Abstract
Context: The fruits of Aronia melanocarpa Elliot (Rosaceae), (black chokeberry), and Sambucus nigra L. (Caprifoliaceae), elderberries are rich in anthocyanins. Many studies have reported that anthocyanins are beneficial in diabetes due to their capacity to stimulate insulin secretion and reduce oxidative stress.
Objective: The purpose of this study is to prove the biologically active properties of polyphenols extracted from S. nigra and A. melanocarpa fruit. The study also details the influence of plant polyphenols on immune system imbalances within diabetes mellitus.
Materials and methods: Polyphenolic extract was administered to Wistar rats 0.040 g/kg body every 2 d for 16 weeks. The absorbencies of all the solutions were determined using a V-550 Able Jasco UV–VIS spectrophotometer. The immunomodulatory capacity of vegetal extracts was assessed by studying cytokines TNF-α and IFN-γ through the ELISA method and fibrinogen values.
Results and discussion: At 48 h, the anti-inflammatory effects of S. nigra and A. melanocarpa substances have been revealed by an increase of the TNF-α and IFN-γ levels in the diabetic group protected by these extracts. Seventy-two hours post-administration of both substances in the diabetic groups, the TNF-α level returns to the values read 24 h after substance administration. The vegetal extracts limit the production of fibrinogen in the diabetic rats under polyphenolic protection, the values being highly significant compared with the diabetic group.
Conclusions: Natural polyphenols extracted from S. nigra and A. melanocarpa modulate specific and non-specific immune defenses in insulin-deficiency diabetes and reduce the inflammatory status and self-sustained pancreatic insulitis.
Introduction
Patients with diabetes have increased serum expression of key inflammatory cytokines. One hypothesis is that hyperglycemia and the associated oxidative stress damages endothelial cells which in turn release cytokines and chemokines (Groot & Rauen, Citation1998). Studies have shown that hyperglycemia-induced glycation end-products stimulate secretion of monocyte chemoattractant protein from human mesangial cells in vitro (Foss-Freitas et al., Citation2006; Yamagishi et al., Citation2002). The kidneys in streptozotocin-induced diabetic rat models also express elevated levels of inflammatory cytokines (TNF-α and IFN-γ) which are produced by cells in the early and late phases of the disease (Brownlee, Citation2001; Mensah-Brown et al., Citation2005).
The study of the effects of some natural extracts on the cellular and molecular mechanisms involved in immune system disorders within diabetes mellitus is one of the main directions of the current research. Polyphenols may affect glycemia through different mechanisms, including the inhibition of glucose absorbtion in the gut or inhibition of its uptake by peripheral tissues (Cheynier, Citation2005; Manach et al., Citation2004; Yao et al., Citation2004). The metabolites of polyphenols are rapidly eliminated from plasma, which indicates that consumption of plant products on a daily basis is necessary to maintain high concentrations of metabolites in the blood (Yeh et al., Citation2003).
Polyphenols might stimulate TNF-α and IFN-γ secretion, thus limiting inflammatory status and development of microvascular diabetic complications. The purpose of our study is to prove the biologically active properties of polyphenols extracted from Sambucus nigra L. (Caprifoliaceae) (elderberries) and Aronia melanocarpa Elliot (Rosaceae), (black chokeberry) fruit, and to find out the way plant polyphenols influence immune system imbalances within induced diabetes mellitus. The immunomodulatory capacity of substances S. nigra and A. melanocarpa was assessed by studying their influence on the secretion of cytokines TNF-α and IFN-γ, as well as the fibrinogen value.
Material and methods
Ripe berries of A. melanocarpa and S. nigra were collected in Botanical Garden, Iasi, Romania, in October 2009. The plant material was identified by Dr. Violeta Tanasescu. A herbarium voucher sample (AMF.09) is deposited in the Department of Pharmacognosy, School of Pharmacy, University of Medicine and Pharmacy “Gr. T. Popa”, Iasi, Romania.
Preparation of extract and chemical determinations
Sambucus nigra and Aronia melanocarpa fruits were washed and shade-dried. Dried and powdered fruits (50 g) were extracted with 2 × 250 mL acidulated methanol (0.5% HCl) using a magnetic stirrer, each time for 1 h. The extract was concentrated to dryness under reduced pressure (Büchi R-210 rotavapor, Büchi V-850 pressure controller, Büchi V-700 vacuum pump; BÜCHI Labortechnik AG, Flawil, Switzerland). The extract was completely dried before administration to animals. The amount of dry vegetable material extracted was 42.31 g/100 g from S. nigra fruit and 69.34 g/100 g from A. melanocarpa fruit. The total phenolic content in elder fruit extract was determined by the Singleton and Rossi method (Singleton & Rossi, Citation1965). The amount of total phenolic content was expressed as gallic acid equivalents (GAE)/100 g extract. The absorbencies of all the solutions were determined by using a V-550 Able Jasco UV–VIS spectrophotometer (JASCO, Easton, MD). The result is the mean of triplicates ± standard deviation. The total content of polyphenols was 7.63 ± 0.12 mg GAE/100 g from S. nigra extract and 3.67 ± 0.31 mg GAE/100 g from A. melanocarpa extract.
The dry polyphenol extract was diluted in dimetilsulphoxide (DMSO), 100 mL polyphenolic solution containing 840 mg natural polyphenols, 95 mL distilled water, and 5 mL DMSO. After repeated testing, it was found that the dose of polyphenols extracted from the fruits of A. melanocarpa and S. nigra to be administered as an enteral solution (by tube feeding) is 0.040 g/kg body every 2 d. The experiment used active therapeutic doses, well-determined fractions of DL50 on an experimental model of diabetes mellitus. Values of DL50 were determined using the Probit analysis method (Finney, Citation1971). Fractions of DL50 are doses representing 1/5, 1/10, 1/20, and 1/40 of DL50. The dose representing 1/20 of DL50 was chosen, as it is the smallest dose that determined the pharmacodynamic effect that is being researched, without producing significant toxic effects.
Experimental model
The research was carried out on Wistar white male rats, aged 28 weeks at the beginning of the study, weighing 250–280 g on the average, which were divided into four 12-rat groups, namely: W group – control, normal animals, that were not given natural polyphenols; DM group – rats with diabetes caused by the rapid intraperitoneal injection of a single dose of streptozotocin (STZ); A group – rats who were administered A. melanocarpa polyphenolic extract for a period of 16 weeks; S group – rats who were administered S. nigra polyphenolic extract for a period of 16 weeks; DM+A group – rats who were administered an A. melanocarpa polyphenolic extract for 16 weeks after diabetes mellitus induction; DM+S group – rats who were administered a S. nigra polyphenolic extract for 16 weeks after diabetes mellitus induction.
Diabetes was induced by STZ [2-deoxy-2(3-methyl-nitrozo-ureido)-p-glucopiranoza] (cytotoxical antibiotic synthesized of Streptomyces achromonogenes) obtained from Sigma S-0130 (Sigma, St. Louis, MO), Batch 31K1379, and delivered in a single dose of 60 mg/kg body mass, as 1% intraperitoneal (i.p.) solution, after 18 h of fasting. Streptozotocin delivery causes insulin-deficiency diabetes through selective endocrino-chemical pancreatectomy, destroying only the cells of Langerhans’ islets (Saltiel & Pessin, Citation2002).
At the end of the experiment, the 44-week-old animals were killed by cardiac puncture under ketamine anesthesia (100 mg/kg body weight). Blood samples were collected using sodium citrate as an anticoagulant buffer, at a 9:1 blood/citrate ratio, or without anticoagulant. Aliquots of plasma and serum were frozen and kept at − 80°C for later analysis.
Biochemical methodology
TNF-α and IFN-γ determination
The immunomodulatory capacity of substances A. melanocarpa and S. nigra was assessed by studying their influence on the secretion of cytokines TNF-α (Pfeffer et al., Citation1993) and IFN-γ (Dalton et al., Citation1993). For this we used mononuclear cells (i.e. peripheral blood mononuclear cells (PBMC)) from the venous peripheral blood collected from 10 diabetic donors and 10 normal donors. PBMC separation was done by the density gradient centrifugation of 10 mL of heparinized blood collected from each patient, using 5 mL of Ficoll–Hypaque 1077 (Sigma, St. Louis, MO). The PBMC ring formed above the Ficoll layer was collected, washed using 15 mL of simple RPMI (Sigma, St. Louis, MO), and centrifuged at 300 g, at room temperature, for 15 min. After settling, a PBMC count was done in a Bürker–Türk counting chamber. The PBMC were then resuspended in a volume of RPMI + 5% fetal serum designed to allow their culturing in 96-well culture plates at a concentration of 1 × 106 cells/mL/well. PBMC were incubated with substance S. nigra/A. melanocarpa in different concentrations (50, 100, and 150 µg/mL/well). The culture plates were incubated in an incubator at 37°C, 5% CO2. The cytokines secreted in the PBMC supernatant were measured after 24, 48, and 72 h of incubation, when the culture plates were centrifuged at 300 g, at room temperature, for 5 min. The supernatant in each well was collected and used to determine the TNF-α and IFN-γ values by the ELISA method, using Endogen® Human TNF-α and IFN-γ ELISA kits, respectively.
The value of the fibrinogen was coagulometrically determined, using the Clauss (Citation1957) method. The experimental study fulfils all the requirements of the guide regarding the use of laboratory animals and biological preparations issued by the International Society of Pain Study (IASP) and the European Council Committee (86/609/EEC). This study was approved by the Laboratory Animal Care Committee of “Grigore T. Popa” University of Medicine and Pharmacy and the rats were kept in accordance with the general guidelines for the care and use of laboratory animals recommended by the Council of European Communities (approval no. 9803/12.09.2006).
Statistical data interpretation
Data were expressed as means ± standard deviation (SD). Mann–Whitney paired test is basically the same in that they compare between two medians to suggest whether both samples come from the same population or not. A test was performed to determine whether there were significant (p < 0.05) differences between groups, using Statistical Software Package SPSS®, version 13 (SPSS Incorporation, Chicago, IL).
The statistical interpretation of the data considered the corresponding differences for a significance threshold: p > 0.05 statistically insignificant; p < 0.01 strong statistical significance; p < 0.001 very strong statistical significance.
The Pearson coefficient (r) is a measure of the linear correlation (dependence) between two variables, giving a value between +1 and −1 inclusive, where 1 is the total positive correlation, 0 is no correlation, and −1 is the total negative correlation. It is widely used as a measure of the degree of linear dependence between two variables.
Results
The TNF-α serum level was checked in both control and diabetic rats groups 24, 48, and 72 h, after substance A. melanocarpa or S. nigra was administered. About 24 h after substance administration, the control rats that were given substance A. melanocarpa had significantly higher TNF-α values than those that were given the same concentration of substance S. nigra, but only when the concentration was 150 µg/mL (p < 0.001) (). About 24 h after substance administration, the diabetic rats included in the DM + A and DM + S groups that were given the same concentration of substance, i.e., 150 µg/mL, had a significantly higher TNF-α secretion value than the DM group (p < 0.01).
Figure 1. Influence of substances S and A on TNF-α secretion 24 h after administration: in the diabetic groups compared with controls were found significantly lower average TNF-α values in groups receiving substance A (except for the dose of 50 mg/mL (p < 0.05), or substance S regardless of the concentration (p < 0.01 at a concentration of 50 mg/mL and p < 0.001 at a concentration 100 mg/mL). Diabetic rats receiving in 24 h substance A or S at a concentration of 150 mg/mL caused a significant increase in TNF-α as compared with the diabetic rats who had not received either A or S (p < 0.01). In diabetic rats, the same concentrations of solutions A and S did not result in significant differences (p < 0.05).
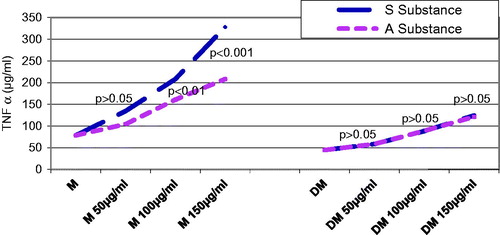
Forty-eight hours after substance administration, the control rats that were given substance S. nigra had significantly different mean TNF-α values, starting with 50 µg/mL, as compared with the control rats that were given substance A. melanocarpa (p < 0.01) (). The control rats that were administered the same concentration of substance A. melanocarpa had significantly higher values of TNF-α than those that were administered substance S. nigra, starting with a concentration of 100 µg/mL (p < 0.01). The diabetic rats that were given 150 µg/mL of substance A. melanocarpa exhibited significantly lower mean TNF-α secretion values than the control rats (p < 0.01); statistically speaking, the mean TNF-α values were significantly lower in the diabetic rats than in the DM + S group, regardless of the concentration of substance S. nigra that was administered (p < 0.01 at a concentration of 50 µg/mL, and p < 0.001 at over 100 µg/mL of substance S. nigra).
Figure 2. Influence of substances S and A on TNF-α secretion 48 h after administration: 48 h after administration, both control and diabetic rats have shown a significant increase in a dose-dependent secretion of TNF-α for both substances. Average values of TNF-α were significantly lower in diabetics compared with controls, regardless of the concentration or substance administered (p < 0.01 at a concentration of 50 mg/mL and p < 0.001 over 100 mg/mL).
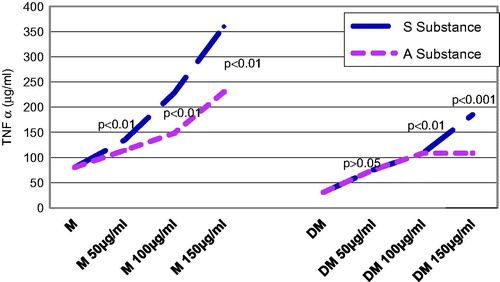
Seventy-two hours after substance administration, the diabetic groups that were given the same concentrations of substance A. melanocarpa or S. nigra did not exhibit statistically significant differences (p > 0.05) ().
Figure 3. Influence of substances S and A on TNF-α secretion 72 h after administration: the level of TNF-α after 72 h from the administration of both substances, both in control groups and in diabetic groups, it is about the same values and the same characteristics as those recorded at 24 h after administration.
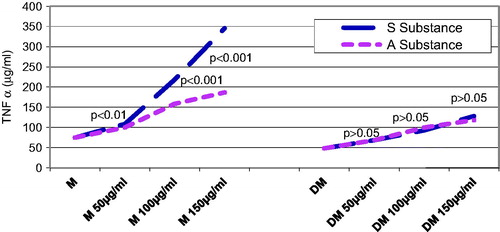
The influence of substances A. melanocarpa and S. nigra on IFN-γ secretion in control and diabetic rats groups was assessed at different points in time, i.e., 24, 48, and 72 h after administration. About 24 h after substance administration in concentrations of at least 100 µg/mL, IFN-γ secretion was significantly higher in the DM + S or DM + A group rats than in the DM group rats (p < 0.05) ().
Figure 4. Influence of substances S and A on IFN-γ secretion 24 h after administration: diabetic group receiving at 24 h, either substance S or substance A, in a concentration of at least 100 mg/mL had a significant increase in secretion of IFN-γ compared with the diabetic group to which these substances have not been administered (p < 0.05). At the same concentration, A and S have shown significant differences (S > A) if administered with a concentration of at least 100 mg/mL (p < 0.05).
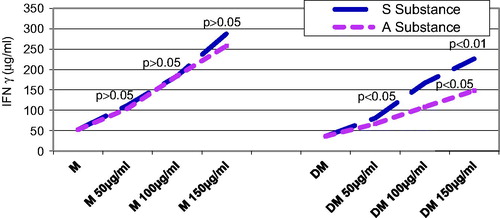
In the case of substance A. melanocarpa, an increase in IFN-γ secretion was noticed after 48 h of administration, regardless of the group. The increase was significant in groups which received substance A. melanocarpa, compared with those who received substance S. nigra. As far as the diabetic group that was administered substance A. melanocarpa is concerned, IFN-γ increases significantly in time with the increase of the administered dose of substance A. melanocarpa. The IFN-γ serum level was much higher when the substance S. nigra was administered than when substance A. melanocarpa was given, regardless of the dose administered to the diabetic group.
Forty-eight hours after the administration of substance S. nigra or A. melanocarpa, the control rats that were given the same concentration of substance A. melanocarpa, i.e. 100 µg/mL, exhibited significantly higher values than the ones that were administered substance S (p < 0.01) (). Forty-eight hours after the administration of at least 50 µg/mL of substance A. melanocarpa, IFN-γ secretion increased very significantly in the diabetic rats that were given the substance as compared with the regular diabetic rats (p < 0.001).
Figure 5. Influence of substances S and A on IFN-γ secretion 48 h after administration: in diabetic rats after 48 h of taking at least 50 mg/mL of substance S, the secretion of IFN-γ has increased significantly in comparison with the untreated diabetic rats (p < 0.01); the same phenomenon is observed with the administration of A substance as well, but the serum level of IFN-γ was lower than in the case of the administration of substance S regardless of the dose.
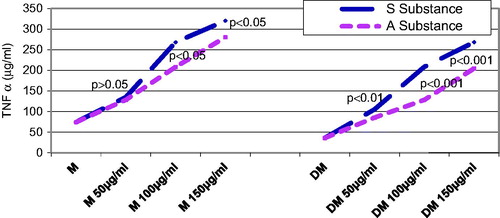
Seventy-two hours after substance administration, the control rats that were given substance S. nigra or A. melanocarpa exhibited very strong significant differences of the mean TNF-α values as compared with the rats that were not given such substances, starting with a concentration of 100 µg/mL (p < 0.001) ().
Figure 6. Influence of substances S and A on IFN-γ secretion 72 h after administration: the diabetic group who received at least 00mL of either S or A substance, compared with the untreated diabetic group, was evidenced a significant increase in IFN-γ (p < 0.001); diabetic groups who received the same concentration of solution A or S did not differ statistically significant (p > 0.05).
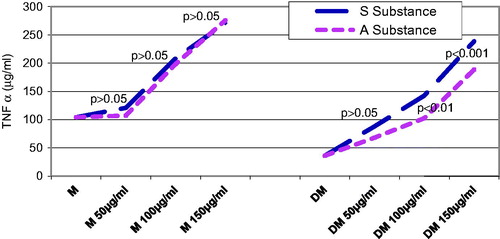
Seventy-two hours after the administration of both substances in the diabetic groups, the TNF-α level returns to approximately the same values read 24 h after substance administration.
The fibrinogen serum concentration is about five times higher in the DM group than in the M group, and it drops very significantly (p < 0.001) in the polyphenol-protected groups M + A and M + S. Although it does not reach the reference values, the DM + A and DM + S groups exhibit a fibrinogen serum concentration that is more than 50% lower than in the DM group ().
Table 1. Influence of substance A. melanocarpa and S. nigra on fibrinogen (g/L).
Discussion
The direct destruction of the β cells through STZ can expose the “cryptic” antigens of the immune system. The infiltration of the pancreatic islands of Langerhans with mononuclear cells (macrophages and T lymphocytes) is one of the characteristics of non-insulin-dependent diabetes mellitus. The lesion of the β-insular cells occurs following direct contact with the infiltrated cells and/or with the products secreted by these cells, called cytokines.
TNF-α plays a major role in the inflammatory process; it stimulates the macrophages in the synthesis of other proinflammatory cytokines: IL-1, IL-6, and IL-12. These interleukins, especially IL-1 and IL-6, contribute greatly to inflammation intensification (Heinrich et al., Citation1990; Pickup et al., Citation2000). On a local level, TNF-α enhances TCD8 lymphocytes adhesion to the vascular endothelium and stimulates their cytotoxicity. It is a proinflammatory cytokine. TNF-α is directly involved in the destruction of insular B-cells, in insulin-dependent diabetes mellitus (McNerlan et al., Citation2002). In contrast, inflammation, which is a non-specific defense mechanism, should be stimulated whenever microbial infections related to diabetes mellitus are involved. Any TNF-α deficit increases the risk of infections related to diabetes mellitus. The immune response is controlled both by direct interactions between different types of co-operating cells and by the synthesis products they secrete (Ozer et al., Citation2003).
The anti-inflammatory effects of S. nigra and A. melanocarpa substances have been revealed by an increase of the TNF-α and IFN-γ levels in the diabetic group protected by these two extracts compared with the unprotected diabetic group. Under these circumstances, a statistical check of these differences is necessary to be able to set the best concentration and the most efficient substance for inducing an increase in TNF-α secretion.
The existence in A. melanocarpa and S. nigra of a high concentration of anthocyanins and proper flavones is a premise for the positive therapeutic effects of these agents on immune system disorders in the evolution of diabetes mellitus (Pascual-Teresa & Sanchez-Ballesta, Citation2008; Scalbert et al., Citation2005). Dietary polyphenols may offer an indirect protection by activating endogenous defense systems and by modulating cellular signaling processes such as NF-κB activation, glutathione biosynthesis, MAPK proteins, and PI3-kinase/Akt pathway (Gray et al., Citation2000; Nijveldt et al., Citation2001; Rahman et al., Citation2006).
The results of our research are in agreement with those quoted in previous studies, according to which the chronic inflammatory reaction related to diabetes mellitus is improved under the action of vegetal polyphenols (Bell & Gochenaur, Citation2006; Trompezinski et al., Citation2003; Ursini et al., Citation1999).
Interferon γ is secreted by activated lymphocytes under the action of specific antigens or of mitogenic substances and it is a type II interferon. Since, in vivo, it is synthesized by activated T lymphocytes, by B series lymphoblasts and by large granular lymphocytes (NK cells), interferon γ is considered to be the immune type (Chen & Liu, Citation2009).
The inflammatory immune response of the islets of Langerhans is known to represent the pathogenetic stage of type 1 diabetes mellitus and to materialize in the insulitis process. The monocytes/macrophages and activated T lymphocytes are the cells infiltrating the islets. It is now that the immunological markers, including anti-islet cytoplasmic antibodies (detected by immunofluorescence), anti-insulin antibodies, and many others, may be detected in the serum. Once the destructive autoimmune process is triggered, this will involve the lymphocytes, natural killer cells (NK), macrophages, and mast cells. The cytokines produced by these cells seem to be responsible for the damage suffered by the pancreatic β-cells. The presence in the diabetic patients’ serum of one or several immunological markers, in significant concentrations, is higher (reaching up to 90%) during the “immunologically active” period preceding the clinical disease onset and then it drops progressively (Mavridis et al., Citation2008).
IFN-γ is expressed in the pancreas of diabetic mice and transgenic overexpression of IFN-γ by beta cells in normal mice leads to the development of autoimmune diabetes (Campbell et al., Citation1991). Furthermore, development of autoimmune diabetes in the non-obese diabetic mice can be prevented by treatment with neutralizing IFN-γ antibodies (Debray-Sachs et al., Citation1991).
Forty-eight hours after substance administration, substance S. nigra determines a significant IFN-γ secretion increase both in the control groups and in the diabetic rats groups. The in vivo studies conducted revealed that the S. nigra and A. melanocarpa polyphenol extract has immunomodulatory properties as it stimulates TNF-α and especially IFN-γ secretion by the peripheral blood mononuclear cells.
The fibrinogen was determined as an acute-phase protein. The extremely high synthesis of the fibrinogen in the diabetic group confirms the self-preserved inflammatory status in the evolution of diabetes mellitus. The polyphenols limit the production of fibrinogen in group DM + A/DM + S, the values being highly significant when compared with the group DM.
Inflammation, and more specifically proinflammatory cytokines and other molecules with a relevant role within the inflammatory process, may be a critical factor in the development of microvascular diabetic complications. This new pathogenic perspective may lead to important new therapeutic considerations and new therapeutic goals for the treatment of diabetes mellitus.
Conclusions
Natural polyphenols extracted from S. nigra and A. melanocarpa modulate specific and non-specific immune defense in insulin-deficiency diabetes and reduce the inflammatory status and self-sustained pancreatic insulitis.
Declaration of interest
The authors declare that they have no competing interests.
Authors’ contributions
M. B. participated in study design, has revisited critically the manuscript for important intellectual content, and given final approval of the version to be published. O. B. carried out the sample analyses and collection of data. L. B. performed the statistical analysis and interpretation of data. M. C. carried out the studies and data analyses and drafted the manuscript. All authors read and approved the final version of the manuscript.
References
- Bell DR, Gochenaur K. (2006). Direct vasoactive and vasoprotective properties of anthocyanin – rich extracts. J Appl Physiol 100:1164–70
- Brownlee M. (2001). Biochemistry and molecular cell biology of diabetic complications. Nature 414:813–20
- Campbell IL, Kay TW, Oxbrow L, Harrison LC. (1991). Essential role for interferon-γ and interleukin-6 in autoimmune insulin-dependent diabetes in NOD/Wehi mice. J Clin Invest 87:739–42
- Chen J, Liu X. (2009). The role of interferon gamma in regulation of CD4 + T-cells and its clinical implications. Cell Immunol 254:85–90
- Cheynier V. (2005). Polyphenols in foods are more complex than often thought. Am J Clin Nutr 81:223S–9S
- Clauss A. (1957). Rapid physiological coagulation method for the determination of fibrinogen. Acta Hematol 17:237–46
- Dalton DK, Pitts-Meek S, Keshav S, et al. (1993). Multiple defects of immune cell function in mice with disrupted interferon-gamma genes. Science 259:1739–42
- Debray-Sachs M, Carnaud C, Boitard C, et al. (1991). Prevention of diabetes in NOD mice treated with antibody to murine IFN-γ. J Autoimmun 4:237–48
- Finney DJ. (1971). Probit Analysis, 3rd ed. New York: Cambridge University Press
- Foss-Freitas MC, Foss NT, Donadi EA, Foss MC. (2006). In vitro TNF-alpha and IL-6 production by adherent peripheral blood mononuclear cells obtained from type 1 and type 2 diabetic patients evaluated according to the metabolic control. Ann NY Acad Sci 1079:177–80
- Gray AM, Abdel-Wahab YH, Flatt PR. (2000). The traditional plant treatment, Sambucus nigra (elder) exhibits insulin-like and insulin-releasing actions in vitro. J Nutr 130:15–20
- Groot H, Rauen U. (1998). Tissue injury by reactive oxygen species and the protective effects of flavonoids. Fundam Clin Pharmacol 12:249–55
- Heinrich PC, Castell JV, Andus T. (1990). Interleukin-6 and the acute phase response. Biochem J 265:621–36
- Manach C, Scalbert A, Morand C, et al. (2004). Polyphenols: Food sources and bioavailability. Am J Clin Nutr 79:727–47
- Mavridis G, Souliou E, Diza E, et al. (2008). Inflammatory cytokines in insulin-treated patients with type 2 diabetes. Nutr Metab Cardiovasc Dis 18:471–6
- McNerlan SE, Rea IM, Alexander HD. (2002). A whole blood method for measurement of intracellular TNF-alpha, INF-gamma and IL-2 expression in stimulated CD3+ lymphocytes: Differences between young and elderly subjects. Exp Gerontol 37:227–34
- Mensah-Brown EP, Obineche EN, Galadari S, et al. (2005). Streptozotocin-induced diabetic nephropathy in rats: The role of inflammatory cytokines. Cytokine 31:180–90
- Nijveldt RJ, van Nood E, van Hoorn DE, et al. (2001). Flavonoids: A review of probable mechanisms of action and potential applications. Am J Clin Nutr 74:418–25
- Ozer G, Teker Z, Cetiner S, et al. (2003). Serum IL-1, IL-2, TNFalpha and INFgamma levels of patients with type 1 diabetes mellitus and their siblings. J Pediatr Endocrinol Metab 16:203–10
- Pascual-Teresa S, Sanchez-Ballesta MT. (2008). Anthocyanins: From plant to health. Phytochem Rev 7:281–99
- Pfeffer K, Matsuyama T, Kündig TM, et al. (1993). Mice deficient for the 55 kd tumor necrosis factor receptor are resistant to endotoxic shock, yet succumb to L. monocytogenes infection. Cell 73:457–67
- Pickup JC, Chusney GD, Thomas SM, Burt D. (2000). Plasma interleukin-6, tumour necrosis factor alpha and blood cytokine production in type 2 diabetes. Life Sci 67:291–300
- Rahman I, Biswas SK, Kirkham PA. (2006). Regulation of inflammation and redox signaling by dietary polyphenols. Biochem Pharmacol 72:1439–52
- Saltiel AR, Pessin JE. (2002). Insulin signaling pathways in time and space. Trends Cell Biol 12:65–71
- Scalbert A, Manach C, Morand C, et al. (2005). Dietary polyphenols and the prevention of diseases. Crit Rev Food Sci Nutr 45:287–306
- Singleton VL, Rossi JA. (1965). Colorimetry of total phenolics with phosphomolybdic–phosphotungstic acid reagents. Am J Enol Vitic 37:144–58
- Trompezinski S, Denis A, Schmitt D, Viac J. (2003). Comparative effects of polyphenols from green tea (EGCG) and soybean (genistein) on VEGF and IL-8 release from normal human keratinocytes stimulated with the proinflammatory cytokine TNF-α. Arch Dermatol Res 295:112–16
- Ursini F, Tubaro F, Rong J, Sevanian A. (1999). Optimization of nutrition: Polyphenols and vascular protection. Nutr Rev 57:241–9
- Yamagishi S, Inagaki Y, Okamoto T, et al. (2002). Advanced glycation end product-induced apoptosis and overexpression of vascular endothelial growth factor and monocyte chemoattractant protein-1 in human-cultured mesangial cells. J Biol Chem 277:20309–15
- Yao LH, Jiang YM, Shi J, et al. (2004). Flavonoids in food and their health benefits. Plant Foods Hum Nutr 59:113–22
- Yeh GY, Eisenberg DM, Kaptchuk TJ, Phillips RS. (2003). Systematic review of herbs and dietary supplements for glycemic control in diabetes. Diabetes Care 26:1277–94