Abstract
Context: Tribulus terrestris L. (Zygophyllaceae) has been commonly used to energize, vitalize, and improve sexual function and physical performance in men.
Objective: This study investigates the potential cytotoxic and genotoxic, and endocrine disrupting activities of T. terrestris in vitro.
Materials and methods: The whole T. terrestris plant was extracted with water, methanol, and chloroform. The genotoxic potential of T. terrestris extracts at 3–2400 µg/mL was assessed by Comet assay in a rat kidney cell line (NRK-52E) and by Ames assay in Salmonella typhimurium TA98 and TA100 strains. Endocrine disrupting effects of the extracts at concentrations of 0.22–25 000 µg/mL were assessed by YES/YAS assay in Saccharomyces cerevisiae. Cytotoxic activity of the extracts was determined by the MTT test in NRK-52E cells. The different exposure times were used for four tests (3–48 h).
Results: The methanol extract of T. terrestris IC50 value was 160 µg/mL. The other extracts did not show cytotoxic effects. In the Comet and Ames genotoxicity assays, none of the extracts possessed genotoxic activities at concentrations of 0–2400 µg/mL. Only the water extract of T. terrestris induced frame shift mutations after metabolic activation. The water extract also showed estrogenic activity by YES/YAS assay in S. cerevisiae at concentrations ≥27 µg/mL (≥2.6-fold), while the other T. terrestris extracts had anti-estrogenic properties.
Conclusion: Tribulus terrestris had estrogenic and genotoxic activities. The study was useful in determining its toxicological effects and the precautions regarding consumption.
Introduction
Tribulus terrestris L. (Zygophyllaceae), an annual herbaceous plant, is used in the traditional medicine of India, China, Africa, Turkey, and the southern part of Europe (Angelova et al., Citation2013; Arcasoy et al., Citation1998). Tribulus terrestris is used to treat vitiligo, urological infections, prostatic hypertrophy, and edema as well as diseases of the eyes, abdomen, and cardiovascular system (Angelova et al., Citation2013; Cai et al., Citation2001). In some countries, T. terrestris has been added to herbal mixtures and drugs, which are used to treat sexual dysfunction and enhance sexual performance (Antonio et al., Citation2000; Gauthaman et al., Citation2003; Rogerson et al., Citation2007). Tribulus terrestris is extremely rich in saponins, flavonoids, alkaloids, glycosides, phytosteroids, and other constituents (Sun et al., Citation2002; Wu et al., Citation1996). Several studies have reported that the main active compounds of the plant were steroidal saponins, which act as a natural testosterone and highly active surface substances (Kostova & Dinchev, Citation2005; Tomova et al., Citation1978, Citation1981). Indeed, many pharmaceutical preparations and dietary supplements that include the saponin fraction of T. terrestris are currently on sale worldwide (Kostova & Dinchev, Citation2005; Mulinacci et al., Citation2003; Xu et al., Citation2000).
Although plants are often assumed safe, many studies have reported that various plants used as food ingredients or in traditional medicine have mutagenic (Déciga-Campos et al., Citation2007; Razak et al., Citation2007), carcinogenic (De Sá Ferreira & Vargas, Citation1999), and endocrine disrupting (Gauthaman et al., Citation2002) properties. The genotoxic properties of chemicals can be tested in vitro using bacterial and mammalian cell cultures to assess several genetic end-points as well as in vivo (Bowen et al., Citation2011; Elgorashi et al., Citation2003). To that end, the alkaline version of single cell gel electrophoresis (SCGE, Comet Assay) provides direct determinations of chemically induced single- and double-stranded DNA breaks. In addition, the short-term bacterial reverse mutation assay (Ames assay), which uses the amino acid (histidine)-dependent Salmonella typhimurium TA98 and TA100 strains, can detect base-pair substitutions and frame shift mutations (Mortelmans & Zieger, 2000; Rojas et al., Citation1999). To determine the endocrine disrupting effects of a particular substance, the recombinant Yeast Estrogen/Androgen Screen assay (YES/YAS assay) in Saccharomyces cerevisiae strains with human estrogen (hERα) and androgen (hAR) receptors is commonly used for the detection of compounds with estrogenic and androgenic agonistic and antagonistic activities (Routledge & Sumpter, Citation1996; Sohoni & Sumpter, Citation1998).
The present study determined the potential cytotoxic, genotoxic, and endocrine disrupting activities of T. terrestris in vitro. Few studies on the toxic potential of this plant are available, despite its common use (Angelova et al., Citation2013; Gauthaman et al., Citation2002). To investigate the genotoxic potential of T. terrestris extracts, we used the Comet assay in a rat kidney cell line (NRK-52E) and the Ames assay in S. typhimurium TA98 and TA100 strains. To understand the extracts endocrine disrupting effects, a YES/YAS assay in S. cerevisiae was used. The cytotoxic activity of the plant was determined by MTT test in NRK-52E cells.
Materials and methods
Chemicals
Chemicals were obtained from Sigma-Aldrich Chemicals Co. (St. Louis, MO) and Merck (Darmstadt, Germany). Phosphate buffer solution (PBS, Ca–Mg free, pH 7.2), cell culture media, and all other supplements were purchased from Wisent Bioproducts (Montreal, Canada) and sterile plastic materials were purchased from Greiner (Frickenhausen, Germany). Ames microplate fluctuation (MPF™) 98/100 and XenoScreen YES/YAS kits were purchased from Xenometrix (Allschwil, Switzerland).
Plant extractions
Tribulus terrestris L. was obtained from herbal markets in May 2013 in Istanbul and identified by Prof. Dr. Emine Akalin at the Department of Pharmaceutical Botany at Istanbul University. To ensure complete extraction of both polar and non-polar compounds, three different extractions (in water, methanol, and chloroform) were performed. Whole plant material was powdered, and 2.5 g of the plant powder was extracted in 25 mL of methanol or chloroform in a sonication bath (25 °C) for 30 min. The extracts were concentrated by rotary evaporator and dried under a gentle stream of nitrogen at 40 °C to give a solid residue. The solid residues were dissolved in 1 mL dimethylsulfoxide (DMSO), and sterilized by passing the extract through 0.45 µm filters. In assay conditions, the final condition of DMSO was 1%. For the water extraction, 2.5 g of plant powder was extracted with 25 mL of water, mixed by stirring for 30 min, and heated to 90 °C. The extracts were filter sterilized using 0.45 µm filters. The final concentrations were 2500 mg/mL for the methanol and chloroform extracts and 100 mg/mL for the water extracts. The extracts were further diluted to produce concentrations of between 3 and 2400 µg/mL for use in the genotoxicity tests. Final concentrations between 6 and 25 000 µg/mL of the methanol and chloroform extracts and between 0.22 and 1000 µg/mL of the water extracts were assessed for endocrine disrupting effects.
Cell culture
The rat kidney proximal tubular epithelial cell line (NRK-52E) was obtained from American Type Culture Collection (ATCC, Manassas, VA). The cells were grown at 37 °C in a humidified incubator with 5% CO2 in Dulbecco's Modified Eagles medium consisting of nutrient mixture F12 (DMEM/F12) containing 5.5 mmol/L d-glucose supplemented with 10% heat-inactivated fetal bovine serum (FBS) and 1% antibiotic (100 U/mL penicillin and 100 µg/mL streptomycin).
Cytotoxicity test (MTT test)
NRK-52E cells were seeded at 104 cells into each well of 96-well plates. After 24 h, the cells were exposed to 62.5–500 µg/mL of the plant extracts. After a 24 h incubation period, cytotoxicity was assessed using MTT test. Viable cells were identified by the production of an insoluble purple formazan precipitate from the reduction of 3-[4,5-dimethylthiazol-2-yl]-2,5-diphenyl-tetrazolium bromide and yellow-colored water-soluble tetrazolium salt by the mitochondrial respiratory chain enzyme, mitochondrial succinate dehydrogenase. This enzyme is only active in viable cells, and in the presence of an electron-coupling reagent (Alley et al., Citation1988; van Meerloo et al., Citation2011). The test was conducted according to the procedure put forward by Alley et al. (Citation1988). Optical densities (OD) of each well were determined at 590 nm and compared, against at a reference wavelength of 670 nm, using a microplate spectrophotometer system (Epoch, Erlangen, Germany).
PBS as the negative control and 1% DMSO as the solvent control were used for all assays. For each extract, all concentrations were tested in triplicates and each test was repeated twice. The absorbance values of samples were compared with those of the solvent controls (1% DMSO) after all values were corrected by subtracting the absorbance value of a blank (negative control). The cytotoxic activity was expressed as an IC50, the concentration of extracts that caused a 50% inhibition of enzyme activity in the cells.
Comet assay
NRK-52E cells were seeded at 5 × 105 cells per well in 6-well plates. After 24 h, the cells were treated with the extracts at concentrations of 3, 6, 30, 60, 120, and 240 µg/mL for 24 h. The exposed cells were washed with PBS, trypsinized, centrifuged at 250 g for 3 min, and re-suspended into 1 mL fresh medium. Cells were also treated with 1% DMSO as a solvent control and 25 μM hydrogen peroxide (H2O2) for 5 min on ice as a positive control under the same conditions. The viability of the cells was determined by trypan blue exclusion and cells used in the Comet assay were ≥80% viable. The Comet assay was performed by incubating cells with pre-warmed low-melting point agarose and layering this mixture on conventional microscope slides pre-coated with normal-melting point agarose and covered with a cover slip. After solidification at 4 °C, the cover slip was removed and slides were incubated for 1 h at 4 °C in a lysis solution (2.5 M NaCl, 100 mM EDTA, and 10 mM Tris-HCl, pH 10), extemporarily added with 10% DMSO and 1% triton X-100. Slides were then incubated for 20 min in fresh cold electrophoresis buffer (0.3 M NaOH, 1 mM EDTA, pH 13) at 4 °C to unravel the DNA strands, and electrophoresis was performed at 4 °C for 20 min. The slides were then washed with 0.4 M Tris-HCl neutralization buffer (pH 7.5) three times for 5 min and fixed in absolute ethanol (Speit & Hartmann, Citation1999). The DNA was stained with ethidium bromide just before examination of the slide using a fluorescent microscope (Olympus BX53, Olympus, Tokyo, Japan) at 400× magnification. Results were visualized using an automated image analysis system (Comet Assay IV, Perceptive Instruments, Suffolk, UK).
One hundred cells were scored per concentration and DNA damage to individual cells was expressed as a percentage of DNA in the comet tail (tDNA%, tail intensity). Each step was performed under indirect light. The protocol was performed in triplicate to ensure reproducibility. The data were analyzed by one-way analysis of variance (ANOVA) or Dunnett’s t-test and expressed as mean ± SE. The level of statistical significance was set at p < 0.05, and all analyses were performed using the SPSS for Windows statistical package (version 17.0, SPSS Inc., Chicago, IL).
Ames assay
The bacterial strains of S. typhimurium TA98 and TA100 were used to detect base-pair substitutions and frame shift mutations, respectively. The assay was performed according to the manufacturer’s kit protocol (Ames MPF™ 98/100, Ames microplate fluctuation).
In the Ames assay, amino acid-dependent S. typhimurium strains carry a mutation, and therefore, cannot form colonies in the absence of an external histidine source. Colony growth resumes if the mutation is reversed, thus allowing histidin to be produced by the bacteria. Spontaneous reversions can occur in each of the strains; however, mutagenic compounds cause the number of relevant colonies to increase relative to the background reversion level. The Ames MPF™ assay kit is a liquid microplate modification of the traditional Ames assay. In this modification of the assay, the catabolic activity of revertant cells decreases the pH of an incubation solution, resulting in color change from purple to yellow. The results are evaluated by counting positive (yellow) and negative (purple) wells (Flückiger-Isler & Kamber Citation2006; Umbuzeiro et al. Citation2010).
In every exposure plate, the strains (OD600 >2.0) were treated with the extracts in a culture medium (1:25 v:v) for 2 h. The exposure concentrations of the extracts were determined as 30, 60, 300, 600, 1200, and 2400 µg/mL. For metabolic activation system (S9 mix), lyophilized rat liver S9 microsomal fraction induced by Aroclor 1254 was used. S9 microsomal fraction was purchased from Xenometrix (Allschwil, Switzerland). Mutagenic potentials of the extracts were assessed in the absence and presence of the S9 mix. Thirty percent of the S9 mix was prepared immediately before combining the reagents in a sterile tube. The final concentration of the S9 mix in the assay was 4.5% v/v.
The positive controls for this assay were 2-nitrofluorene (2 μg/mL) and 4-nitroquinoline N-oxide (0.1 µg/mL) incubated without the S9 mix and 2-aminoanthracene (5 µg/mL) incubated with the S9 mix. DMSO was used as the negative control (1%). The number of positive (yellow) wells out of the total number of wells were counted in triplicate and compared with the negative control.
The criteria used to evaluate the Ames results were the fold increase in the number of positive wells over the solvent control baseline and the dose dependency. The fold increase of the revertants relative to the solvent control was determined by dividing the mean number of positive wells at each dose by those in the solvent control at baseline. The solvent control baseline was defined as the mean number of positive wells in the solvent control plus one standard deviation (SD). All solvent controls from an experiment with identical conditions (same day, same bacterial culture, solvent, and incubation conditions) were combined. An increase of ≥2-fold relative to the baseline was classified as being positive for that dose. A test sample was classified as negative when no response ≥2-fold of the baseline and no dose-dependent response were observed. To evaluate dose-dependent responses, Student’s t-test (one-sided, unpaired) was used. p Values <0.05 were considered statistically significant. Each experiment was repeated at least twice.
Recombinant yeast estrogen/androgen screen (YES/YAS) assay
Yeast strains of Saccharomyces cerevisiae genetically modified with the hERα and hAR receptors were used to assess the potential endocrine disrupting activities of the extracts. These strains also contained expression plasmids carrying the reporter gene lac-Z (encoding the enzyme β-galactosidase), which are used to measure the receptor’ activities. The strains were included in the XenoScreen YES/YAS assay kit according to the manufacturer’s protocol.
In the YES/YAS assay, ligand-bound, hERα, and hAR interact with the corresponding response elements on the lac-Z expression plasmid and modulate the transcription of the lacZ reporter gene. As a result, β-galactosidase is secreted into the medium and converts the yellow substrate chlorophenol red-β-d-galactopyranoside into a red product that is quantified colorimetrically at 570 nm. The measured OD570 correlates directly with the amount of secreted β-galactosidase and thus with the receptor-binding activity of the test substances. Plant-derived substances were tested at concentrations 6–25 000 µg/mL for methanol and chloroform extracts and between 0.22 and 1000 µg/mL for water extracts. For each sample, growth factor (G, OD690,sample/OD690,solvent control), β-galactosidase activity (relative units, U, OD570,sample/OD690,sample), and the induction ratio (IR, OD570,sample/OD570,solvent control × G) were calculated. Dose–response curves and agonist/antagonist effects were determined. If the IR of a sample was higher than the IR10, defined as 10% above the IR of solvent, the sample was considered to have an agonistic effect. Similarly, if the IR of a sample was lower than the IR90, defined as 90% of the IR of solvent, the sample was considered to have an antagonistic effect. DMSO (1%) was used as the solvent control. In determining agonistic effects of the extratcs, 17β-estradiol (10−11–10−8 M) and 5α-dihydrotestosterone (3 × 10−10−3 × 10−7 M) were used as positive controls for estrogen and androgen effects, respectively. In determining antagonistic effects of the extracts, hydroxytamoxifen (10−8–10−5 M) and flutamide (10−7–10−4 M) were used as positive controls for anti-estrogenic and anti-androgenic effects, respectively. 17β-Estradiol (1 × 10−9 M) and 5α-dihydrotestosterone (3 × 10−8 M) were added as natural ligands to the medium. Also, it was determined if the extracts inhibit the color change induced by the natural ligands. Each experiment was repeated at least twice.
Results and discussion
The present study was undertaken to determine safety of T. terrestris, commonly used as an aphrodisiac, on mutagenicity, DNA damage, and disruption of endocrine signaling in vitro.
Cytotoxicity was determined by MTT assay in NRK-52E cells exposed to plant extracts for 24 h. The methanol extracts of T. terrestris reduced cell viability in a concentration-dependent manner. The cell viabilities after exposure to 62.5, 125, 250, and 500 µg/mL of the methanol extracts were recorded as being 68.5, 62.6, 28.0, and 8.2% of control, respectively. The IC50 value was 160 µg/mL. Cytotoxic effects were not observed upon exposure to the water and chloroform extracts within the studied concentration range. The minimum cell viability after exposure to the chloroform extracts was 69.8% at 250 µg/mL (). Based on these data, we determined the genotoxic effects of extracts by Comet assay in NRK-52E cells using a selected concentration range of up to 240 µg/mL.
Table 1. Cytotoxic activity of the T. terrestris L. extracts on NRK-52E cell.
Our results obtained from the cytotoxicity studies were in agreement with data previously published by other researchers. Ali et al. (Citation2001) measured the cytotoxic activity of an ethanol extract of T. terrestris by assessing neutral red uptake in FL-cells (human amniotic epithelial cell line). They reported an IC50 value for this extract was 22 µg/mL. Angelova et al. (Citation2013) analyzed the effects of a total extract and a saponin fraction of T. terrestris on cell viability and apoptotic activity in human breast cancer (MCF7) and normal (MCF10A) cell lines. They found that the total plant extract had a marked dose-dependent inhibitory effect on the viability of breast cancer cells and the saponin fraction had an increased inhibitory effect compared with the total extract (IC50: 15 µg/mL). Similarly, Neychev et al. (Citation2007) found that a saponin fraction from T. terrestris is less toxic to normal human fibroblasts than to many cancer lines. Sun et al. (Citation2003, Citation2004) determined that saponins isolated from the ethanol extracts T. terrestris inhibit growth of Bcap-37 breast cancer cell line and exerts a cytotoxic effect on BEL-7402 cells by inducing apoptosis. Wang et al. (Citation2009) reported that steroidal saponins isolated from the fruits of T. terrestris showed potential anti-tumor activity on NCI-H460, SF-268, MCF-7, and HepG2 tumor cells.
The genotoxic effects of plants are commonly tested using bacterial and mammalian cultured cells, with several genetic end-points considered (Maron & Ames, Citation1983). In the present study, the genotoxic potential of T. terrestris was characterized by two separate in vitro assays. The results obtained from the Comet assay showed that DNA damage was not induced in NRK-52E cells exposed to extracts of T. terrestris for 24 h (). Significant differences were not found in tail intensity in NRK-52E cells treated with the plant extracts at 3, 6, 30, 60, 120, and 240 µg/mL compared with the control group (p > 0.05). Mean tail intensity was 1.76–11.43 in cells exposed to the highest concentration of T. terrestris extracts, which was approximately 5.51-fold and less than the positive control (25 μM H2O2) (tDNA%: 62.24) (). Among three different extractions, the methanol extracts had the highest genotoxic activities. The methanol and chloroform extracts of T. terrestris showed no evidence of mutagenic activity against S. typhimurium TA98 and TA100 at concentrations of 30, 60, 300, 600, 1200, and 2400 µg/mL in the Ames assay. However, the water extracts of T. terrestris at the concentrations studied were mutagenic to the TA100 strain when metabolically activated (≥2.11-fold) and induced frame shift mutations (). Our study is the first comprehensive examination of the genotoxic potential of T. terrestris. Trouillas et al. (Citation2005) reported that the saponins, structurally similar to diosgenin, present in T. terrestris extracts, might block the cell cycle, suppress proliferation, and induce apoptosis in human sarcoma cell lines. Kim et al. (Citation2011) reported that the water extracts of T. terrestris blocked proliferation and induced apoptosis in human liver cancer cells and could be used as an anticancer drug for hepatocellular carcinoma patients.
Table 2. DNA migration in the Comet assay for the assessment of genotoxicity of T. terrestris L. extracts to NRK-52 cell.
Table 3. Mutagenic potential of T. terrestris L. extracts with Ames assay in S. typhimurium TA100 and TA98 strains.
The present study also investigated the endocrine disrupting effect of T. terrestris using the recombinant YES/YAS assay. We found that the water extracts of T. terrestris showed estrogenic activity at concentrations higher than 27 µg/mL (≥2.6-fold) and none of the extracts had androgenic activity (). However, the water extracts of T. terrestris had anti-androgenic activity (). In addition, the methanol and chloroform extracts of T. terrestris possessed anti-estrogenic activity. These extracts inhibited 17β-estradiol activity at concentrations higher than 675 µg/mL. These results are supported by those of Routledge and Sumpter (Citation1996), which indicated that the YES assay showed high degree of specificity for estrogenic compounds in contrast to the YAS assay.
Figure 1. Activity of T. terrestris L. extracts in the recombinant estrogen and androgen agonist screens. The results are shown in two panels; “a” and “b” for YES and YAS assays, respectively.
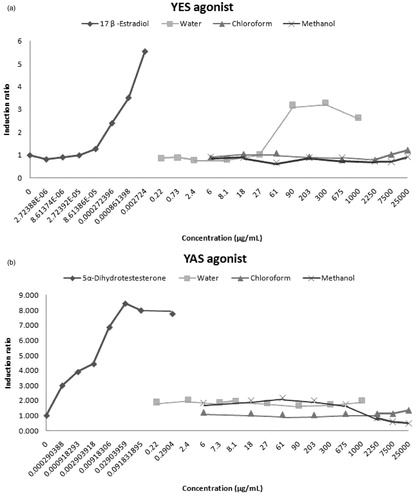
Figure 2. Activity of T. terrestris L. extracts in the recombinant anti-estrogen and anti-androgen agonist screens. The results were shown in two panels; “a” and “b” for YES and YAS assays, respectively.
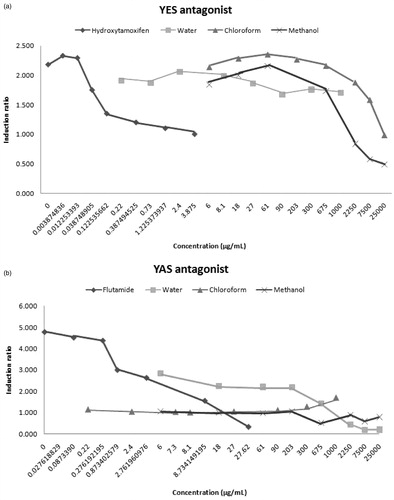
Studies from other laboratories have previously indicated the endocrine disrupting potential of T. terrestris, particularly, the water, methanol, and ethanol extracts of T. terrestris. Hussain et al. (Citation2009) showed that methanol extracts of T. terrestris increased free serum testosterone levels in male mice. Gauthaman et al. (Citation2003) reported that the pro-erectile, aphrodisiac property of the water extracts of T. terrestris might result from an increase in androgen production and a subsequent release of nitric oxide from the nerve endings innervating the corpus cavernosum. Similarly, Tomova et al. (Citation1981) showed that administration of T. terrestris to humans and animals improved libido and spermatogenesis. Sebata et al. (Citation2005) reported that supplementing sheep basal diets with T. terrestris allowed the animals to retain a positive nitrogen balance. Because T. terrestris is effective for anyone seeking rapid gains in muscle size, strength, and power, athletes involved in strength- and power-based sports, such as bodybuilders and regular gym users, are likely to notice significant gains in both muscular size and performance. In humans, this plant has been used to increase testosterone levels and improve muscle size (Adaikan et al., Citation2000; Adimoelja & Adaikan, Citation1997; Gauthaman et al., Citation2002). Conversely, Antonia et al. (Citation2000), in their study examining the effects of the herbal preparation on body composition and exercise performance in resistance-trained men, claimed that supplementation with T. terrestris did not enhance body composition or exercise performance. Similarly, Rogerson et al. (Citation2007) found that 5 weeks of supplementation with T. terrestris did not have any significant effect on strength, body mass, or fat free mass in elite rugby league players. Adimoelja et al. (Citation1997) indicated that the compound protodioscin from T. terrestris could act as a precursor of dehydroepiandrosterone, necessary for spermatozoa maturation in the epididymis, in patients with low serum levels of this hormone.
Conclusion
Contrary to popular belief that “natural are safe”, herbs can cause significant toxic effects, drug interaction, and even morbidity or mortality. However, data obtained from in vitro, in vivo, and human studies, which are used as the basis for risk characterization and the establishment of safe intakes, are limited. Data obtained from the present study may provide useful information on the toxic potential of T. terrestris. It was concluded that T. terrestris extracts did not exhibit genotoxic effects on DNA on NRK-52E cells while the water extract of T. terrestris might induce frame shift mutations when metabolically activated. In contrast, T. terrestris should be carefully used because of its endocrine disrupting potential.
Declaration of interest
This work was supported by the Research Fund of Istanbul University 37264/33751.
References
- Adaikan PG, Gauthaman K, Prasad RN, Ng SC. (2000). Proerectile pharmacological effects of Tribulus terrestris on the rabbit corpus cavernosum. Ann Acad Med Singapore 29:22–6
- Adimoelja A, Adaikan PG. (1997). Protodioscin from herbal plant Tribulus terrestris L. improves male sexual functions possibly via DHEA. Int J Impotence Res 9:64–9
- Ali NAA, Julich WD, Kusnick C, Lindequist U. (2001). Screening of Yemeni medicinal plants for antibacterial and cytotoxic activities. J Ethnopharmacol 74:173–9
- Alley MC, Scudiero DA, Monks A, et al. (1988). Feasibility of drug screening with panels of human tumor cell lines using a microculture tetrazolium assay. Cancer Res 48:589–601
- Angelova S, Gospodinova Z, Krasteva M, et al. (2013). Antitumor activity of Bulgarian herb Tribulus terrestris L. on human breast cancer cells. J BioSci Biotech 2:25–32
- Antonio J, Uelmen J, Rodriguez R, Earnest C. (2000). The effects of Tribulus terrestris on body composition and exercise performance in resistance-trained males. Int J Sport Nutr Exerc Metab 10:208–15
- Arcasoy HB, Erenmemisoglu A, Tekol Y, et al. (1998). Effect of Tribulus terrestris L. saponin mixture on some smooth muscle preparations: A preliminary study. Boll Chim Farm 137:473–5
- Bowen DE, Whitwell JH, Lillford L, et al. (2011). Evaluation of a multi-endpoint assay in rats, combining the bone-marrow micronucleus test, the Comet assay and the flow-cytometric peripheral blood micronucleus test. Mutat Res 722:7–19
- Cai L, Wu Y, Zhang J, et al. (2001). Steroidal saponins from Tribulus terrestris. Planta Med 67:196–8
- De Sá Ferreira ICF, Vargas VMF. (1999). Mutagenicity of medicinal plant extracts in Salmonella/microsome assay. Phytotherapy Res 13:397–400
- Déciga-Campos M, Rivero-Cruz I, Arriaga-Alba M, et al. (2007). Acute toxicity and mutagenic activity of Mexican plants used in traditional medicine. J Ethnopharmacol 110:334–42
- Elgorashi EE, Taylor JLS, Maes A, et al. (2003). Screening of medicinal plants used in South African traditional medicine for genotoxic effects. Toxicol Lett 143:195–207
- Flückiger-Isler S, Kamber M. (2006). The Ames MPF™ 98/100 Assay: Novel Mutagenicity Testing in Liquid Microplate Format Using S. typhimurium TA98 and TA100. Prague: EEMS
- Gauthaman K, Adaikan PG, Prasad RNV. (2002). Aphrodisiac properties of Tribulus terrestris extract (Protodioscin) in normal and castrated rats. Life Sci 71:1385–96
- Gauthaman K, Ganesan AP, Prasad RN. (2003). Sexual effects of puncture vine (Tribulus terrestris) extract (protodioscin): An evaluation using a rat model. J Altern Complement Med 9:257–65
- Hussain AA, Abbas AM, Heba HI, Amir HA. (2009). Study the biological activities of Tribulus terrestris extracts. WASET 57:433–5
- Kim HJ, Kim JC, Min JS, et al. (2011). Aqueous extract of Tribulus terrestris Linn induces cell growth arrest and apoptosis by down regulating NF-κB signaling in liver cancer cells. J Ethnopharmacol 136:197–203
- Kostova I, Dinchev D. (2005). Saponins in Tribulus terrestris – Chemistry and bioactivity. Phytochem Rev 4:111–37
- Maron DM, Ames BN. (1983). Revised methods for the Salmonella mutagenicity test. Mutation Res 113:173–215
- Mortelmans K, Zeiger E. (2000). The Ames Salmonella/microsome mutagenicity assay. Mutation Res 455:29–60
- Mulinacci N, Vignolini P, La Marca G, et al. (2003). Food supplements of Tribulus terrestris L. Chromatographia 57:581–92
- Neychev VK, Nicolova E, Zhelev N, Mitev VI. (2007). Saponins from Tribulus terrestris L. are less toxic for normal human fibroblasts than for many cancer lines: Influence on apoptosis and proliferation. Exp Biol Med 232:126–33
- Razak MFA, Aidoo KE, Candlish AGG. (2007). Mutagenic and cytotoxic properties of three herbal plants from Southeast Asia. Trop Biomed 24:49–59
- Rogerson S, Riches CJ, Jennings C, et al. (2007). The effect of five weeks of Tribulus terrestris supplementation on muscle strength and body composition during preseason training in Elite Rugby League Players. J Strength Cond Res 21:348–53
- Rojas E, Lopez MC, Valverde M. (1999). Single cell gel electrophoresis assay: Methodology and applications. J Chromat B 722:225–54
- Routledge EJ, Sumpter JP. (1996). Estrogenic activity of surfactants and some of their degradation products assessed using a recombinant yeast screen. Environ Toxicol Chem 15:241–8
- Sebata A, Ngongoni NT, Mupangwa JF, et al. (2005). Effects of supplementing native pasture hay with puncture vine Tribullus terrestris on the intake, weight change, nitrogen balance and excretion of purine derivatives of sheep. Trop Subtrop Agroecosyst 5:123–8
- Sohoni P, Sumpter JP. (1998). Several environmental oestrogens are also anti-androgens. J Endocrinol 158:327–39
- Speit G, Hartmann A. (1999). The comet assay (single-cell gel test): A sensitive genotoxicity test for the detection of DNA damage and repair. DNA Repair Protocols 113:203–12
- Sun B, Qu WJ, Zhang XL. (2004). Ivestigation on inhibitory and apoptosis-inducing effects of saponins from Tribulus terrestris on hepatoma cell line BEL-7402. Zhongguo Zhong Yao Za Zhi 29:681–4
- Sun WJ, Gao J, Tu GZ, et al. (2002). A new steroidal saponin from Tribulus terrestris Linn. Nat Prod Lett 16:243–7
- Sun B, Qu W, Bai Z. (2003). The inhibitory effect of saponins from Tribulus terrestris on Bcap-37 breast cancer cell line in vitro. Zhong Yao Cai 26:104–6
- Tomova M, Gyulemetova R, Zarkova S. (1978). An agent for stimulation of sexual function. Bulg Patent (11) 27584, A61K35/1978
- Tomova M, Gyulemetova R, Zarkova S, et al. (1981). Steroidal saponins from Tribulus terrestris L. with a stimulating action on the sexual functions. In: Proceedings of the First International Conference on Chemistry and. Biotechnology of Biological Active Natural Products, Varna, September 3:289–91
- Trouillas P, Corbière C, Liagre B, et al. (2005). Structure–function relationship for saponin effects on cell cycle arrest and apoptosis in the human 1547 osteosarcoma cells: A molecular modelling approach of natural molecules structurally close to diosgenin. Bioorg Med Chem 13:1141–9
- Umbuzeiro GDA, Rech CM, Correia S, et al. (2010). Comparison of the Salmonella/microsome microsuspension assay with the new microplate fluctuation (MPF) protocol for testing the mutagenicity of environmental samples. Environ Mol Mutagen 51:31–8
- Van Meerloo J, Kaspers GJ, Cloos J. (2011). Cell sensitivity assays: The MTT assay. Methods Mol Biol 731:237–45
- Wang J, Zu X, Jiang Y. (2009). Five furostanol saponins from fruits of Tribulus terrestris and their cytotoxic activities. Nat Prod Res 23:1436–44
- Wu G, Jiang S, Jiang F, et al. (1996). Steroidal glycosides from Tribulus terrestris. Phytochemistry 42:1677–81
- Xu YX, Chen HS, Liang HQ, et al. (2000). Three new saponins from Tribulus terrestris. Planta Med 66:545–50