Abstract
Context: Ixeris gracilis DC. Stebbins (Asteraceae) is a plant considered to be medicinal by local communities of Meghalaya, India.
Objective: To evaluate the antidiabetic potential, antioxidant activity, and effect of the 80% methanolic extract of the leaves of Ixeris gracilis on tumor necrosis factor-α (TNF-α) expression.
Materials and methods: Varying doses (250–1000 mg/kg body weight) were administered intraperitoneally to normoglycemic mice and their hypoglycemic properties noted for 24 h; the optimum dose observed was used to evaluate its antihyperglycemic activity and effect on glucose tolerance. In vitro antioxidant activity was analyzed by assessing the DPPH radicals scavenging ability of the extract and the total polyphenols, flavonoid, carbohydrate, and protein contents were determined. Diabetic mice were then subjected to daily intraperitoneal injections of the extract for 12 days after which the antioxidant enzyme activities in the tissues were assayed and serum TNF-α was evaluated by ELISA.
Results: The extract displayed varying hypoglycemic activity. The dose of 250 mg/kg body weight exhibited potent antihyperglycemic activity and improved glucose tolerance. The extract was able to scavenge free radicals (IC50 57.544 µg/ml) and contained polyphenol (76.269 ± 0.204 mg GAE/g dry wt), flavonoid (70.070 ± 0.626 mg rutin equivalent/g dry wt), protein (4.368 ± 8.916 mg/g dry wt), and carbohydrate (558.189 ± 0.002 mg/g dry wt). TNF-α level and overall activity of glutathione peroxidase and superoxide dismutase in the liver, kidney, and brain of extract-treated diabetic mice were improved.
Conclusion: The study supports the inclusion of Ixeris gracilis in the list of plants with antidiabetic potential.
Introduction
Type 2 diabetes is a metabolic disorder characterized by hyperglycemia, insufficiency of secretion or action of endogenous insulin, and inflammation (Donath & Shoelson, Citation2011; Maritim et al., Citation2003). This disease is rapidly increasing, with the number of diabetics worldwide reaching epidemic proportions (Wild et al., Citation2004). More than 60% of the world's diabetic population is contributed by Asian countries (Ramachandran et al., Citation2012) and these populations tend to develop diabetes at younger ages (Hu, Citation2011). This could greatly impact a nation's health and economy, particularly if it is a developing country (Ramachandran et al., Citation2010). Currently, oral therapeutic agents like sulfonylureas, biguanides, and α-glucosidase inhibitors are effective as antidiabetic agents (Codario, Citation2005). The use of these drugs is, however, restricted by factors such as secondary failure rates especially with increasing therapy duration and unwanted side effects (Bailey & Day, Citation1989; Bocuzzi et al., Citation2001). In spite of developments in drug discovery, traditional medicine is still widely used for the treatment of ailments such as diabetes (Al-Aboudi & Afifi, Citation2011) and the search for more effective yet safer therapeutics to manage the disease continues. Moreover, over the years, natural products and their derivatives have provided leads for developing new drugs, especially on an average they are more readily absorbed than synthetic drugs (Harvey, Citation2008).
Many plants have been reported to possess antihyperglycemic, hypoglycemic, or antioxidant properties (Eidi et al., Citation2006; Marles & Farnsworth, Citation1995; Olaokun et al., Citation2013; Panda et al., Citation2010), including plants from the North Eastern parts of India such as Potentilla fulgens L. (Rosaceae) Osbeckia chinensis L. (Melastomaceae), and Smilax perfoliata Lour. (Smilacaceae) (Syiem & Khup, Citation2006; Syiem & Warjri, Citation2013; Syiem et al., Citation2002). Plants are also rich sources of antioxidants, especially polyphenols and flavonoids (Chis et al., Citation2009; Egert & Rimbach, Citation2011; Manach et al., Citation2004; Morales et al., Citation2014). In fact, polyphenols are the major antioxidants found in plants, while flavonoids are the most abundant polyphenols in the diet (Bouayed & Bohn, Citation2012; Scalbert & Williamson, Citation2000). Flavonoids are polyphenols which have a common structure consisting of two aromatic rings linked together by three carbon atoms that form an oxygenated heterocycle (Bouayed & Bohn, Citation2012). It is an accepted fact that polyphenols and other antioxidants scavenge free radicals and exert a protective effect on the cell. With time, however, this has become an over generalized view of their mode of action as it is more likely that cells respond to polyphenols through direct interactions with receptors or enzymes involved in signal transduction, which may result in modification of the redox status of the cell triggering a series of redox-dependent reactions (Scalbert et al., Citation2005). There may be more to these antioxidants than what was previously thought. Increasing evidence show that polyphenols such as flavonoids possess several physiological properties such as antioxidant, antibacterial, antiviral, anti-inflammatory, antidiabetic, antimutagenic, and antitumoral activity, as well as the activation or inactivation of certain enzymes (Chis et al., Citation2009; Pandey & Rizvi, Citation2009).
A well-accepted fact is that a balance between oxidation and autoxidation is important for a healthy biological system (Bouayed & Bohn, Citation2010). To prevent the body from being overcome by the daily oxidative stress, cells have developed a very efficient system of defense derived from sources both endogenous and exogenous. Under normal conditions, antioxidant defense systems such as glutathione peroxidase (GPx) and superoxide dismutase (SOD) protect the cell from the damage induced by free radicals (Sadi & Guray, Citation2009). SOD converts intracellular superoxide radicals into hydrogen peroxide which are cleared by GPx and catalase further by converting it into water. In the diabetic state, however, these systems are compromised. It is known that type 2 diabetes mellitus patients are subjected to chronic oxidative stress (Robertson et al., Citation2004). Diabetes is usually accompanied by increased production of free radicals or impaired antioxidant defenses (Maritim et al., Citation2003). The high concentration of free radicals damages cellular components such as lipids, nucleic acids, and proteins (Campos et al., Citation2013). Moreover, it has been postulated that type 2 diabetes mellitus may represent a disease of the innate immune system responsible for an ongoing cytokine-mediated acute phase response with increased levels of IL-6 and tumor necrosis factor-α (TNF-α) (Esposito et al., Citation2002).
In the quest for antidiabetic agents, therefore, we decided to screen for plants with both antidiabetic and antioxidant activities, since there is clearly a close connection between oxidative stress and diabetic status. Ixeris gracilis DC. Stebbins is found growing in the East Khasi Hills district of Meghalaya, India. It belongs to the family Asteraceae, which is reported to be rich in plants with hypoglycemic potential (Erasto et al., Citation2005; Patel et al., Citation2012). Locally known as “Khmut sim” (Kayang, Citation2007), it is believed to have medicinal properties by the local Khasi population of Meghalaya, and is used in traditional medicine to alleviate many disorders such as flatulence and in the management of blood sugar levels. There has been, however, no proper scientific validation of properties or constituents. The ability of the aqueous extract to lower blood glucose in diabetic mice was reported by Syiem and Warjri (Citation2011). The present study further investigates the healing and protective properties of this traditionally used plant by checking its antioxidant potential and its ability to lower the expression levels of the inflammatory cytokine, TNF-α, since perturbations of TNF-α metabolism may affect the onset and progression of type 2 diabetes (Swaroop et al., Citation2012). Furthermore, since 80% methanol may be a better solvent than water for antioxidants like polyphenols and flavonoids (Senapati et.al, Citation2013), it was taken as the solvent of choice.
Additionally, the antioxidant effect of the extract has been evaluated by conducting both in vitro and in vivo assays. While screening for antioxidants, it is practical to first perform in vitro assays, since they are relatively easier to perform and prevent the unnecessary use of laboratory animals (Hermans et al., Citation2007; Kniewald et al., Citation2005). The free radical 1,1-diphenyl-2-picryl-hydrazyl or DPPH was used in order to assess the free radical scavenging ability of the extract. DPPH is classified as a “stable” free radical due to the delocalisation of the spare electron over the molecule as a whole, so that the molecules do not dimerise, unlike most other free radicals (Molyneux, Citation2004). The effect of antioxidants on DPPH radical scavenging may be attributed to their hydrogen-donating ability (Brighente et al., Citation2007). The DPPH method has been widely used to investigate the ability of plant extracts to act as free radical scavengers (Mayakrishnan et al., Citation2013).
Materials and methods
Chemicals
Alloxan monohydrate, 1,1-diphenyl-2-picryl-hydrazyl (DPPH), 5,5-dithio bis-2-nitro benzoic acid (DTNB), and reduced glutathione were procured from Sigma Chem. Co., St. Louis, MO. ELISA kit for TNF-α expression determination was procured from R and D Systems (Minneapolis, MN), glucometer and glucostix used were from SD Check, Kolkata, West Bengal India. Glibenclamide was obtained from Aventis Pharma Ltd. Chennai, India, insulin from Torrent Pharmaceuticals Ltd., Chennai, Tamil Nadu, metformin from USV Ltd., Mumbai, India, sodium azide, diethylenetriaminepentaaceticacid (DETAPAC), sodium lauryl sulfate, and bovine serum albumin were purchased from Sisco Research Laboratories (SRL, Mumbai, India) while other chemicals used were of analytical grade.
Test animals
Healthy, adult female Swiss albino mice weighing 20–30 g were used for the study. Mice were housed in a room kept under controlled conditions maintained at a temperature of 22 °C on a 12 h light/dark cycle. The mice were fed with balanced mice feed obtained from Pranav Agro Ltd., New Delhi, India. All experiments were performed following Institutional ethical guidelines.
Preparation of diabetic mice
Diabetes was induced by the administration of alloxan monohydrate to mice according to Syiem et al. (Citation2002). Blood glucose level was checked and mice with two- to three-fold elevations in their blood sugar levels were considered diabetic and used for further tests.
Plant material
Leaves of Ixeris gracilis were collected during the months of April 2011–June 2012 from the fields of Smit village of East Khasi Hills District of Meghalaya (Voucher no. NEHU-11875). The specimen was submitted and identified by Dr. P. B. Gurung, the Curator, Herbarium, Department of Botany, NEHU, Shillong, Meghalaya.
Plant extraction
The plant extraction protocol used was that originally given by Harborne (Citation1998). Leaves of I. gracilis were first separated, weighed, washed, and then dried in the shade. The dried leaves were finely powdered, following which the powdered mass was weighed and extracted with 10 times the volume of solvent (methanol:water, 4:1) for 24 h. The mixture was filtered and the filtrate evaporated to dryness in a Hetolyolab 3000 lyophilyzer (Heto-Holten, Allerod, Denmark). The dried mass obtained was transferred into air-tight containers used for the required studies.
Preparation of extracts for use in experiments
Prior to use, the appropriate amount of extract was calculated and weighed. The extract was dissolved in methanol for in vitro experiments. For administration to mice, extracts were dissolved in 2% ethanol.
Administration of extract to normal mice for hypoglycemic studies
Mice were divided into six test groups and one control group, each group comprising a minimum of six mice (n = 6). The mice were fasted overnight prior to performing the experiment. The control group received only water. The crude extract in varying doses ranging from 250 to 1000 mg/kg body weight (b.w.) was administered to test groups by intraperitoneal (i.p.) injection and the blood glucose level was monitored at different time intervals following the extract administration. 2% ethanol was the solvent used to dissolve the dried extracts and hence one group received 2% ethanol. Food but not water was withheld during the 6 h experiment period. After determination of blood glucose level at 6 h, food was given to the mice and the blood glucose level was monitored again at 24 h.
Administration of extract to alloxan-induced diabetic mice
Alloxan-induced diabetic mice were administered (i.p.) a single dose of 250 mg/kg b.w. of the plant extract. This was the optimum dose determined in the normoglycemic studies. The blood glucose level was measured at varying time intervals for 24 h.
Oral glucose tolerance test
The oral glucose tolerance test and collection of blood for determination of blood glucose level were performed as described by Syiem et al. (Citation2002) with slight modifications, in which the glucose load given was 3000 mg/kg b.w. The standard reference drugs used in the present study were glibenclamide, metformin, and the hormone insulin.
Assays for total polyphenol, flavonoid, protein, and carbohydrate contents of plant extract
Total polyphenol content in the plant extract was assayed using the Folin–Ciocalteu reagent according to Singleton et al. (Citation1999) with slight modifications. Flavonoid estimation was performed according to the protocol of Lamaison and Carnet (Citation1990). Carbohydrates were estimated using anthrone reagent while Bradford's method was employed for the quantification of proteins (Bradford, Citation1976; Yemm & Willlis, Citation1954). Total polyphenols were expressed as milligram gallic acid equivalents per gram dry weight (mg GAE/g dry weight), flavonoids as milligram rutin equivalents per gram dry weight (mg rutin equivalents/g dry weight), and proteins and carbohydrates as milligrams per gram dry weight (mg/g dry weight).
Free radical scavenging activity of plant extract
Free radical scavenging activity of the plant extract was determined by the capability of the extract to scavenge the DPPH radical, according to the method of Brand-Williams et al. (Citation1995). In brief, various concentrations of the plant extract were taken and made to react with DPPH radicals in methanol. The mixture was mixed and incubated for 30 min at room temperature. The absorbance of the test samples and control were then recorded at λ = 517 nm. The IC50 for each extract, which corresponds to amount of antioxidants necessary to decrease the initial DPPH absorbance by 50%, was calculated.
Treatment of mice for in vivo antioxidant activity studies
In vivo antioxidant activity studies were carried out to test the efficacy of the extracts on diabetic mice. Various groups of mice were administered 2% ethanol, the extract, drug (glibenclamide) via daily intraperitoneal injections (i.p.) for a period of 12 d, which represents an early or sub-acute stage (Attele et al., Citation2002; Chen et al., Citation1998) of diabetes. Mice were grouped as shown in .
Table 1. Grouping of normoglyclemic mice for sub-acute (12 days) studies.
After the treatment period, blood was collected by retro orbital sinus puncture and the serum was used for assaying TNF-α expression. The mice were sacrificed by cervical dislocation under local anesthesia and tissues (liver, kidney, and brain) used for in vivo enzyme assays.
In vivo assays for antioxidant enzymes
Tissues (liver, kidney, and brain) were weighed and homogenized (10% w/v) in 10 mM potassium phosphate buffer, pH 7.0, and centrifuged at 18 000 × g for 15 min at 4 °C. The pellet was discarded and the supernatant was used for enzyme assays. Protein concentrations of the tissue homogenates were determined according to the method of Bradford (Citation1976); using bovine serum albumin (BSA) as the standard.
GPx was assayed by measuring the amount of reduced glutathione (GSH) at λ = 412 nm according to the method of Rotruck et al. (Citation1973). The activity of GPx is expressed as units/mg protein, in which 1 unit corresponds to μmoles of GSH utilized/min.
SOD activity was assayed by the method of Marklund and Marklund (Citation1974). The amount of inhibition of autoxidation of pyrogallol by SOD was used as a measure of enzyme activity by measuring the change in absorbance at λ = 470 nm. Enzyme activity is expressed as units/mg protein, with 1 unit defined as the amount of enzyme required for 50% inhibition of pyrogallol autoxidation/min.
Detection of TNF-α expression level
TNF-α expression level in serum of all groups of mice under study was performed by enzyme-linked immunosorbent assay using an ELISA kit for TNF-α detection. Results were expressed as pg/ml. The manufacturer's protocol was followed.
Statistical analysis
Student's t-test was used for determining the levels of significance among the normal, diabetic control, and the diabetic-treated groups. Results are expressed as mean ± standard error of mean (SEM).
Results
Hypoglycemic activity
Normoglycemic mice were used in order to assess the ability of the 80% methanolic extract of I. gracilis in exerting a hypoglycemic effect. The extract was seen to exhibit moderate hypoglycemic activity (). When compared with other higher doses used, the dose of 250 mg/kg b.w. exhibited greatest glucose lowering potential at the time interval of 2 h following extract administration, reducing the blood glucose level by 20% as compared with the control group. 2% ethanol did not have any effect on the level of blood glucose at this time interval. At 4 and 6 h following extract administration, the effect of the extract was more apparent with the same low dose of 250 mg/kg b.w. reducing the elevated glucose level by 18 and 30%, respectively. 2% ethanol, however, seemed to cause a slight increase of blood glucose at 4 h following administration. The higher doses of the extract brought about a slight increase rather than decrease in blood glucose level at 2 h, which gradually declined over the other later time intervals. The dose of 450 mg/kg b.w. was also effective in reducing the blood sugar with a percentage reduction of 24% at 6 h after administration. Overall, it was observed that the response elicited was not dose- dependent, and that the dose of 250 mg/kg b.w. was the most effective, displaying a consistent blood glucose lowering effect on normoglycemic mice. Mice were fed after the 6th h reading and blood glucose again recorded at 24 h in the fed state. This reading showed that the 80% methanolic extract of I. gracilis exerted a prolonged effect on the blood glucose level since mice treated with the extract were found to have significantly lower levels of blood glucose than the control or 2% ethanol groups at this time interval. None of the doses studied lowered blood glucose level below 40 mg/dl and, hence, it may be stated that the hypoglycemic effect was moderately mild.
Antihyperglycemic activity
Based on the hypoglycemic studies, the dose of 250 mg/kg b.w. was exclusively used for the antihyperglycemic studies as this was the most potent dose. The extract significantly reduced blood glucose level by 31% (p < 0.05) at 4 h and by 37% (p < 0.01) at 6 h after extract administration (). Results were not significant for 2 and 24 h. Notably, 2% ethanol did not bring about any reduction in blood glucose levels at any of the time intervals studied.
Oral glucose tolerance test (OGTT)
OGTT for both normoglycemic () and diabetic () groups of mice was carried out in which the extract at the dose of 250 mg/kg b.w. was used. In addition, 2% ethanol and standard drugs were also administered to separate groups of mice as stated earlier. At 30 min following oral glucose load, a spike in the blood glucose level was observed in normoglycemic control mice and the group administered with 2% ethanol. This glucose peak was reduced (23% reduction) by the 80% methanolic extract of I. gracilis. The standard oral glycemic drugs used in the experiment could also markedly bring about this glucose peak suppression. The maximum glucose peak suppression was exhibited at 120 min after oral glucose load with mice treated with the plant extract displaying an average blood glucose reading of 106 mg/dl versus 131 mg/dl observed in the control group. The magnitude of effect on blood glucose was comparable with the drug metformin.
Figure 3. Glucose tolerance in normoglycemic mice administered with 250 mg/kg b.w. 80% methanolic extract of I. gracilis and reference drugs. Values are expressed as mean ± SEM.
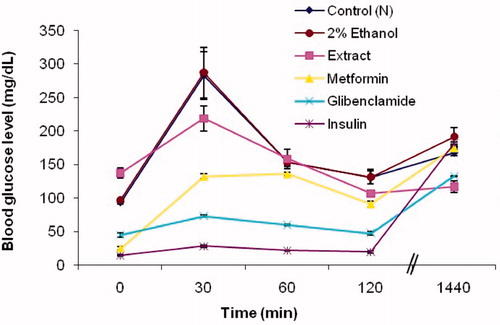
Figure 4. Glucose tolerance in alloxan-induced diabetic mice administered with 250 mg/kg b.w. About 80% methanolic extract of I. gracilis and reference drugs. Values are expressed as mean ± SEM.
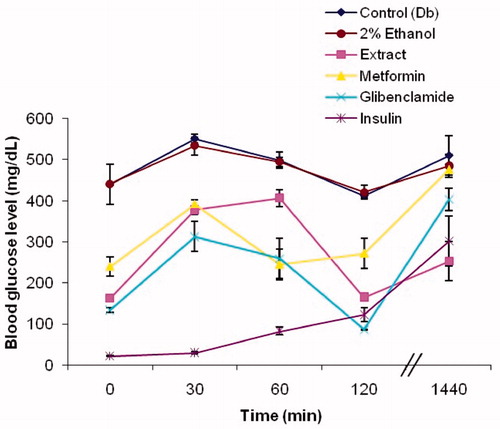
Diabetic control mice and mice treated with 2% ethanol displayed compromised glucose tolerance, as was evident from the elevated blood glucose level at 30, 60, 120, and 1440 min following oral glucose load. Diabetic mice treated with the 80% methanolic extract of I. gracilis showed improved glucose tolerance. A 32% suppression of the glucose peak at 30 min was observed, which was more than the 29% suppression seen in diabetic mice treated with metformin. Blood glucose levels were observed to be lower than the control group at 60 min and this subdual effect continued at 120 min also, where the plant extract was observed to bring blood glucose to normal levels (165 mg/dl), lower than those produced by metformin (271 mg/dl). The ability of the extract to exert a prolonged effect on blood glucose levels was observed when blood glucose levels were measured at 1440 min, i.e., when the mice were no longer in the starved condition. Blood glucose levels were observed to be 252 mg/dl, lower than even the 301 mg/dl reading displayed by insulin.
Estimations of total polyphenol, flavonoid, carbohydrate, and protein contents
Results for estimations of polyphenol, flavonoid, carbohydrate, and protein contents of methanolic extract of I. gracilis are summarized in . Polyphenol content of the extract was found to be 76.269 ± 0.204 mg GAE/g dry wt of plant extract, flavonoid was found to be 70.070 ± 0.626 mg rutin equivalent/g dry wt of plant extract, proteins were 4.368 ± 8.916 mg/g dry wt while carbohydrate concentration was 558.189 ± 0.002 mg/g dry wt.
Table 2: Total polyphenol, flavonoid, protein and carbohydrate content, and DPPH radical scavenging activity of 80% methanolic extract of I. gracilis. Results are expressed as mean ± SEM.
DPPH radical scavenging activity
The 80% methanolic extract of I. gracilis was found to be able to scavenge free radicals effectively. It exhibited an IC50 value of 57.544 µg/ml. The results are shown in .
Effects on antioxidant enzymes' activities
GPx activity
Increased GPx activity (p < 0.001) was observed () in the brain of diabetic control mice as compared with normoglycemic control mice (35.146 ± 2.69 versus 14.914 ± 1.680). The 80% methanolic extract of I. gracilis could significantly (p < 0.01) lower GPx levels in the brain tissues (12.497 ± 1.054). Levels of Gpx in the liver of diabetic mice showed no significant change. GPx activity in the kidney of diabetic mice was increased by 40%. This elevation was reduced to 5.706 ± 1.086 in extract treated mice. It was observed that in all three tissues, glibenclamide-treated mice had higher levels of GPx activity than both normal and diabetic test groups.
Figure 5. Glutathione peroxidase (GPx) activity in various tissues (liver, kidney, and brain) of normoglycemic, diabetic, 2% ethanol-treated, extract-treated, and glibenclamide-treated diabetic mice compared against their respective controls: diabetic versus normoglycemic, values are expressed as mean ± SEM (#p < 0.05, ##p < 0.01, ###p < 0.001); 2% ethanol-treated, extract-treated, and glibenclamide-treated diabetic versus the diabetic control group, values are expressed as mean ± SEM (*p < 0.05, **p < 0.01, ***p < 0.001).
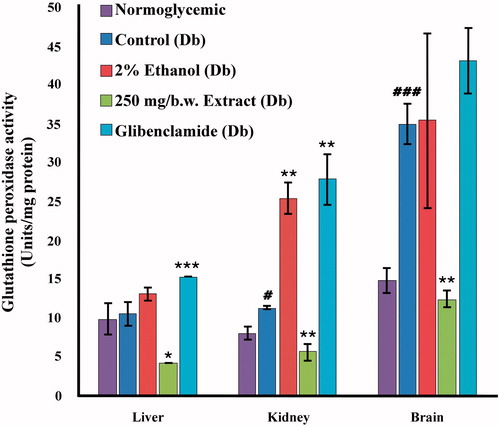
SOD activity
SOD activity was significantly (p < 0.001) elevated in the diabetic control group as compared with the normal control group () in all three tissues studied. Liver, kidney, and brain SOD levels of diabetic control mice were 6.863 ± 0.192, 11.235 ± 0.882, and 22.395 ± 0.340 versus 1.562 ± 0.028, 2.790 ± 0.069, and 6.587 ± 0.428, of normoglycemic mice, respectively. The 80% methanolic extract of I. gracilis could reduce SOD activities in the brain of diabetic mice, although levels observed in normoglycemic mice could not be attained (11.084 ± 0.646 in extract treated diabetic mice and 6.587 ± 0.428 in normal control). The extract was found to have no effect on the activities of the liver and brain enzymes. The drug glibenclamide could not bring levels of SOD activity to those observed in normoglycemic mice in any of the tissues studied, but instead further elevated brain SOD activity to 47.117 ± 1.574.
Figure 6. Superoxide dismutase (SOD) activity in various tissues of normoglycemic, diabetic, 2% ethanol-treated, extract-treated, and glibenclamide-treated diabetic mice compared against their respective controls: diabetic versus normoglycemic, values are expressed as mean ± SEM (#p < 0.05, ##p < 0.01, ###p < 0.001); 2% ethanol-treated, extract-treated, and glibenclamide-treated diabetic versus the diabetic control group, values are expressed as mean ± SEM (*p < 0.05, **p < 0.01, ***p < 0.001).
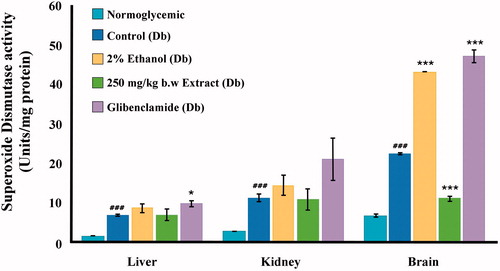
TNF-α expression levels
The levels of TNF-α in the sera of normoglycemic, diabetic, 80% methanolic extract-treated and glibenclamide-treated diabetic mice were assayed using ELISA. A 16-fold increase in the expression of the inflammatory cytokine was observed when compared with normoglycemic levels (). The 80% methanolic extract of I. gracilis exhibited a very potent ability to bring down the elevated expression of the cytokine. The levels of TNF-α in the serum of diabetic mice treated with the extract reached levels detected in normoglycemic mice (11.133 ± 1.162 in extract treated mice versus 10.667 ± 1.306 in normoglycemic mice). The 2% ethanol was also very effective in reducing TNF-α levels with a percentage reduction of 72%. The effects that 2% ethanol exerted on TNF-α expression was comparable with those produced by glibenclamide, which brought about a reduction of 70%.
Figure 7. TNF-α expression level in normoglycemic, diabetic, 2% ethanol-treated, extract-treated, and glibenclamide-treated diabetic mice compared against their respective controls: diabetic versus normoglycemic, values are expressed as mean ± SEM (#p < 0.05, ##p < 0.01, ###p < 0.001); 2% ethanol-treated, extract-treated and glibenclamide-treated diabetic versus the diabetic control group, values are expressed as mean ± SEM (*p < 0.05, **p < 0.01, ***p < 0.001).
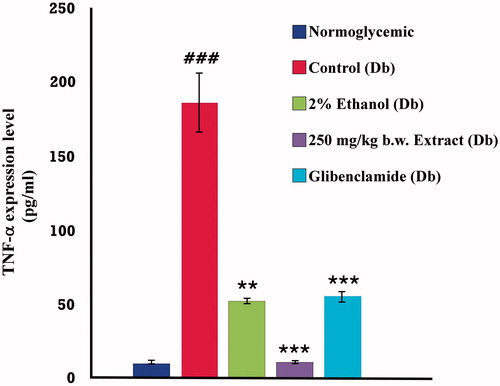
Discussion and conclusion
The studies carried out in normoglycemic and diabetic mice indicate that the 80% methanolic extract of I. gracilis possesses mild hypoglycemic activity, i.e., it could lower blood glucose to levels slightly lower than those observed in normoglycemic controls. A notable pattern was observed in which, on one hand, the lower doses elicited a time-dependent blood glucose lowering response: blood glucose levels gradually decreasing with increasing passage of time. The higher doses, on the other hand, were seen to bring about an increase in blood glucose levels at 2 h following extract administration. This may be indicative of the presence of other entities in the 80% methanolic extract that counter the hypoglycemic effects at high dosage. Results show that the 80% methanolic extract of I. gracilis at 250 mg/kg b.w. was effective in reducing blood glucose of diabetic mice at 6 h following extract administration. This was further confirmed by the glucose tolerance test which is the “Gold standard” in the diagnosis of diabetes (Olabi & Bhopal, Citation2009). OGTT in diabetic mice showed that although the extract was not as effective as insulin, its ability to reduce the glucose peak at 30 min was comparable with metformin. It was also seen to be more effective than metformin in reducing blood glucose levels at 120 min following glucose load. From these experiments, it was seen that this extract was effective in improving glucose tolerance and seemed to resemble glibenclamide with regard to the pattern of glucose lowering, but was more similar to metformin with respect to the extent of modulation. Plants can act either as insulin mimics or insulin sensitizing agents. They also act on the glucose transporters or promote glucose uptake by cells (Cetto et al., Citation2000; Gray & Flatt, Citation1999; Zhang & Tan, Citation2000). Although similarities exist between the 80% methanolic extract of I. gracilis and metformin on the extent of blood glucose lowering effects, a comparable pattern to glibenclamide was also apparent. The plant I. gracilis may, therefore, behave in a similar manner as plants which possess insulin secretagogue compounds such as Kalanchoe pinnata Lam. (Crassulaceae), Momordica charantia L. (Cucurbitaceae), and Trigonella foenum graecum L. (Fabaceae) (Patil et al., Citation2013; Shah et al., Citation2006; Yibchok-Anun et al., Citation2006).
The presence of the antioxidants flavonoids and other polyphenols in the 80% methanolic extract of I. gracilis was observed and is probably the reason why the free radical DPPH was effectively scavenged. Flavonoids and other polyphenols have powerful antioxidant activities in vitro, being able to scavenge a wide range of reactive species (Halliwel, Citation2007). Furthermore, the extract's effects on the antioxidant enzymes GPx and SOD clearly indicate that the plant extract could improve the antioxidant status of the cell and reduce oxidative stress. Oxidative stress is widely accepted as playing a key mediatory role in the development and advancement of diabetes and its complications, due to the increase in production of free radicals and impaired antioxidant defenses (Ceretta et al., Citation2012). According to Ceriello et al. (Citation1996), high glucose levels bring about an increase in the production of free radicals which then induce antioxidant enzyme expression in some tissues. Elevations in blood glucose levels have also been stated as the cause for an increase in the TNF-α concentration which then, together with glucose, bring about an inhibition of insulin receptor signalling (Kroder et al., Citation1996). Moreover, the drugs thiazolidinediones (TZDs) such as pioglitazone which are used for treating diabetes are reported to reduce circulating TNF-α levels (Tonelli et al., Citation2004). The ability of the 80% methanolic extract of I. gracilis to bring down TNF-α expression and to lower the elevated GPx and SOD in diabetic mice may, therefore, be because of its blood glucose-reducing properties and the presence of polyphenols and flavonoids, which may have helped in decreasing the amount of free radicals thereby restoring the antioxidant enzymes to normal or near-normal levels. It may be pointed out that this is largely speculative at this point, although all results strongly point in this direction.
It is noteworthy, however, that there has been much debate over the benefits of dietary polyphenols. They are known to alter levels of antioxidant enzymes, but much depends on their bioavailability (Halliwel, Citation2007; Scalbert & Williamson, Citation2000). According to Scalbert and Williamson (Citation2000), the chemical structure of polyphenols determines their rate and extent of intestinal absorption and the nature of the metabolites circulating in the plasma, with the few bioavailability studies in humans showing that the quantities of polyphenols found intact in urine vary from one phenolic compound to another. It becomes imperative, therefore, to state that in vitro studies have to be supported by in vivo studies as well, in order to ascertain the antioxidant capacity of a particular plant extract. The in vivo antioxidant enzyme activities and serum TNF-α expression support the in vitro findings that the 80% methanolic extract of I. gracilis possesses antioxidant properties.
In conclusion, therefore, we can say that I. gracilis contains antioxidative and antidiabetic properties and may be included in the list of medicinal plants with antidiabetic potential. Further studies on this plant to identify the active principles and detailed mechanism of action will be useful.
Acknowledgements
The authors thank the Department of Biochemistry, North Eastern Hill University, Shillong, India, for providing facilities.
Declaration of interest
The authors affirm that they have no declarations of interest. The authors thank the UGC for sponsoring the University with potential for Excellence Project, DST, DBT and the Department of Biochemistry, North Eastern Hill University, Shillong, India, for providing facilities.
References
- Al-Aboudi A, Afifi FU. (2011). Plants used for the treatment of diabetes in Jordan: A review of scientific evidence. Pharm Biol 49:221–39
- Attele AS, Zhou YP, Xie JT, et al. (2002). Antidiabetic effects of Panax ginseng berry extract and the identification of an effective component. Diabetes 51:1851–8
- Bailey CJ, Day C. (1989). Traditional plant medicines as treatments for diabetes. Diabetes Care 12:553–64
- Boccuzzi SJ, Wogen J, Fox J, et al. (2001). Utilization of oral hypoglycemic agents in a drug-insured US population. Diabetes Care 24:1411–15
- Bouayed J, Bohn T. (2010). Exogenous antioxidants – Double-edged swords in cellular redox state: Health beneficial effects at physiologic doses versus deleterious effects at high doses. Oxid Med Cell Longev 3:228–37
- Bouayed J, Bohn T. (2012). Dietary derived antioxidants: Implications on health. In: Bouayed J, ed. Nutrition, Well-being and Health. USA: InTech. Available from: http://www.intechopen.com/books/nutrition-well-being-and-health/dietary-derived-antioxidants-implication-on-health. ISBN: 978-953-51-0125-3
- Bradford MM. (1976). A rapid and sensitive method for the quantitation of microgram quantities of protein utilizing the principle of protein-dye binding. Anal Biochem 72:248–54
- Brand-Williams W, Cuvelier ME, Berset C. (1995). Use of a free radical method to evaluate antioxidant activity. LWT – Food Sci Technol 28:25–30
- Brighente IMC, Dias M, Verdi LG, Pizzolatti MG. (2007). Antioxidant activity and total phenolic content of some Brazilian species. Pharm Biol 45:156–61
- Campos MRS, Gómez KR, Ordoñez YM, Ancona DB. (2013). Polyphenols, ascorbic acid and carotenoids contents and antioxidant properties of Habanero pepper (Capsicum chinense) fruit. FNS 4:47–54
- Ceretta LB, Réus GZ, Abelaira HM, et al. (2012). Increased oxidative stress and imbalance in antioxidant enzymes in the brains of alloxan-induced diabetic rats. Exp Diabetes Res 2012:Article ID: 302682. doi:10.1155/2012/302682
- Ceriello A, dello Russo P, Amstad P, Cerutti P. (1996). High glucose induces antioxidant enzymes in human endothelial cells in culture: Evidence linking hyperglycemia and oxidative stress. Diabetes 45:471–7
- Cetto AA, Wiedenfeld H, Revilla MC, Sergio IA. (2000). Hypoglycemic effect of Equisetum myriochaetum aerial parts on streptozotocin diabetic rats. J Ethnopharmacol 72:129–33
- Chen HH, Yu C, Ueng TH, et al. (1998). Acute and subacute toxicity study of water-soluble polyalkyl sulfonated C60 in rats. Toxicol Pathol 26:143–51
- Chis IC, Ungureanu MI, Marton A, et al. (2009). Antioxidant effects of a grape seed extract in a rat model of diabetes mellitus. Diab Vasc Dis Res 6:200–4
- Codario RA. (2005). Type 2 Diabetes, Pre-Diabetes, and the Metabolic Syndrome: The Primary Care Guide to Diagnosis and Management. Totowa, NJ: Humana Press
- Donath MY, Shoelson SE. (2011). Type 2 diabetes as an inflammatory disease. Nat Rev Immunol 11:98–107
- Egert S, Rimbach G. (2011). Which sources of flavonoids: Complex diets or dietary supplements? Adv Nutr 2:8–14
- Eidi A, Eidi M, Esmaeili E. (2006). Antidiabetic effect of garlic (Allium sativum L.) in normal and streptozotocin-induced diabetic rats. Phytomedicine 13:624–9
- Erasto P, Adebola PO, Grierson DS, Afolayan AJ. (2005). An ethnobotanical study of plants used for the treatment of diabetes in the Eastern Cape Province, South Africa. Afr J Biotechnol 4:1458–60
- Esposito K, Nappo F, Marfella R, et al. (2002). Inflammatory cytokine concentrations are acutely increased by hyperglycemia in humans, role of oxidative stress. Circulation 106:2067–72
- Gray AM, Flatt PR. (1999). Insulin-releasing and insulin like activity of the traditional anti-diabetic plant Coriandrum sativum (coriander). Br J Nutr 81:203–9
- Halliwel B. (2007). Dietary polyphenols: Good, bad, or indifferent for your health? Cardiovasc Res 73:341–7
- Harborne JB. (1998). Phytochemical Methods, 3rd ed. London: Chapman & Hall
- Harvey AL. (2008). Natural products in drug discovery. Drug Discov Today 13:894–901
- Hermans N, Cos P, Maes L, De Bruyne T, et al. (2007). Challenges and pitfalls in antioxidant research. Curr Med Chem 14:417–30
- Hu FB. (2011). Globalization of diabetes the role of diet, lifestyle, and genes. Diabetes Care 34:1249–57
- Kayang H. (2007). Tribal knowledge on wild edible plants of Meghalaya, Northeast India. Indian J Tradit Know 6:177–81
- Kniewald J, Kmetic I, Gaurina-Srcek V, Kniewald Z. (2005). Alternative models for toxicity testing of xenobiotics. Arh Hig Rada Toksikol 56:195–204
- Kroder G, Bossenmaier B, Kellerer M, et al. (1996). Tumor necrosis factor-alpha-and hyperglycemia-induced insulin resistance. Evidence for different mechanisms and different effects on insulin signaling. J Clin Invest 97:1471–7
- Lamaison JLC, Carnet A. (1990). Teneurs en principaux flavonoids des fleurs de Crataegeus monogyna Jacq et de Crataegeus laevigata (Poiret D. C) en fonction de la vegetation. Pharm Acta Helv 65:315–20
- Manach C, Scalbert A, Morand C, et al. (2004). Polyphenols: Food sources and bioavailability. Am J Clin Nutr 79:727–47
- Maritim AC, Sanders RA, Watkins III JB. (2003). Diabetes, oxidative stress, and antioxidants: A review. J Biochem Mol Toxic 17:24–38
- Marklund SL, Marklund G. (1974). Involvement of the superoxide anion radical in the autoxidation of pyrogallol and a convenient assay for superoxide dismutase. Eur J Biochem 47:469–74
- Marles RJ, Farnsworth NR. (1995). Antidiabetic plants and their active constituents. Phytomedicine 2:137–89
- Mayakrishnan V, Veluswamy S, Sundaram KS, et al. (2013). Free radical scavenging potential of Lagenaria siceraria (Molina) Standl fruits extract. Asian Pac J Trop Med 6:20–6
- Molyneux P. (2004). The use of the stable free radical diphenylpicrylhydrazyl (DPPH) for estimating antioxidant activity. Songklanakarin J Sci Technol 26:211–19
- Morales P, Ferreira IC, Carvalho AM, et al. (2014). Mediterranean non-cultivated vegetables as dietary sources of compounds with antioxidant and biological activity. LWT-Food Sci Technol 55:389–96
- Olabi B, Bhopal R. (2009). Diagnosis of diabetes using the oral glucose tolerance test. BMJ 339:b4354
- Olaokun OO, McGaw LJ, Eloff JN, Naidoo V. (2013). Evaluation of the inhibition of carbohydrate hydrolysing enzymes, antioxidant activity and polyphenolic content of extracts of ten African Ficus species (Moraceae) used traditionally to treat diabetes. BMC Complem Altern M 13:1–10
- Panda SP, Haldar PK, Bera S, et al. (2010). Antidiabetic and antioxidant activity of Swietenia mahagoni in streptozotocin-induced diabetic rats. Pharm Biol 48:974–9
- Pandey KB, Rizvi SI. (2009). Plant polyphenols as dietary antioxidants in human health and disease. Oxid Med Cell Longev 2:270–8
- Patel DK, Prasad SK, Kumar R, Hemalatha S. (2012). An overview on antidiabetic medicinal plants having insulin mimetic property. Asian Pac J Trop Med 2:320–30
- Patil SB, Dongare VR, Kulkarni CR, et al. (2013). Antidiabetic activity of Kalanchoe pinnata in streptozotocin-induced diabetic rats by glucose independent insulin secretagogue action. Pharm Biol 51:1411–18
- Ramachandran A, Das AK, Joshi SR, et al. (2010). Current status of diabetes in India and need for novel therapeutic agents. J Assoc Phys India 58:7–9
- Ramachandran A, Snehalatha C, Shetty AS, Nanditha A. (2012). Trends in prevalence of diabetes in Asian countries. World J Diabetes 3:110–17
- Robertson RP, Harmon J, Phuong Oanh T, et al. (2004). β-Cell glucose toxicity, lipotoxicity, and chronic oxidative stress in type 2 diabetes. Diabetes 53:S119–24
- Rotruck JT, Pope AL, Ganther HE, et al. (1973). Selenium: Biochemical role as a component of glutathione peroxidase. Science 179:588–90
- Sadi G, Guray T. (2009). Gene expressions of Mn-SOD and GPx-1 in streptozotocin induced diabetes: Effect of antioxidants. Mol Cell Biochem 327:127–34
- Scalbert A, Johnson IT, Saltmarsh M. (2005). Polyphenols: Antioxidants and beyond. Am J Clin Nutr 81:215S–17S
- Scalbert A, Williamson G. (2000). Dietary intake and bioavailability of polyphenols. J Nutr 130:2073–85
- Senapati MR, Behera PC, Parija SC, Bisoi PC (2013). HPTLC finger print of phytophenols and flavonoids of Artimisia nilagirica under different extraction regimen. Am J Pharm Tech Res 3:838–48
- Shah H, Bodhankar S, Bhonde R, Mohan V. (2006). Combinative therapeutic approach for better blood sugar level control in alloxan diabetic mice. Int J Diabetes Metabol 14:104–5
- Singleton VL, Orthofer R, Lamuela-raventos RM. (1999). Analysis of total phenols and other oxidation substrates and antioxidants by means of Folin-Ciocalteu reagent. In: Methods in Enzymology: Oxidants and Antioxidants, Part A, vol. 299. San Diego, CA: Elsevier Inc, 152–178
- Swaroop JJ, Rajarajeswari D, Naidu JN. (2012). Association of TNF-α with insulin resistance in type 2 diabetes mellitus. Indian J Med Res 135:127–30
- Syiem D, Syngai G, Khup PZ, et al. (2002). Hypoglycemic effects of Potentilla fulgens L. in normal and alloxan induced diabetic mice. J Ethnopharmacol 83:55–61
- Syiem D, Warjri P. (2013). The effect of aqueous extract of Smilax perfoliata on blood glucose, lipids and selected hepatic enzymes of alloxan-induced diabetic mice. Int J Pharm Bio Sci 4:945–55
- Syiem D, Khup PZ. (2006). Study of the traditionally used medicinal plant Osbeckia chinensis for hypoglycemic and anti-hyperglycemic effects in mice. Pharm Biol 44:613–18
- Syiem D, Warjri P. (2011). Hypoglycemic and antihyperglycemic effects of aqueous extract of Ixeris gracilis DC. on normal and alloxan-induced diabetic mice. Diabetol Croat 40:89–95
- Tonelli J, Li W, Kishore P, et al. (2004). Mechanisms of early insulin-sensitizing effects of thiazolidinediones in type 2 diabetes. Diabetes 53:1621–9
- Wild S, Roglic G, Green A, et al. (2004). Global prevalence of diabetes: Estimates for the year 2000 and projections for 2030. Diabetes Care 27:1047–53
- Yemm EW, Willis AJ. (1954). The estimation of carbohydrates in plant extracts by anthrone. Biochem J 57:508–14
- Yibchok-Anun S, Adisakwattana S, Yao CY, et al. (2006). Slow acting protein extract from fruit pulp of Momordica charantia with insulin secretagogue and insulinomimetic activities. Biol Pharm Bull 29:1126–31
- Zhang XF, Tan BKH. (2000). Effects of an ethanolic extract of Gynura procumbens on serum glucose, cholesterol and triglyceride levels in normal and streptozotocin-induced diabetic rats. Singapore Med J 41:9–13