, PPARα, MCAD, and VLCAD by 111, 111, 73%, and 72%, respectively, compared with controls ().
Differentiated T3T-L1 control cells showed lower mRNA levels of PPARα, MCAD, and VLCAD than ND cells. However, a 1 μg/ml dose of GGEx18 elevated the mRNA levels of PPARα, MCAD, and VLCAD by 64, 77, and 62%, respectively, relative to untreated, differentiated controls (). Similarly, a 1 μg/ml dose of GGEx18 increased the mRNA levels of AMPKα1 and AMPKα2, and CPT-1 by 22, 104, and 112% respectively. The 1 μg/ml dose of GGEx18 decreased ACC mRNA levels by 17%, when compared with the control (). Taken together, these results suggest that GGEx18 may decrease visceral adipose tissue mass, adipocyte size, and adipocyte lipid accumulation, in part, through the increased expression of fatty acid oxidation genes.
Figure 4. The mRNA expression levels of genes involved in fatty acid oxidation in 3T3-L1 cells. (A) 3T3-L1 preadipocytes (ND) were differentiated into mature adipocytes (control). Differentiated control cells were treated with GGEx18, fenofibrate (FF) or Wy14,643 (Wy). Total cellular RNA was extracted from cells and mRNA levels were measured using RT-PCR. All values are expressed as the mean ± SD of relative density units using β-actin as a reference. #p < 0.05 compared with the ND group, *p < 0.05 compared with the control group. (B) Representative RT-PCR bands from one of the three independent experiments are shown.
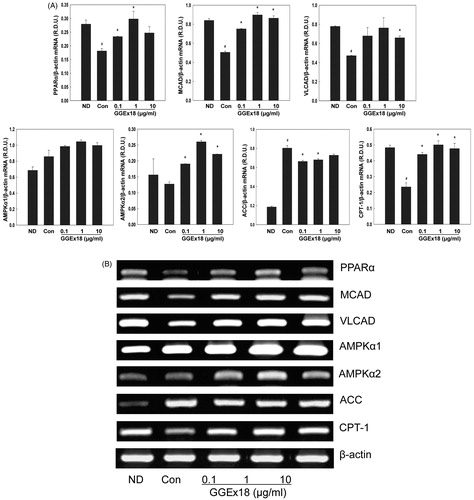
Adipocyte marker gene expression in mesenteric adipose tissue and differentiated 3T3-L1 adipocytes
We tested the effects of GGEx18 on the expression of adipocyte marker genes, such as TNFα, aP2, and leptin, which are overexpressed in obese, insulin-resistant rodents with hypertrophic adipocytes. Compared with normal mice, adipose tissue from obese control mice had 33, 60, and 39% higher mRNA levels of aP2, TNFα, and leptin, respectively (). Consistent with the effects of GGEx18 on adipose tissue size, GGEx18 (250 mg/kg/d) decreased aP2, TNFα, and leptin mRNA levels by 19, 34, and 29%, respectively, compared with obese controls.
Figure 5. The mRNA expression levels of adipocyte marker genes in visceral adipose tissue of obese mice. (A) Adult male C57BL/6J mice were fed a low-fat diet (normal), a high-fat diet (control), or the high-fat diet supplemented with 250 mg/kg/d GGEx18 for 8 weeks. Total cellular RNA was extracted from visceral adipose tissue and mRNA levels were measured using RT-PCR. All values are expressed as the mean ± SD of relative density units using β-actin as a reference. #p < 0.05 compared with the normal group, *p < 0.05 compared with the obese control group. (B) Representative RT-PCR bands from one of the three independent experiments are shown.
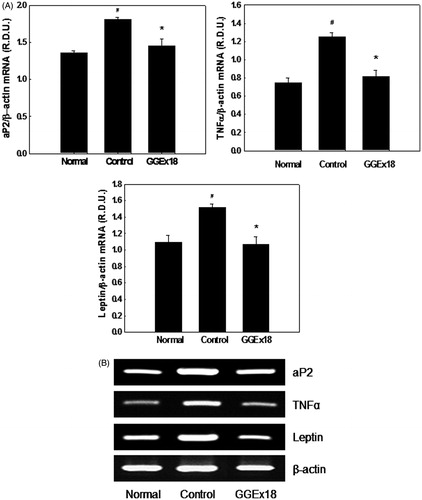
Adipogenesis is initiated by activation of a key transcription factor, PPARγ, which is responsible for inducing the expression of adipogenic genes. We tested the effects of GGEx18 on the adipogenic gene expression of 3T3-L1 cells, including PPARγ, aP2, and leptin. Compared with ND, control cells had 38, 49, and 105% higher mRNA levels of PPARγ, aP2, and leptin, respectively (). Consistent with the lipid accumulation inhibition effects, GGEx18 decreased PPARγ, aP2, and leptin mRNA levels. A 1 μg/ml dose of GGEx18 reduced PPARγ, aP2, and leptin mRNA levels by 21, 24, and 41%, respectively, compared with controls.
Figure 6. The mRNA expression levels of adipogenic genes in 3T3-L1 cells. (A) 3T3-L1 preadipocytes (ND) were differentiated into mature adipocytes (control). Differentiated control cells were treated with GGEx18, fenofibrate (FF) or Wy14,643 (Wy). Total cellular RNA was extracted from cells and mRNA levels were measured using RT-PCR. All values are expressed as the mean ± SD of relative density units using β-actin as a reference. #p < 0.05 compared with the ND group, *p < 0.05 compared with the control group. (B) Representative RT-PCR bands from one of the three independent experiments are shown.
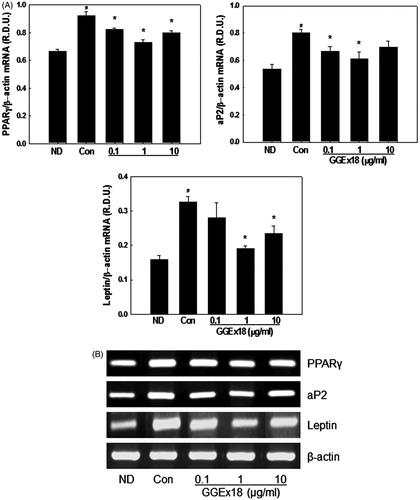
Circulating levels of insulin and glucose
To examine whether GGEx18 regulates visceral obesity-induced insulin resistance, serum levels of glucose and insulin were measured in high-fat diet-induced obese control mice and the insulin-to-glucose (I/G) ratios were calculated. Serum insulin and glucose levels were higher by 3246 and 52%, respectively, in obese control mice when compared with lean normal mice (). However, GGEx18 treatment showed serum insulin levels 57, 80, and 84% and serum glucose levels 29, 31, and 35% lower than obese control mice at 125, 250, and 500 mg/kg/d doses, respectively. I/G ratios increased about 20-fold in obese mice compared with normal mice, suggesting insulin resistance in the obese mice. Consistent with the reduced visceral fat mass and adipocyte size, I/G ratios were significantly lower in GGEx18-treated mice at 250 and 500 mg/kg/d doses. These results indicate that GGEx18 inhibits high-fat diet-induced hyperglycemia and hyperinsulinemia.
Figure 7. Serum (A) glucose levels, (B) insulin levels, and (C) insulin-to-glucose ratios. Adult male C57BL/6J mice were fed a low-fat diet (normal), a high-fat diet (control), or the high-fat diet supplemented with 125, 250, and 500 mg/kg/d for 8 weeks. All values are expressed as the mean ± SD. #p < 0.05 compared with the normal group, *p < 0.05 compared with the control group.
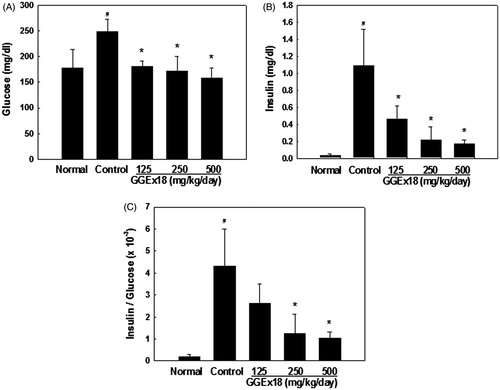
Oral glucose tolerance test
The effects of GGEx18 on changes in blood glucose concentrations were measured using an oral glucose tolerance test (OGTT) in the high-fat diet-fed mice. After 8 weeks of treatment with GGEx18, mice exhibited a significant reduction in blood glucose levels following oral glucose administration when compared with obese control mice (). These results indicate that GGEx18 may improve glucose tolerance in mice with diet-induced obesity.
Figure 8. Changes in blood glucose levels during the oral glucose tolerance test. Adult male C57BL/6J mice were fed a low-fat diet (normal), a high-fat diet (control), or the high-fat diet supplemented with 125, 250, and 500 mg/kg/d for 8 weeks. After a 12-h fast, mice orally received glucose (2 g/kg body weight). All values are expressed as the mean ± SD. #p < 0.05 compared with the normal group, *p < 0.05 compared with the control group.
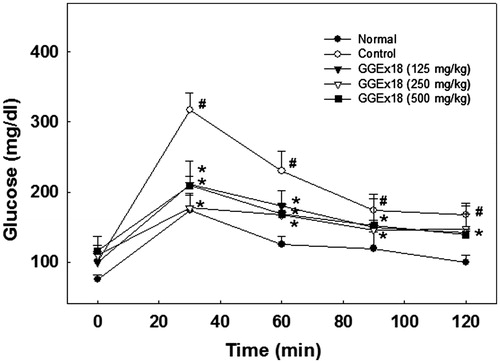
Discussion
Our previous study demonstrated that GGEx18 inhibits obesity and hepatic steatosis by activating liver and skeletal muscle PPARα (Shin & Yoon, Citation2012; Shin et al., Citation2012). Thus, we investigated the effects of GGEx18 on visceral obesity and insulin resistance in diet-induced obese mice and examined the cellular mechanisms involved in this event.
Our results show that GGEx18 reduced visceral adipose tissue mass increases in a high-fat diet-induced obesity mouse model. High-fat diet-fed mice had much higher visceral fat mass compared with normal, low-fat diet-fed mice. However, administration of GGEx18 (250 and 500 mg/kg/d) to high-fat diet-fed mice for 8 weeks significantly reduced visceral fat mass when compared with untreated control mice. Consistent with the animal study, our human study also showed that administration of GGEx18 (800–900 mg/d) to obese patients for 6 months lowered body weights and abdominal fat area compared with untreated obese patients (data not shown). Treated patients did not show any other side effects except for negligible, slight symptoms, such as dry mouth, insomnia, and constipation. These results suggest that GGEx18 may be an effective and safe treatment to prevent visceral obesity.
In addition to the effects of GGEx18 on visceral fat mass, histological examination of mesenteric adipose tissue revealed that GGEx18 (250 and 500 mg/kg/d) significantly reduced the average adipocyte size in high-fat diet-induced obese mice. Concomitantly, GGEx18 increased the number of small adipocytes, while decreasing the number of large adipocytes in a fixed area (data not shown), indicating that GGEx18 may induce the conversion of large adipocytes into smaller ones. Since large adipocytes are associated with insulin resistance, whereas smaller adipocytes are associated with insulin sensitivity (Kadowaki, Citation2000; Kubota et al., Citation1999; Okuno et al., Citation1998); it is likely that GGEx18 may decrease insulin resistance by inhibiting adipocyte hypertrophy in obese mice.
It is known that lipid accumulation is the main volume determinant of adipocytes and induces adipocyte hypertrophy. Conversion of preadipocytes into lipid-containing adipocytes is closely associated with obesity development (Camp et al., Citation2002). Obesity reduction can be achieved by inhibiting lipid accumulation in adipocytes (Otto & Lane, Citation2005). Consistent with the in vivo data, Oil red O staining and microscopy revealed that GGEx18 decreased lipid accumulation in cultured 3T3-L1 adipocytes. Moreover, similar to PPARα activators such as fenofibrate and Wy14,643, GGEx18 suppressed lipid accumulation in adipocytes. These results suggest that GGEx18 prevents lipid accumulation in 3T3-L1 adipocytes, leading to obesity inhibition, possibly through adipose PPARα activation.
We next examined whether the decrease in visceral adipose tissue mass and adipocyte size following GGEx18 treatment may result from stimulating adipose expression of fatty acid β-oxidation genes. We observed that GGEx18 increased mRNA levels of fatty acid oxidation-related genes such as adiponectin, AMPKα1, AMPKα2, CPT-1, and PPARα and its target genes. This treatment decreased ACC mRNA levels. GGEx18 similarly affected the mRNA levels of these genes in differentiated 3T3-L1 adipocytes. Adiponectin has been shown to increase fatty acid oxidation and insulin sensitivity, and reduce serum glucose levels (Díez & Iglesias, Citation2003; Nedvídková et al., Citation2005) and its expression decreases in obese patients (Díez & Iglesias, Citation2003; Ukkola & Santaniemi, Citation2002). Studies indicate that AMPK and PPARα mediate most of the adiponectin effects (Dunmore & Brown, Citation2013; Padmalayam & Suto, Citation2013). Adiponectin increases AMPK expression and activity, which results in PPARα and CPT-1 activation. PPARα modulates the expression of numerous genes critical for lipid homeostasis and obesity (Yoon et al., Citation2002, Citation2003). PPARα target genes include those involved in the plasma triglyceride hydrolysis, fatty acid uptake and binding, and fatty acid β-oxidation (Yoon, Citation2009, Citation2010). PPARα plays important roles in liver and skeletal muscle fatty acid oxidation and PPARα activators may affect adipose tissue metabolism (Cabrero et al., Citation2001; Jeong & Yoon, Citation2009; Vazque et al., Citation2001). AMPK also stimulates CPT-1 activity both through ACC inhibition and PPARα activation. Enhancing the fatty acid oxidation pathway could potentially prevent or treat obesity and insulin resistance. Insulin sensitizers like metformin generally act through AMPK-triggered pathways. Drugs regulating AMPK and its downstream targets may potentially modulate obesity and insulin resistance. These results suggest that GGEx18 may reduce visceral obesity, in part, through adipose activation of PPARα and the AMPK-ACC-CPT1 pathway.
Hypertrophic adipocytes produce and secrete adipokines that are implicated in the development of insulin resistance (Dunmore & Brown, Citation2013). Since adipokines such as aP2, leptin, and TNFα are reported to contribute to obesity-related insulin resistance (Dunmore & Brown, Citation2013; Kralisch & Fasshauer, Citation2013), decreases in leptin, TNFα, and aP2 expression may contribute to its amelioration. In parallel with adipocyte size reduction, GGEx18 decreased mRNA levels of aP2, TNFα, and leptin in the mesenteric adipose tissues of obese mice. Moreover, GGEx18 also reduced the expression of adipogenic genes, such as PPARγ, aP2, and leptin in differentiated 3T3-L1 adipocytes. Thus, it is likely that GGEx18 could alleviate insulin resistance, partly due to the reduced signaling molecule expression in hypertrophic adipocytes.
GGEx18 decreased both blood insulin and glucose levels. Diet-induced obese control mice showed markedly increased hyperglycemia, hyperinsulinemia, and I/G ratios compared with lean normal mice. The I/G ratio is used as an index for insulin resistance (Hrnciar, Citation1993). This ratio increases during insulin resistance development because increased insulin is necessary to maintain normal glucose levels. Control mice had approximately 20-fold higher I/G ratios than normal mice, indicating that the control mice had become insulin resistant. However, GGEx18 treatment produced serum glucose levels similar to normal levels and serum insulin levels were decreased by 57–84% when compared with control mice. Moreover, GGEx18 significantly decreased I/G ratios at all concentrations administered in this study. In parallel to the fasting glucose-lowering effects, GGEx18 decreased blood glucose levels during OGTT. These results suggest that GGEx18 may inhibit diet-induced insulin resistance.
In conclusion, these studies demonstrate that GGEx18 increases the expression of fatty acid β-oxidation genes in the visceral adipose tissue of obese, insulin-resistant mice. These changes led to decreased adipocyte size, lower adipose marker gene expression, and decreased serum glucose and insulin levels, reducing the incidence of visceral obesity and insulin resistance. Based on our results, GGEx18 appears to utilize many of the desired anti-obesity mechanisms for modulating visceral obesity and insulin resistance and could potentially be developed into a treatment for these conditions.
Declaration of interest
This work supported by the National Research Foundation of Korea (NRF) Grant funded by the Korea Government (MEST) (2012R1A1A3002100 and 2012R1A2A2A01004508). The authors report no conflicts of interest. The authors alone are responsible for the content and writing of the paper.
References
- Bijland S, Mancini SJ, Salt IP. (2013). Role of AMP-activated protein kinase in adipose tissue metabolism and inflammation. Clin Sci 124:491–507
- Boozer CN, Nasser JA, Heymsfield SB, et al. (2001). An herbal supplement containing Ma Huang–Guarana for weight loss: A randomized, double-blind trial. Int J Obes 25:316–24
- Brunzell JD, Hokanson JE. (1999). Dyslipidemia of central obesity and insulin resistance. Diabetes Care 22:C10–3
- Cabrero A, Alegret M, Sánchez RM, et al. (2001). Bezafibrate reduces mRNA levels of adipocyte markers and increases fatty acid oxidation in primary culture of adipocytes. Diabetes 50:1883–90
- Camp HS, Ren D, Leff T. (2002). Adipogenesis and fat-cell function in obesity and diabetes. Trends Mol Med 8:442–7
- de Souza CJ, Eckhardt M, Gagen K, et al. (2001). Effects of pioglitazone on adipose tissue remodeling within the setting of obesity and insulin resistance. Diabetes 50:1863–71
- Díez JJ, Iglesias P. (2003). The role of the novel adipocyte-derived hormone adiponectin in human disease. Eur J Endocrinol 148:293–300
- Dunmore SJ, Brown JE. (2013). The role of adipokines in β-cell failure of type 2 diabetes. J Endocrinol 216:T37–45
- Hardie DG. (2008). AMPK: A key regulator of energy balance in the single cell and the whole organism. Int J Obes 32:S7–12
- Hrnciar J. (1993). Methods of measuring hyperinsulinism and insulin resistance. Vnitr Lek 39:481–6
- Huang L, Wen K, Gao X, Liu Y. (2010). Hypolipidemic effect of fucoidan from Laminaria japonica in hyperlipidemic rats. Pharm Biol 48:422–6
- Jensen MD. (2006). Is visceral fat involved in the pathogenesis of the metabolic syndrome? Human model. Obesity 14:20S–4
- Jeong S, Yoon M. (2009). Fenofibrate inhibits adipocyte hypertrophy and insulin resistance by activating adipose PPARalpha in high fat diet-induced obese mice. Exp Mol Med 41:397–405
- Kadowaki T. (2000). Insights into insulin resistance and type 2 diabetes from knockout mouse models. J Clin Invest 106:459–65
- Kemp BE, Mitchelhill KI, Stapleton D, et al. (1999). Dealing with energy demand: The AMP-activated protein kinase. Trends Biochem Sci 24:22–5
- Kissebah AH. (1997). Central obesity: Measurement and metabolic effects. Diabetes Rev 5:8–20
- Kralisch S, Fasshauer M. (2013). Adipocyte fatty acid binding protein: A novel adipokine involved in the pathogenesis of metabolic and vascular disease? Diabetologia 56:10–21
- Kubota N, Terauchi Y, Miki H, et al. (1999). PPAR gamma mediates high-fat diet-induced adipocyte hypertrophy and insulin resistance. Mol Cell 4:597–609
- Long YC, Zierath JR. (2006). AMP-activated protein kinase signaling in metabolic regulation. J Clin Invest 116:1776–83
- Murphy JE, Zhou S, Giese K, et al. (1997). Long-term correction of obesity and diabetes in genetically obese mice by a single intramuscular injection of recombinant adeno-associated virus encoding mouse leptin. Proc Natl Acad Sci USA 94:13921–6
- Nedvídková J, Smitka K, Kopský V, Hainer V. (2005). Adiponectin, an adipocyte-derived protein. Physiol Res 54:133–40
- Okuno A, Tamemoto H, Tobe K, et al. (1998). Troglitazone increases the number of small adipocytes without the change of white adipose tissue mass in obese Zucker rats. J Clin Invest 101:1354–61
- Otto TC, Lane MD. (2005). Adipose development: From stem cell to adipocyte. Crit Rev Biochem Mol Biol 40:229–42
- Padmalayam I, Suto M. (2013). Role of adiponectin in the metabolic syndrome: Current perspectives on its modulation as a treatment strategy. Curr Pharm Des 19:5755–63
- Schreurs M, Kuipers F, van der Leij FR. (2010). Regulatory enzymes of mitochondrial beta-oxidation as targets for treatment of the metabolic syndrome. Obes Rev 11:380–8
- Shin SS, Park D, Lee HY, et al. (2012). The herbal composition GGEx18 from Laminaria japonica, Rheum palmatum, and Ephedra sinica reduces obesity via skeletal muscle AMPK and PPARα. Pharm Biol 50:506–15
- Shin SS, Yoon M. (2012). The herbal composition GGEx18 from Laminaria japonica, Rheum palmatum, and Ephedra sinica inhibits high-fat diet-induced hepatic steatosis via hepatic PPARα activation. Pharm Biol 50:1261–8
- Spiegelman BM, Flier JS. (1996). Adipogenesis and obesity: Rounding out the big picture. Cell 87:377–89
- Ukkola O, Santaniemi M. (2002). Adiponectin: A link between excess adiposity and associated comorbidities? J Mol Med 80:696–702
- Vazque M, Roglans N, Cabrero A, et al. (2001). Bezafibrate induces acyl-CoA oxidase mRNA levels and fatty acid peroxisomal beta-oxidation in rat white adipose tissue. Mol Cell Biochem 216:71–8
- Wickelgren I. (1998). Obesity: How big a problem? Science 280:1364–7
- Xue J, Ding W, Liu Y. (2010). Anti-diabetic effects of emodin involved in the activation of PPARgamma on high-fat diet-fed and low dose of streptozotocin-induced diabetic mice. Fitoterapia 81:173–7
- Yoon M. (2009). The role of PPARα in lipid metabolism and obesity: Focusing on the effects of estrogen on PPARα actions. Pharmacol Res 60:151–9
- Yoon M. (2010). PPARα in obesity: Sex difference and estrogen involvement. PPAR Res 2010:584296
- Yoon KH, Lee HY, Jung YS, et al. (2010). Modulation of obesity by Gyeongshingangjeehwan 18 in ob/ob mice. Korean J Herb 25:1–9
- Yoon M, Jeong S, Lee H, et al. (2003). Fenofibrate improves lipid metabolism and obesity in ovariectomized LDL receptor-null mice. Biochem Biophys Res Commun 302:29–34
- Yoon M, Jeong S, Nicol CJ, et al. (2002). Fenofibrate regulates obesity and lipid metabolism with sexual dimorphism. Exp Mol Med 34:481–8
- You JS, Sung MJ, Chang KJ. (2009). Evaluation of 8-week body weight control program including sea tangle (Laminaria japonica) supplementation in Korean female college students. Nutr Res Pract 3:307–14
- Zheng CD, Duan YQ, Gao JM, Ruan ZG. (2010). Screening for anti-lipase properties of 37 traditional Chinese medicinal herbs. J Chin Med Assoc 73:319–24