Abstract
Context: Atractylodes macrocephala Koidz is a traditional herb. Atractylodes macrocephalaon polysaccharides (AMP) have been found to enhance immunity and improve heart function. However, the mechanisms of the immunomodulatory effect have not been investigated.
Objective: We examined whether AMP activated macrophages and explored the mechanisms of activation.
Materials and methods: AMP was prepared and evaluated its immunomodulatory activity (25, 50, 100, and 200 μg/mL) by detecting the phagocytosis and the production of tumor necrosis factor-α (TNF-α), IFN-γ, and nitric oxide (NO) in RAW264.7 macrophages. Furthermore, the role of nuclear factor-κB (NF-κB) pathway was examined in regulating TNF-α and NO production.
Results: The phagocytosis of macrophages was enhanced by AMP in a dose-dependent manner and the maximal phagocytosis of macrophages occurred at concentrations of 100 and 200 μg/mL. NO, TNF-α, and IFN-γ release was also found to be dose dependent by increasing concentrations of AMP and reached the peak at a concentration of 200 μg/mL. In addition, AMP induced inhibitor kappaB (IκB) degradation and the activation of NF-κB by p65 nuclear translocation, and then the activation of NF-κB in nucleus peaked at a concentration of 200 μg/mL. Besides, NF-κB-specific inhibitor pyrrolidine dithiocarbamate (PDTC) decreased AMP-induced NO and TNF-α production.
Discussion and conclusion: These data suggest that AMP may modulate macrophage activities by stimulating NF-κB or activating NF-κB-dependent mechanisms.
Introduction
Macrophages play critical roles in immune defense, including the phagocytosis of pathogens, the production of many cytokines, and the proteolytic processing and presentation of foreign antigens. Macrophages also serve as a source of various proinflammatory cytokines or mediators such as nitric oxide (NO), tumor necrosis factor-α (TNF-α), and IFN-γ. NO and TNF-α as the main effector molecules play a critical role in the innate immunity (MacMicking et al., Citation1997). Nuclear factor-κB (NF-κB), a transcription factor, plays a vital role in modulating the transcription of many immunomodulatory mediators (Chen et al., Citation1999). NF-κB is located in the cytosol in quiescent cells. When activated, NF-κB translocates into the nucleus. NF-κB regulates immune and inflammatory response such as inducible nitric oxide synthase (iNOS) and TNF-α (Ngo et al., Citation2011).
Recently, studies on polysaccharides from natural products have increasingly been done for wide therapeutic application and relatively low toxicity (Chen et al., Citation2011; Zhang et al., Citation2011; Zhao et al., Citation2010). It is well known that polysaccharides enhance various immune responses in vivo and in vitro (Ladányi et al., Citation1993; Lee & Jeon, Citation2003; Liu et al., Citation1996; Tzianabos, Citation2000). In addition, many polysaccharides activated immune responses first by activation of macrophages (Lee & Jeon, Citation2005).
Atractylodes macrocephala Koidz is a traditional Chinese medicine from Zhejiang, Jiangsu, and Anhui Provinces in China. A recent study has reported that the polysaccharides extract of Atractylodes macrocephala enhanced immunity and improved heart function in aged rats (Guo et al., Citation2012). However, little is known regarding the role of Atractylodes macrocephalaon polysaccharides (AMP) in macrophage activation. Here, we first examined the effect of AMP on the phagocytosis and the production of NO, TNF-α, and IFN-γ production in RAW264.7 macrophages. Moreover, the activation of NF-κB by AMP was also investigated in mouse macrophages.
Materials and methods
Reagents and antibodies
Atractylodes macrocephala was extracted using the traditional boiling water extraction method. The supernatant was applied to a DEAE-Sephacel column, and bound materials were eluted with a linear gradient of NaCl (0–2 mol/L NaCl). The fractions containing carbohydrate were pooled and precipitated three times with ethanol. The polysaccharide extract was dialyzed and lyophilized. Its primary structural features and molecular weight were characterized (Liang et al., Citation2007). AMP was a homogeneous polysaccharide, with a weight-average molecular weight of 8.374 kDa based on the high-performance liquid chromatography analysis. AMP was mainly composed of glucose, galactose, rhamnose, and mannose in a molar ratio of 7.36:1.00:3.05:1.52, detected by gas chromatography. Less than 0.005 EU (endotoxin units) of endotoxin was present in 1 mg of AMP as tested by Limulus amebocyte lysate assay. This level of endotoxin did not affect the experimental results obtained by AMP. FITC-dextran and lipopolysaccharide (LPS) were obtained from Sigma (St. Louis, MO). Inhibitor kappaB (IκB), NF-κB p65, LaminB1, and β-actin antibodies were from Santa Cruz Biotechnology (Santa Cruz, CA).
Cell lines-RAW264.7 cells
The murine macrophage cell line RAW264.7 (American Type Culture Collection, Rockville, MD) was cultured in Dulbecco's Modified Eagle Medium (DMEM) containing 10% heat-inactivated fetal bovine serum (Gibco, Gran Island, NY), and antibiotics (100 U/mL penicillin and 100 μg/mL streptomycin), and were maintained at 37 °C in 5% CO2 incubators.
Cell viability
Cells were seeded into 96-well plates for 24 h, and then incubated for 24 h after stimulation in the presence or absence of AMP or 100 ng/mL LPS. MTT solution (5 mg/mL) was added, and the cells were incubated at 37 °C for 4 h. The crystallized MTT was dissolved in DMSO, and the absorbance was measured at 540 nm using a microplate reader.
Phagocytic uptake
Macrophages (1 × 106 cells/mL) were preincubated with different concentrations of AMP (25, 50, 100, and 200 μg/mL) or 100 ng/mL LPS, and then incubated with FITC-dextran (1 mg/mL) at 37 °C for 1 h. The incubation was stopped by the addition of cold phosphate-buffered saline (PBS), and the cells were washed four times. After fixing the cells with 2% paraformaldehyde, phagocytic uptake was analyzed by a flow cytometer (FACS caliber, Beckton Dikinson, Franklin Lakes, NJ).
NO assay
Macrophages were incubated with different concentrations of AMP (25, 50, 100, and 200 μg/mL) or 100 ng/mL LPS for 24 h. Supernatants were harvested and the level of NO was determined by Griess reagent (Promega, Madison, WI). Cell culture supernatant (50 μL) was mixed with an equal volume of Griess reagent and incubated for 10 min. The absorbance was measured at 550 nm using a 96-well assay plates, and a standard curve was obtained using a serial known concentration of NaNO2.
Determination of TNF-α and IFN-γ
Macrophages were incubated with different concentrations of AMP (25, 50, 100, and 200 μg/mL) or 100 ng/mL LPS for 24 h. Supernatants were harvested and the level of TNF-α and IFN-γ was determined by enzyme-linked immunosorbent assay (ELISA) using a commercial kit (R&D Systems) according to the manufacturer's instructions.
Western blotting
After removal of the medium, macrophages were washed by 1 mL of ice-cold PBS (pH 7.2), centrifuged at 1000g for 5 min, resuspended in 400 μL of ice-cold hypotonic buffer (10 mM HEPES-KOH pH 7.9, 2 mM MgCl2, 0.1 mM EDTA, 10 Mm KCl, 1 mM DTT, 1 μg/mL leupeptin, and 1 mM PMSF), left on ice for 10 min, vortexed, and centrifuged at 15 000g (at 4 °C for 30 s). Pelleted nuclear protein was resuspended in 50 μL of ice-cold saline buffer (50 mM HEPES-KOH pH 7.9, 10% glycerol, 300 mM NaCl, 1.5 mM KCl, 0.1 mM EDTA, 1 mM DTT, 1 μL/mL leupeptin, and 1 mM PMSF), left on ice for 20 min, vortexed, and centrifuged at 15 000g (at 4 °C for 10 min). The total proteins of RAW 264.7 cells were directly prepared in lysis buffer (0.5% Triton, 50 mM β-glycero phosphate ( pH 7.2), 0.1 mM sodium vanadate, 2 mM MgCl2, 1 mM EGTA, 1 mM dithiothreitol, 2 μg/mL leupeptin, 0.1 mM phenylmethyl-sulfonyl urea, and 4 μg/mL aprotinin). The proteins were separated on SDS-PAGE and transferred onto the PVDF membrane. The membrane was incubated overnight at 4 °C with primary antibodies and incubated with second antibody for 1 h at room temperature. Chemiluminescence was detected using the ECL plus reagent according to the manufacturer's recommended protocol.
Statistical analysis
Results are expressed as mean ± SD. Statistical significance of results was determined by Student's t-test or ANOVA followed by Turkey's post hoc test, where appropriate. Results were determined to be statistically significant when p values < 0.05 were obtained. All experiments were repeated at least three times.
Results
Cell viability
The cell viability was assessed by MTT assay. As shown in , AMP showed no inhibitory effect on cell proliferation at concentrations ranging from 1 to 200 µg/mL. LPS also showed no inhibitory effect on cell proliferation. The range of concentrations of AMP applied in subsequent experiments.
Effect of AMP on phagocytosis of macrophages
Macrophage function was assessed by lysosomal enzymes and phagocytic activities. To investigate the effect of AMP on the phagocytic activity of macrophages, we measured phagocytic activity for FITC-dextran with mouse macrophages. Thus, the phagocytosis of macrophages induced by AMP or LPS was examined. As shown in , LPS enhanced phagocytosis of macrophages. The phagocytosis of macrophages was also enhanced by AMP in a dose-dependent manner and the maximal phagocytosis of macrophages occurred at concentrations of 100 and 200 μg/mL.
Figure 2. Effect of AMP on phagocytosis of macrophages. Macrophages (1 × 106 cells/mL) were preincubated with different concentrations of AMP (25, 50, 100, and 200 μg/mL) or 100 ng/mL LPS, and then incubated with FITC-dextran (1 mg/mL) at 37 °C for 1 h. The extent of the phagocytic uptake was determined by flow cytometry. *p < 0.05.
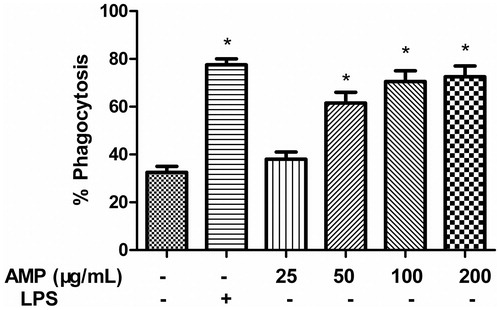
Effect of AMP on NO production
Since NO plays a critical role in the non-specific immune defense, NO production from macrophages stimulated by AMP or LPS was examined. We found that LPS induced NO production. NO production was also found to be dose dependent by increasing the concentrations of AMP and reached the peak at a concentration of 200 μg/mL ().
Figure 3. NO, TNF-α and IFN-γ production in macrophages incubated with AMP. Macrophages were incubated with different concentrations of AMP (25, 50, 100, and 200 μg/mL) or 100 ng/mL LPS for 24 h. Supernatants were harvested and the level of NO, TNF-α, and IFN-γ was determined by the Griess reagent and ELISA. *p < 0.05.
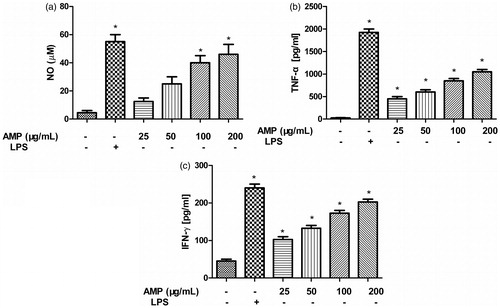
Effect of AMP on TNF-α and IFN-γ production
Cytokines play important roles in defense functions such as immune responses and inflammatory reactions and were used to evaluate augmentation activity of innate immunity. The effects of AMP or LPS on the production of TNF-α and IFN-γ by macrophages were determined by ELISA. Macrophages were treated with different concentrations of AMP for 24 h, and supernatants were collected. LPS induced TNF-α and IFN-γ release. TNF-α () and IFN-γ () secretion was also found to be dose dependent by increasing concentrations of AMP and reached the peak at a concentration of 200 μg/mL.
IκB degradation and activation of NF-κB in macrophages treated with AMP
NF-κB as a ubiquitous transcription factor plays a vital role in macrophage activation via the transcription of downstream inflammatory mediators (Li et al., Citation2011). Thus IκB degradation and activation of NF-κB were investigated in macrophages stimulated with AMP. AMP induced IκB degradation () and activation of NF-κB () in a dose-dependent manner. Moreover, the activation of NF-κB in nucleus peaked at a concentration of 200 μg/mL.
Figure 4. IκB degradation and activation of NF-κB in macrophages treated with AMP. Macrophages were stimulated with different concentrations of AMP for 4 h. (a and c) IκB degradation and activation of NF-κB was analyzed by Western blotting with anti-IκB and anti-NF-κB p65 antibodies. (b and d) Expression of IκB and NF-κB p65 was quantified. The ratios for these proteins are shown. *p < 0.05 compared with the untreated group.
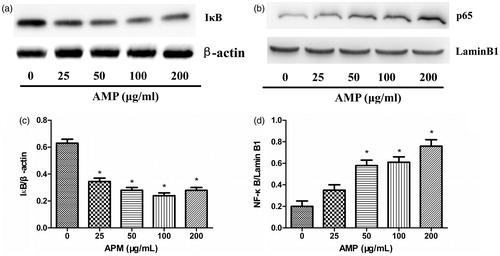
Effect of NF-κB inhibitor on NO, TNF-α and IFN-γ production in macrophages stimulated by AMP
To explore the signaling mechanisms, we investigated the effect of NF-κB on NO and TNF-α secretion in the absence and in the presence of NF-κB inhibitor pyrrolidine dithiocarbamate (PDTC). Macrophages were pretreated with PDTC (100 μM) for 90 min, and then stimulated by AMP. NO () and TNF-α () production was inhibited by PDTC in macrophages after stimulation with AMP. However, IFN-γ () secretion was not inhibited by PDTC.
Figure 5. Effect of NF-κB inhibitor on NO, TNF-α, and IFN-γ production in macrophages incubated with AMP. Macrophages were pretreated with PDTC (100 μM) for 90 min, and then stimulated by AMP. Supernatants were harvested and the level of NO (a), TNF-α (b), and IFN-γ (c) was determined by ELISA and Griess reagent.
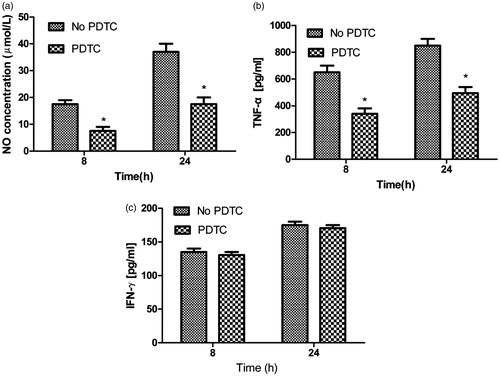
Discussion
Recently, there have been some studies regarding the role of polysaccharides in macrophage activation. In the present study, AMP isolated from Atractylodes macrocephala was confirmed as an immunomodulator of macrophages. Our hypotheses were supported by the following observations: AMP enhanced the phagocytosis of macrophages. The treatment of AMP to macrophages increased the production of NO, TNF-α, and IFN-γ and induced IκB degradation and NF-κB activation. Furthermore, inhibition of NF-κB activation decreased NO and TNF-α production.
Phagocytosis is the first step of macrophage response to the invading microorganisms. Thus, phagocytosis was enhanced by AMP, implying the elevation of innate immune response. This is consistent with purified Ganoderma atrum black (Yu et al., Citation2013) and Astragalus membranaceus (Zhao et al., Citation2011) polysaccharides. In addition to proinflammatory cytokines, NO has been recognized as the new type of mediator which has the similar functions to these cytokines. NO is regarded as the central molecular in the regulation of immune response (Jiao et al., Citation2009). IFN-γ and TNF-α regulate immune and inflammatory reactions. TNF-α also can act on monocytes and macrophages in an autocrine manner to enhance various function responses and induce the expression of a number of other immunoregulatory and inflammatory mediators. Since NO, IFN-γ, and TNF-α play a critical in the antimicrobial and tumoricidal activities of macrophages, great attention has been paid to developing drugs that modulate NO and TNF-α production (Poderoso et al., Citation1999). Many polysaccharides can activate macrophages by inducing the production of cytokines (Belska et al., Citation2010; Lee et al., Citation2010; Togola et al., Citation2008). Our study showed that AMP promoted NO, TNF-α, and IFN-γ secretion, indicating its stimulatory effect in the host defense against microbial infection on activated macrophages.
In the present study, NF-κB is positively regulated by AMP for NO and TNF-α production. NF-κB associated with inhibitor proteins IκBs exists in the cytoplasm of unstimulated cells. However, when the cells are activated by certain external stimuli, IκBs are rapidly phosphorylated, degraded, and thereby dissociated from NF-κB. The active NF-κB translocates to the nucleus and regulates immune and inflammatory response such as iNOS and TNF-α (Lawrence & Fong, Citation2010; Karin & Greten, Citation2005). Moreover, activation of the NF-κB transcription factor is often related to the secretion of inflammatory cytokines (Baldwin, Citation1996; Barnes & Karin, Citation1997; Sweet & Hume, Citation1996). There is evidence that supports NO and TNF-α production by AMP through the positive regulation of NF-κB. First, AMP markedly induced IκB degradation and the activation of NF-κB. Second, specific inhibitor of NF-κB significantly suppressed AMP-induced NO and TNF-α production, indicating that AMP stimulated macrophages to produce NO and TNF-α through IκB degradation and the activation of NF-κB. Besides, recent studies have shown that expression of a subset of IFN-γ-regulated genes requires the function of the IKK (inhibitor of NF-κB (IκB) kinase) complex and the effects of IKK complex on IFN-γ-inducible gene transcription are independent of NF-κB (Sizemore et al., Citation2004). Our data also showed that IFN-γ secretion was not inhibited by PDTC, indicating that IFN-γ secretion was not regulated by NF-κB and has additional signaling pathways.
Conclusion
This study demonstrated that AMP stimulated macrophages to promote phagocytic activity and the production of NO, TNF-α, and IFN-γ. In addition, NF-κB was involved in the release of NO and TNF-α in AMP-activated macrophages. These data suggest that the polysaccharide isolated from Atractylodes macrocephala activates macrophages and has potent immunostimulating activity. Further study is needed to elucidate the upstream signaling pathways in host immune and inflammatory responses in the presence of AMP.
Declaration of interest
There are no conflicts of interest. The authors alone are responsible for the content and writing of the paper.
References
- Baldwin AS Jr. (1996). The NF-kappa B and I kappa B proteins: New discoveries and insights. Annu Rev Immunol 14:649–83
- Barnes PJ, Karin M. (1997). Nuclear factor-kappaB: A pivotal transcription factor in chronic inflammatory diseases. N Engl J Med 336:1066–71
- Belska NV, Guriev AM, Danilets MG, et al. (2010). Water-soluble polysaccharide obtained from Acorus calamus L. classically activates macrophages and stimulates Th1 response. Int Immunopharmacol 10:933–42
- Chen F, Castranova V, Shi X, Demers LM. (1999). New insights into the role of nuclear factor-kappaB, a ubiquitous transcription factor in the initiation of diseases. Clin Chem 45:7–17
- Chen X, Wang Y, Wu Y, et al. (2011). Green tea polysaccharide-conjugates protect human umbilical vein endothelial cells against impairments triggered by high glucose. Int J Biol Macromol 49:50–4
- Guo L, Sun YL, Wang AH, et al. (2012). Effect of polysaccharides extract of rhizoma Atractylodis macrocephalae on thymus, spleen and cardiac indexes, caspase-3 activity ratio, Smac/DIABLO and HtrA2/Omi protein and mRNA expression levels in aged rats. Mol Biol Rep 39:9285–90
- Jiao L, Li X, Li T, et al. (2009). Characterization and anti-tumor activity of alkali-extracted polysaccharide from Enteromorpha intestinalis. Int Immunopharmacol 9:324–9
- Ladányi A, Tímár J, Lapis K. (1993). Effect of lentinan on macrophage cytotoxicity against metastatic tumor cells. Cancer Immunol Immunother 36:123–6
- Lawrence T, Fong C. (2010). The resolution of inflammation: Anti-inflammatory roles for NF-kappaB. Int J Biochem Cell Biol 42:519–23
- Lee JS, Kwon JS, Yun JS, et al. (2010). Structural characterization of immunostimulating polysaccharide from cultured mycelia of Cordyceps militaris. Carbohydr Polym 80:1011–17
- Lee KY, Jeon YJ. (2003). Polysaccharide isolated from Poria cocos sclerotium induces NF-kappaB/Rel activation and iNOS expression in murine macrophages. Int Immunopharmacol 3:1353–62
- Lee KY, Jeon YJ. (2005). Macrophage activation by polysaccharide isolated from Astragalus membranaceus. Int Immunopharmacol 5:1225–33
- Liang ZH, Guo ZX, Zhang LP. (2007). Structure aspects of water soluble polysaccharide isolated from Atractylodes macrocephala Koidz. J Mol Sci 23:185–8
- Li H, Kim JY, Hyeon J, et al. (2011). In vitro antiinflammatory activity of a new sesquiterpene lactone isolated from Siegesbeckia glabrescens. Phytother Res 25:1323–7
- Liu F, Fung MC, Ooi VE, Chang ST. (1996). Induction in the mouse of gene expression of immunomodulating cytokines by mushroom polysaccharide–protein complexes. Life Sci 58:1795–803
- MacMicking J, Xie QW, Nathan C. (1997). Nitric oxide and macrophage function. Annu Rev Immunol 15:323–50
- Ngo DH, Qian ZJ, Vo TS, et al. (2011). Antioxidant activity of gallate-chitooligo saccharides in mouse macrophage RAW264.7 cells. Carbohydr Polym 84:1282–8
- Poderoso JJ, Carreras MC, Schöpfer F, et al. (1999). The reaction of nitric oxide with ubiquinol: Kinetic properties and biological significance. Free Radic Biol Med 26:925–35
- Karin M, Greten FR. (2005). NF-kappaB: Linking inflammation and immunity to cancer development and progression. Nat Rev Immunol 5:749–59
- Sizemore N1, Agarwal A, Das K, et al. (2004). Inhibitor of kappaB kinase is required to activate a subset of interferon gamma-stimulated genes. Proc Natl Acad Sci USA 101:7994–8
- Sweet MJ, Hume DA. (1996). Endotoxin signal transduction in macrophages. J Leukoc Biol 60:8–26
- Togola A, Inngjerdingen M, Diallo D, et al. (2008). Polysaccharides with complement fixing and macrophage stimulation activity from Opilia celtidifolia, isolation and partial characterisation. J Ethnopharmacol 115:423–31
- Tzianabos AO. (2000). Polysaccharide immunomodulators as therapeutic agents: Structural aspects and biologic function. Clin Microbiol Rev 13:523–33
- Yu Q, Nie SP, Li WJ, et al. (2013). Macrophage immunomodulatory activity of a purified polysaccharide isolated from Ganoderma atrum. Phytother Res 27:186–91
- Zhang H, Wang ZY, Zhang Z, Wang X. (2011). Purified Auricularia auricular-judae polysaccharide (AAP I-a) prevents oxidative stress in an ageing mouse model. Carbohydr Polym 84:638–48
- Zhao LH, Ma ZX, Zhu J, et al. (2011). Characterization of polysaccharide from Astragalus radix as the macrophage stimulator. Cell Immunol 271:329–34
- Zhao LY, Dong YH, Chen GT, Hu QH. (2010). Extraction, purification, characterization and antitumor activity of polysaccharides from Ganoderma lucidum. Carbohydr Polym 80:783–9