Abstract
Context: Tagetes patula Linn. (Asteraceae) (French Marigold) flowers are used by local practitioners for cancer treatment; however, it lacks scientific justification.
Objective: Identification of bioactive compounds in T. patula flower for cytotoxic and growth inhibition in human cancer cell lines along with its antioxidant properties using chemical and cell based systems.
Materials and methods: The T. patula flower methanol extract, its seven fractions, and three phenolic compounds including methyl protocatechuate (1), patuletin (2), and patulitrin (3) were evaluated using sulforhodamine-B assay against HeLa, HT-144, NCI-H460, MCF-7, PC-3, and SF-268 human cancer cell lines. In parallel, antioxidant activity was evaluated using chemical (DPPH·, deoxyribose, and lipid peroxidation assays) and cell-based chemiluminescence systems (human neutrophils and mice macrophages).
Results: The methanol extract and ethyl acetate insoluble fraction exhibited cytotoxic and growth inhibitory effects against HeLa in which 2 exhibited highest cell growth inhibition (GI50: 0.6 ± 0.1 µg/ml) and cytotoxicity (LC50: 2.5 ± 0.1 µg/ml). It also scavenged LOO· (IC50: 6.5 ± 0.7 µg/ml) and (IC50: 27.5 ± 1.3 μg/ml) in chemical systems and human neutrophils, respectively. However, 1 preferably scavenged H2O2–Cl− (IC50: 0.5 ± 0.01 μg/ml) in mice macrophages.
Discussion and conclusion: Compound 2 from T. patula flower exhibited both growth inhibitory and cytotoxic properties while 1 and 3 were only growth inhibitory against HeLa. 1–3 also displayed antioxidant properties implying its probable role in growth inhibition/cytotoxic action. The present study provides scientific evidence for the use of T. patula flower in cancer treatment by traditional healer.
Introduction
Cancer is a leading cause of death and disability causing approximately 7.6 million deaths each year globally (Beaglehole et al., Citation2011) and is a major public health concern in many parts of the world. According to the World Health Organization (WHO) in 2009, about 750 million people died from cancer worldwide (Liu et al., Citation2012). Cervical cancer is the third most common cancer among women with 86% of global burden occurring in developing regions (De Sanjose et al., Citation2012). Although a number of chemotherapeutic agents are in clinical use against different types of cancers, however, undesirable side effects have been associated with them, e.g., cisplatin against cervical cancer (Zagouri et al., Citation2012) induces nephrotoxicity, paclitaxel exhibits musculoskeletal pain in breast and lung cancer patients (Heney et al., Citation2010), and vincristine used for leukemia is neurotoxic (Vilpo et al., Citation2000). The mechanism involved in cisplatin-induced nephrotoxicity is due to reduction of antioxidant status of kidney (Kilic et al., Citation2013) and vincristine neurotoxicity occurs via activation of glycogen synthase kinase-3 pathway by destabilization of microtubules that are abundant in nervous tissue (Alimoradi et al., Citation2012).
In spite of tremendous development in synthetic drugs, plants still constitute one of the major sources for modern drug development (Rahman et al., Citation2011). This is clearly reflected by many plant derived anticancer drugs, e.g., taxol from Taxus brevifolia Nuttall (Texaceae), vinblastine, and vincristine from Catharanthus roseus L. G. Don (Apocynaceae), and etoposide and teniposide from Podophyllum Linn (Berberidaceae) (Shoeb, Citation2006). The growing interest in plant natural products for anticancer activity is due to their secondary metabolites like terpenes, phenolics, and alkaloids (Dai & Mumper, Citation2010). There are many examples of plants rich in phenolic compounds that have demonstrated growth inhibitory and cytotoxic activities due to the presence of different types of flavonoids, e.g., quercetin, rutin, and apigenin in Brassica oleracea italica × albograbla against colon (SW480) and liver cancer (HepG2) cells (Wang & Zhang, Citation2011), quercetin, catechin, and fistein in Zingiber officinale against human breast cancer (MCF-7 and MDA-MB-231) (Rahman et al., Citation2011) and kaempferol, isorhamnetin, and rutin in Chenopodium quinoa against rat prostate cancer (AT-2) and human melanoma (HTB-140) cell lines (Dziki et al., Citation2013). The flavonoids quercetin, apigenin, and kaempferol have also demonstrated antitumor activity in the xenograft mouse model against human lung (Chan et al., Citation2013), colon (Shao et al., Citation2013), and osteosarcoma (Huang et al., Citation2010), respectively. The flavones flavopiridol and phenoxodiol are under clinical trials against prostate (Newcomb, Citation2004) and ovarian (Kelly et al., Citation2011) cancers. Most recently, a comprehensive review on Australian fruits (used as food and medicine by aboriginals) rich in phenolic compounds (apigenin, genestin, and gallic acid) demonstrated high antioxidant and antiproliferative properties associated with multiple mechanisms such as down regulation of NF-k B, pAKT, and inhibition of intrinsic mitochondrial pathway (Vuong et al., Citation2014). These phenolic compounds have been proposed as promising future anti-pancreatic cancer candidates emphasizing the folklore use of plants against cancer treatment.
Tagetes patula Linn. (Asteraceae) (French Marigold) is edible and used in folklore for the treatment of colics, diarrhea, vomiting, fever, skin, and hepatic diseases (Rondon et al., Citation2006). This plant possesses various biological properties including nematicidal (Faizi et al., Citation2011a), antibacterial, antifungal (Faizi et al., Citation2008), anti-inflammatory (Kasahara et al., Citation2002), analgesic, and antioxidant (Faizi et al., Citation2011b). Its phytochemical investigation revealed the presence of alkaloid (Faizi & Naz, Citation2002), flavonoids, thiophenes as well as terpenes (Vasudevan et al., Citation1997). A Pakistani scholar of international repute and traditional healer, Khawaja Shamsuddin Azeemi, is using T. patula flower petals in early morning under fasting condition for all types of cancer patients with promising results (Azeemi, Citation1999). Keeping this in mind, bioassay-directed cytotoxic and growth inhibitory activities of T. patula flower were investigated using human cancer cell lines and its antioxidant properties were evaluated using chemical and cellular antioxidant assays.
Materials and methods
Chemicals
1,1-Diphenyl-2-picryl-hydrazyl (DPPH), 2-deoxy-d-ribose, ascorbic acid, bovine brain extract Type VII, butylhydroxytoluene (BHT), dimethyl sulfoxide (DMSO), ethylene diaminetetraacetic acid (EDTA), ferric chloride (FeCl3), ficoll paque (lymphocyte separation medium), fetal bovine serum (FBS), l-glutamine penicillin–streptomycin solution (GPSS), Hank’s buffered saline solution (HBSS), hydrogen peroxide, lucigenin (bis-N-methyl-lacridinium nitrate), phorbol-12-myristate-13-acetate (PMA), potassium phosphate monobasic (KH2PO4), potassium phosphate dibasic (K2HPOċ3H2O), quercetin, Roswell Park Memorial Institute-1640 medium (RPMI-1640), sulforhodamine-B (SRB), rutin, thiobarbituric acid (TBA), trichloroacetic acid, trypsin-EDTA, and Tris base were obtained from Sigma (St. Louis, MO). Amphotericin-B (Forme Pharma, Karachi, Pakistan), gelatin (Scharlau, Barcelona, Spain), luminol (3-aminophthalhydrazide) (Alfa Aesar, Karlsruhe, Germany), and zymosan A (MP Biomedicals, Strasbourg, France) were purchased from respective companies.
Plant collection
Flowers of T. patula were collected during the months of September–January (2000–2003) from the University of Karachi campus. It was identified by Dr. Rubina Dawar of the Department of Botany, University of Karachi, Pakistan, and the voucher specimen (No. 67280) was deposited in the herbarium.
Extraction and isolation
Fresh, shade-dried, and uncrushed mix color flowers (5.2 kg) of T. patula were extracted three times with petroleum ether (PE) at room temperature (RT) (). The PE extract was evaporated in vacuo into a gummy residue (JFP). The remaining marc-1 was extracted with methanol and evaporated under reduced pressure to obtain dried residue (JFM). This was first partitioned between distilled water (D/W) and PE to give PE phase (JFM-P) followed by extraction of aqueous phase with dichloromethane (CH2Cl2), ethyl acetate (EtOAc), and butanol (BuOH). After a few days, insoluble matter deposited in the organic phases: JFM-P, JFM-DC, and JFM-EA (Faizi et al., Citation2008, Citation2011b).
The PE phase (JFM-P) was concentrated and decanted to obtain insoluble material (JFM-PI) and the decantate JFM-PD. The dichloromethane (CH2Cl2) phase was treated in the same way yielding the insoluble material (JFM-DC-I) and decantate soluble material (JFM-DC-D). Furthermore, needle-like crystals appeared in CH2Cl2 phases (JFM-DC-I), which were collected with spatula and dissolved in CHCl3:MeOH (1:1). The needle-like white crystals re-appeared which were filtered to give 70.3 mg of cyclooctasulfur S8 (TLC = RP-18, MeOH, Rf = 0.37, pink spot under UV-254). The ethyl acetate phases afforded yellow flakes on concentration at RT that were also decanted furnishing the insoluble material and decantate (JFM-EA-D). The JFM-EA-D was further concentrated at RT and filtered to give filtrate JFM-EA-DF and insoluble matter JFM-EA-DI. The methanol yellowish filtrate (JFM-EA-DF) on concentration provided yellow powdery matter (1.7 g) which was filtered and washed several times with PE and CH2Cl2 to furnish patuletin (2) (TLC = Rf = 0.28, CHCl3:MeOH 9:1). From the CH2Cl2 washing, methyl protocatechuate (1) was isolated as white crystals through preparative thin-layer chromatography (PTLC) (silica gel, CHCl3:MeOH, 9.5:0.5) (Faizi et al., Citation2008, Citation2011b). All the butanol phases were combined together and evaporated in vacuo to give a residue, JFM-Bu. A small fraction of JFM-Bu was treated with methanol and then filtered to give 0.1 g of patulitrin (3) () (Faizi et al., Citation2008, Citation2011b).
Identification of compounds
Compounds 1 (methyl protocatechuate), 2 (patuletin), and 3 (patulitrin) were identified and compared with chemical/physical data reported by NMR and mass spectrometry in the literature (Faizi et al., Citation2010, Citation2011b; Valant-Vetschera et al., Citation2003).
Preparation of samples
The stock solutions of plant extract (JFM), fractions (JFM-P, JFM-PI, JFM-DC-D, JFM-DC-I, JFM-EA-DF, JFM-EA-DI, and JFM-Bu) (40 mg/ml) and compounds 1, 2, and 3 and cisplatin (20 mM) were prepared in DMSO (100%) with a final concentration of DMSO not exceeding 0.5% (v/v) while doxorubicin (20 mM) in sterile (D/W). Further dilutions were prepared in RPMI-1640 medium. For antioxidant assays, stock solutions (1 mg/ml) of samples including extracts/fractions/pure compounds were prepared in methanol (100%). The samples were diluted in ethanol for DPPH assay and phosphate buffer (10 mM, KH2PO4 + K2HPO4, pH 7.4) for deoxy ribose and lipid peroxidation, and HBSS buffer was used in chemiluminescence assay.
Human cancer cell lines
Human cancer cell lines, NCI-H460 (large cell carcinoma, lung) MCF-7 (adenocarcinoma, breast), and SF-268 (anaplastic astrocytoma, CNS) were kindly provided by the National Cancer Institute (NCI), Frederick, MD. The HT-144 (melanoma, skin) was obtained from Biomedical and Genetic Engineering Division, KRL, Islamabad, Pakistan, while HeLa (uterine cervix) and PC-3 (prostate) were obtained from ATCC (American Type Culture Collection). The cancer cell lines were maintained in culture medium (15 ml) containing serum (FBS, 10% v/v), l-glutamine (1% w/v or 200 mM), penicillin (1% w/v, 104 U/ml), streptomycin (100 µg/ml), and antifungal amphotericin-B (10 µg/ml) in culture flasks (75 ml). These were kept in the CO2 incubator (5% CO2) at 37 °C till a confluent monolayer was formed within 3–4 d.
Bioassay
SRB assay
The in vitro cytotoxicity assay used was initially developed at NCI in 1990 for pre-clinical screening of natural products and synthetic compounds against a panel of 60 different human cancer cell lines. It is still most widely used assay as it provides information about both cell growth inhibition and cytotoxicity (Qamar et al., Citation2010; Skehan et al., Citation1990). The monolayer was trypsinized for 2–3 min with trypsin/EDTA (0.05%/0.02%) to obtain cell suspension. The cell concentration and viability were determined for each cell line using a hemocytometer. Cell suspension (100 µl/well) with appropriate cell number of HT-144 (2 × 104), MCF-7, PC-3, HeLa, NCI-H460 (1 × 104), and SF-268 (1.5 × 104) was added in each well of 96-well plates except the blank and incubated at 37 °C in 5% CO2 for 24 h. Test agents (100 µl) including T. patula extract (1–250 µg/ml), fractions (1–100 µg/ml), and pure compounds 1–3 (0.001–100 µM) along with anticancer drugs doxorubicin (0.001–10 µM) and cisplatin (0.1–100 µM) were added in the appropriate wells. The plates were then incubated for 48 h followed by fixation with ice-cold trichloroacetic acid (50 µl, 50%) for 30 min and washed with distilled water. The plates were air-dried overnight and stained with SRB (0.4%) for 30 min followed by the removal of unbound stain with acetic acid (1%). Tris-base (100 µl, 10 mM) was used to solubilize SRB stain and optical density was recorded spectrophotometrically at 515 nm. The GI50 (50% growth inhibition) and LC50 (50% cell death) indicating the sample concentration for 50% growth inhibition and cell death of total cells, respectively, were obtained graphically as concentration versus percent cell growth inhibition and cytotoxicity.
DPPH free radical-scavenging assay
The DPPH assay was conducted as described by Mensor et al. (Citation2001). The test agents (1.5 ml) including T. patula extract, fractions (2.5–100 µg/ml), pure compounds (2–50 µg/ml), and standard antioxidants, namely quercetin and rutin (2–20 µg/ml) were added in ethanolic DPPH solution (1 ml, 300 µM) in a total volume of 2.5 ml. After 30 min of incubation at RT, absorbance was recorded at 518 nm using a spectrophotometer (UV–vis spectrophotometer, Secomam, Alès Cedex, France). The IC50 values (concentration causing 50% DPPH radical-scavenging activity) were obtained graphically between the concentration versus percent free radical-scavenging activity.
Deoxyribose degradation assay
The assay was performed in a reaction mixture (500 µl) containing 250 µl of test agents (1–50 µg/ml), 2-deoxy-d-ribose (50 µl, 28 mM), EDTA and FeCl3 (1:1, 100 µl, 1 mM), and H2O2 and ascorbic acid each (50 µl, 1 mM). The reagents were mixed gently and incubated at 37 °C for 1 h. The control reaction contained equal volume of phosphate buffer instead of test agents. TBA (500 µl, 1% in 50 mM NaOH) and trichloroacetic acid (500 µl, 2.8 % in D/W) were added and the mixture was heated at 100 °C for 20 min. Upon cooling, the absorbance of pink chromogens generated was measured at 532 nm against a blank containing deoxyribose and phosphate buffer (Halliwell et al., Citation1987).
Lipid peroxidation assay
The test agents (150 µl, 1–100 µg/ml) were reacted with bovine brain extract (250 µl, 0.5 mg/ml), FeCl3, and ascorbic acid each (50 µl, 1 mM), whereas control contains equal volume of phosphate buffer in the place of test agents. After incubation at 37 °C, TBA, TCA, and butylhydroxytoulene (50 µl, 1% in methanol) were added and heated at 100 °C for 20 min followed by the addition of n-butanol (1.25 ml). The reaction mixture was centrifuged at 3000 rpm and an organic layer (top layer) was aspirated. The absorbance of this top layer was measured at 532 nm against a blank containing bovine brain phospholipids and phosphate buffer (Houghton et al., Citation1995).
Chemiluminescence assay
The scavenging activity of reactive oxygen species (ROS) generated intracellularly or extracellularly was measured using luminol and lucigenin chemiluminescence probes, respectively, as described by Helfand et al. (Citation1982). The test agents (25 µl, 0.5–100 µg/ml) were incubated at 37 °C either with human whole blood (25 µl, 1:20 dilution in HBSS) or human neutrophils or mice macrophages (25 µl, 1 × 106 cells/ml) for 15 min in a 96-well plate. The opsonized zymosan (25 µl, 3 mg/ml) or phorbol-myristate-acetate (25 µl, 1.3 × 10−7 M) was added as an intra- or extracellular stimulator of ROS, respectively. After addition of luminol (25 µl, 1.53 mM) or lucigenin (25 µl, 5 mM), chemiluminescence was measured on repeated scan mode nm in a luminescence reader as relative light units (RLUs).
Statistical analysis
One-way analysis of ANOVA followed by Duncan multiple comparisons was used to calculate differences between the control and treated groups. The p value <0.05*, <0.01**, and <0.001*** were considered significant.
Results
The methanol extract (JFM) and its fractions (JFM-P, JFM-PI, JFM-DC-D, JFM-DC-I, JFM-EA-DF, JFM-EA-DI, and JFM-Bu) along with three pure compounds, namely methyl protocatechuate (1), patuletin (2) and patulitrin (3) of T. patula flower were evaluated for growth inhibitory, cytotoxic, and antioxidant activities as described below.
Growth inhibitory and cytotoxic activity
The methanol extract (1–250 µg/ml) and its fractions (1–100 µg/ml) demonstrated growth inhibitory activity against all the human cancer cell lines used (). However, the methanol extract was most effective against HeLa cells showing both growth inhibitory (GI50: 7.3 ± 0.9 µg/ml) and cytotoxic properties (LC50: 37 ± 0.3 µg/ml) (). Among fractions, the ethyl acetate insoluble fraction (JFM-EA-DI) was consistently more effective against HeLa cell line (GI50: 3.5 ± 0.3 μg/ml and LC50: 25 ± 1.1 μg/ml). Its further fractionation yielded methyl protocatechuate (1) and two flavonoids, namely patuletin (2) and patulitrin (3). Among them, compound 2 was the most active exhibiting growth inhibitory as well as cytotoxic properties (GI50: 0.6 ± 0.1 µg/ml and LC50: 2.5 ± 0.1 µg/ml), whereas compound 1 (GI50: 9.3 ± 0.1 µg/ml) and 3 (GI50: 5 ± 2 µg/ml) demonstrated only growth inhibitory potential. The growth inhibition of doxorubicin and cisplatin (GI50: 0.35 ± 0.02 µg/ml and 1.2 ± 0.24 µg/ml) and their respective cytotoxicities (LC50: 4 ± 0.08 µg/ml and 15 ± 0.6 µg/ml) against HeLa cell line were also evaluated. The GI50 potency order appears to be doxorubicin = patuletin (2) = cisplatin > JFM-EA-DI > patulitrin (3) > JFM > methyl protocatechuate (1), whereas the potency order for LC50 was patuletin (2) > doxorubicin > cisplatin > JFM-EA-DI > JFM > patulitrin (3) = methyl protocatechuate (1).
Figure 3. The bar graph represents concentrations values of (a) GI50 (50% cell growth inhibition) and (b) LC50 (50% cell kill) of T. patula methanol extract (JFM) and its fractions (JFM-PI, JFM-P, JFM-DC-D, JFM-DC-I, JFM-EA-DF, JFM-EA-DI, and JFM-Bu) against PC-3 (





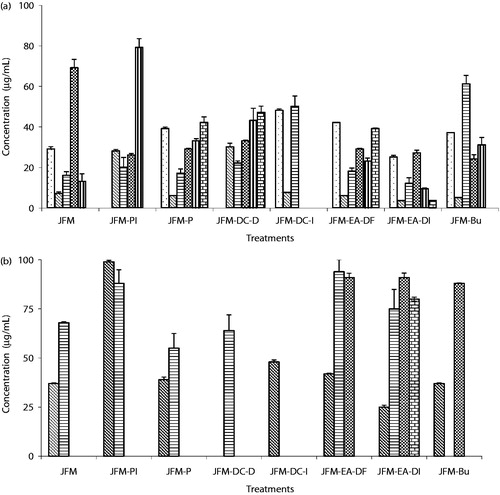
Table 1. Growth inhibitory and cytotoxic effects of extract, fraction and pure compounds of T. patula flower and standard anticancer agents against HeLa cell line.
Antioxidant activity
Free radical-scavenging activity was evaluated using (i) chemical assays including DPPH, deoxyribose degradation, and lipid peroxidation to scavenge 1,1-diphenyl-2-picryl-hydrazyl (DPPH•), hydroxyl (OH•), and peroxy radicals (LOO•) respectively, whereas (ii) cell-based chemiluminescence assay was applied using human whole blood, neutrophils, and mice macrophages for scavenging ROS including superoxide anion () and hydrogen peroxide–hypochlorite (H2O2–Cl)−.
Chemical-based free radical-scavenging activity
Against DPPH• radical, the methanol extract of T. patula flower exhibited IC50: 41 ± 0.8 µg/ml while its ethyl acetate insoluble fraction was about seven-times (∼7×) more potent (). Among pure compounds, methyl protocatechuate (1) (IC50: 2.1 ± 0.06 µg/ml) showed two-times (2×) and three-times (3×) more potent activity than patuletin (2) and patulitrin (3), respectively. In contrast, in the deoxyribose assay, the methanol extract (IC50: 5.8 ± 1.1 µg/ml) was more active than its ethyl acetate insoluble fraction (1.5×) in scavenging OH• while in the lipid peroxidation assay, again ethyl acetate insoluble fraction was 3× more potent than the methanol extract (IC50: 23 ± 3 µg/ml). Compound 1 was the most active scavenger of OH• (IC50: 2.75 ± 0.5 µg/ml) which exhibited ∼3× and ∼6× more potent effects than that of compounds 2 and 3, respectively. However, against LOO• radical, compound 2 (IC50: 6.5 ± 0.7 µg/ml) was ∼5× and ∼3× more active than 1 and 3, respectively (). Thus, potency order to scavenge DPPH• and OH• appears to be compound 1 >compound 2 > compound 3; whereas, in case of LOO• radical, the potency order appears to be compound 2 >compound 3 >compound 1.
Table 2. IC50 values representing DPPH•, OH•, and LOO• radicals scavenging activity of extract, fraction, and pure compounds of T. patula flower and standard antioxidant agents.
Cell-based (ROS)-scavenging activity
The methanol extract showed a similar potency (IC50: ∼30 µg/ml) in scavenging superoxide anion () in human neutrophils and hydrogen peroxide–hypochlorite (H2O2–Cl−) in mice macrophages whereas its ethyl acetate insoluble fraction was 2× more active against H2O2–Cl− but ineffective against
(). Among the pure compounds, methyl protocatechuate (1) was the most potent in scavenging H2O2–Cl− (IC50: 0.5 ± 0.01 µg/ml) in mouse macrophages and was 6× and 32× more potent than compounds 2 and 3, respectively (). Its activity to scavenge H2O2–Cl− in mouse macrophages was ∼8× more potent than those of widely used antioxidants, quercetin and rutin. In human neutrophils, patuletin (2) scavenged
(IC50: 27.5 ± 1.3 µg/ml) more actively than compound 1 (1.4×) while compound 3 was ineffective against superoxide (O2·_) radical.
Table 3. IC50 values representing H2O2–Cl− and
radicals scavenging effects of extract, fraction, and pure compounds of T. patula flower along with standard antioxidant agents.
Discussion
The Tagetes species have been reported earlier to possess cytotoxic activity as aqueous and ethanol extracts from aerial parts of T. lucida were effective against HeLa (GI50: 13.2 µg/ml and GI50: >50 µg/ml) and breast cancer (T47D) cell lines (GI50: 18.9 µg/ml and GI50: 1.82 µg/ml), respectively (Vega-Avila et al., Citation2009). Similarly, aqueous extract of T. minuta was effective against Lewis lung carcinoma of mouse C57BL (43% treated/control value at 266 mg/kg) (Ickes et al., Citation1973). In the present study, methanol extract of T. patula flower also exhibited growth inhibition and cytotoxicity against various human cancer cell lines including HT-144, HeLa, MCF-7, NCI-H460, SF-268, and PC-3; however, it was the most active (GI50: 7.3 ± 0.9 µg/ml and LC50: 37 ± 0.3 µg/ml) against HeLa as reflected by lowest GI50 and LC50 values suggesting Tagetes species sensitivity towards it. In HeLa cell line, bioassay-guided fractionation of methanol extract of T. patula flower afforded ethyl acetate-insoluble fraction that exhibited ∼2-fold better activity, whereas patuletin isolated from the same fraction showed profound growth inhibitory (GI50: 12× and 6×) and cytotoxic (LC50: 15× and 10×) effects compared with extract and fraction, respectively. This improvement of bioassay-guided growth inhibitory, and cytotoxic effects of T. patula methanol extract and ethyl acetate fraction are more likely due to the presence of phenolic compounds (flavonoids and phenolic acid) that prefer solvents of medium polarity (Hincapie et al., Citation2011). The ethyl acetate insoluble fraction of T. patula flower on purification also revealed major flavonoid, patuletin (2), and its 7-O-glucoside, patulitrin (3), along with an ester, methyl protocatechuate (1) (Faizi et al., Citation2008, Citation2010). The ethyl acetate fractions from other Tagetes spp., such as T. maxima (Parejo et al., Citation2005) and T. erecta (Valyova et al., Citation2012) were also reported to be flavonoid rich with high phenolic contents. There are also many examples of plants with polyphenol-rich methanol extracts such as Mangifera indica (Joona et al., Citation2013), Ipomoea pes-caprae (Parekh et al., Citation2012), and Ficus elastica Roxb exhibiting cytotoxic properties (EL-Hawary et al., Citation2012).
The comparison of patuletin with two clinically used drugs, doxorubicin and cisplatin, indicated that it was (2-fold) better growth inhibitor than cisplatin, a drug of choice in cervical cancer treatment (Zagouri et al., Citation2012) implying that patuletin is a promising cytotoxic molecule against cervical cancer derived from plants. In contrast, it was (1.6-fold) more cytotoxic than doxorubicin which is used against breast cancer (Prados et al., Citation2012). The cytotoxic studies of patuletin against normal human fetal lung fibroblast (MRC-5) cell line (GI50: 171 µg/ml) (Schmeda-Hirschmann et al., Citation2004) and intraperitoneal administration (100 mg/kg) in NMRI mice did not exhibit any mortality, also proven its safety (Faizi et al., Citation2011b) towards normal cells.
Patuletin shows certain features of flavonoids such as orthocatechol moiety in the B ring, unsaturated C2–C3 double bond, and hydroxylation at C-3 position that are crucial for flavonoid’s antiproliferative action (Kawaii et al., Citation1999). Additionally, the presence of a methoxy group at its C-6 position further increases its cytotoxic effect (Yanez et al., Citation2004) as methylation of hydroxyl groups in flavonoids improves lipophilicity (Heim et al., 2003) due to their enhanced entry into cells (Fiuza et al., Citation2004). A similar phenomenon has also been observed for quercetagetin (Qt), a characteristic flavonoid of genus Tagetes (Abdala, Citation2003) and a parent molecule of patuletin (6-methyl ether quercetagetin) lacking the methyl group which exhibited very weak antiproliferative activity against HeLa cell line (GI50: 168 µM) (Mori et al., Citation1988), while its polymethoxylated derivatives, centaureidin (trimethyl ether Qt, GI50: 0.082 µM) and casticin (tetramethyl ether Qt, GI50: 1.28 µM), showed better activities (Csupor-Loffler et al., Citation2009). Recently, polymethoxyflavone (naringenin) was found to be more cytotoxic as compared with their non-methylated analogues (Wesolowska et al., Citation2012). In our study, both patulitrin (3, a glucosidic patuletin) and methyl protocatechuate (1, phenolic acid with methyl ester) exhibited weak antiproliferative activity as compared with patuletin (2). The lower activity of 3 is possible due to O-glycosylation (replacement of OH moiety with glucose at C-7 position of patuletin) (Cao et al., Citation1997); while in the presence of methyl protocatechuate (1), this might be related to the shorter alkyl length (Fiuza et al., Citation2004) leading to reduced lipophilicity (Masaki et al., Citation1997).
It is widely accepted that cancer cells are under oxidative stress (Kobayashi & Suda, Citation2012) displaying higher ratios of ÷ H2O2 in human breast (2÷20×) and colon cancer cells (1.8÷10×) as compared with their normal cells (Aykin-Burns et al., Citation2009). H2O2 activates mitogen-activated protein kinase (MAPK) signaling pathway (Muller et al., Citation1997) and activator protein-1 (AP-1) transcription factor (Loo, Citation2003) which ultimately triggers cell proliferation (Reuter et al., Citation2010). These free radicals and ROS are neutralized or scavenged by phenolic compounds, thereby preventing the growth of cancer cells (Vuong et al., Citation2014). Also, plants rich in flavonoids have been reported to possess both antioxidant and cytotoxic activities (Prasad et al., Citation2009). Therefore, T. patula flower was evaluated for antioxidant properties against ROS.
The methanol extract and phenolic compounds 1 and 2 of T. patula flower attenuated the production of luminol and lucigenin-amplified chemiluminescence against human neutrophils and mice macrophages, respectively, which is dependent on the production of H2O2 and , respectively (Edwards, Citation1996; Dahlgren & Karlsson, Citation1999; Wymann et al., Citation1987). Also, in lucigenin-amplified chemiluminescence, neutrophils and macrophages are stimulated through protein kinase C (PKC) activation which is a cellular receptor for tumor-promoting phorbol ester (Blumberg, Citation1988) and produces mitogenic effects in early G1 cell cycle (Livneh & Fishman Citation1997) leading to cell proliferation (Nishizuka, Citation1992). In addition to cellular antioxidant effects, methyl protocatechuate (1) also actively scavenged DPPH· (IC50: 2.1 ± 0.06 μg/ml) and hydroxyl radical (IC50: ∼3 μg/ml), whereas patuletin (2) emerged as a potent scavenger of peroxy radical (IC50: 6.50 ± 0.7 μg/ml) in the chemical antioxidant system. This is in agreement with earlier report where ester of protocatechuic acid acted as the potent scavenger of hydroxyl ion (Yen et al., Citation2005); whereas patuletin scavenged peroxy radical (LOO·) possibly due to the presence of dihydroxy group at carbon 3′ and 4′ positions (catechol moiety), a property of most flavonoids (Cao et al., Citation1997).
The strong antioxidant properties of T. patula flower indicates that its antiproliferative and cytotoxic properties might be related to antioxidant potential of its phenolic compounds specially flavonoid patuletin. However, other mechanisms associated with flavonoid induced cytotoxicity such as cell-cycle arrest (Yanez et al., Citation2004), topoisomerase, and enzyme kinase inhibition (Bandele et al., Citation2008; Kanadaswami et al., Citation2005) cannot be ignored and evaluation of patuletin addressing them will allow better understanding of its cytotoxic mechanism of action.
Conclusion
The bioassay-guided activity of a methanol extract of T. patula flower led to the isolation of patuletin (2), a principal flavonoid, mainly responsible for its cytotoxic and growth inhibitory activities preferably towards cervical cancer (HeLa cell line). Its glucoside, patulitrin (3) and phenolic acid, methyl protocatechuate (1) also exhibited growth inhibition. One possible mechanism of their growth inhibitory or cytotoxic activities appears to be related with their antioxidant properties. Thus, the present study justifies the folkloric use of T. patula flower against cancer treatment.
Acknowledgements
We are thankful to the National Cancer Institute, Frederick, MD, for providing us cell lines under a Material Transfer Agreement.
Declaration of interest
The authors declare that there are no conflicts of interest. Ms. Samina Bano was financially supported through funds provided by Higher Education Commission, Islamabad, Pakistan, through the “Indigenous 5000 Fellowship Program”.
References
- Abdala LR. (2003). Chemosystematic interpretations of the flavonoids identified in Tagetes gracilis (Asteraceae). Biochem Syst Ecol 31:323–25
- Alimoradi H, Pourmohammadi N, Mehr SE, et al. (2012). Effects of lithium on peripheral neuropathy induced by vincristine in rats. Acta Med Iran 50:373–9
- Aykin-Burns N, Ahmad IM, Zhu Y, et al. (2009). Increased levels of superoxide and H2O2 mediate the differential susceptibility of cancer cells versus normal cells to glucose deprivation. J Biochem 418:29–37
- Azeemi KS. (1999). Rang-o-Roshni se Ilaj. Karachi: Al-Kitab Publications, 42–3. Available from: http://urdunovelsbookspdfdownload.blogspot.com/2013/09/rang-or-roshni-say-ilaj-by-khawaja.html [last accessed 6 Feb 2014]
- Bandele OJ, Clawson SJ, Osheroff N. (2008). Dietary polyphenols as topoisomerase II poisons: B ring and C ring substituents determine the mechanism of enzyme-mediated DNA cleavage enhancement. Chem Res Toxicol 21:1253–60
- Beaglehole R, Bonita R, Magnusson R. (2011). Global cancer prevention: An important pathway to global health and development. Public Health 125:821–31
- Blumberg PM. (1988). Protein kinase C as the receptor for the phorbol ester tumor promoters: Sixth Rhoads memorial award lecture. Cancer Res 48:1–8
- Cao G, Sofic E, Prior RL. (1997). Antioxidant and prooxidant behavior of flavonoids: Structure activity relationships. Free Radical Biol Med 22:749–60
- Chan ST, Yang NC, Huang CS, et al. (2013). Quercetin enhances the antitumor activity of trichostatin A through upregulation of p53 protein expression in vitro and in vivo. PLoS One 8:e54255
- Csupor-Loffler B, Hajdu Z, Zupko I, et al. (2009). Antiproliferative effect of flavonoids and sesquiterpenoids from Achillea millefolium s.I. on cultured human tumor cell lines. Phytother Res 23:672–6
- Dahlgren C, Karlsson A. (1999). Respiratory burst in human neutrophils. J Immunol Methods 232:3–14
- Dai J, Mumper RJ. (2010). Plant phenolics: Extraction, analysis and their antioxidant and anticancer properties. Molecules 15:7313–52
- De Sanjose S, Serrano B, Castellsague X, et al. (2012). Human papillomavirus (HPV) and related cancers in the global alliance for vaccines and immunization (GAVI) countries. A WHO/ICO HPV information centre report. Vaccine 30:1–84
- Dziki UG, Sweica M, Sulkowski M, et al. (2013). Antioxidant and anticancer activities of Chenopodium quinoa leaves extracts: In vitro study. Food Chem Toxicol 57:154–60
- Edwards SW. (1996). The O-2 generating NADPH oxidase of phagocytes: Structure and methods of detection. Methods 9:563–77
- EL-Hawary SS, Wassel GM, EL-Manshawi BS, et al. (2012). Antitumor and antioxidant activity of Ficus elastica Roxb and Ficus bengalensis Linn. family Moraceae. World Appl Sci J 19:1532–9
- Faizi S, Naz A. (2002). Jafrine: A novel and labile β-carboline alkaloid from the flowers of Tagetes patula. Tetrahedron 58:6185–97
- Faizi S, Dar A, Siddiqi H, et al. (2011b). Bioassay guided isolation of antioxidant agents with analgesic properties from flowers of Tagetes patula. Pharm Biol 4:516–25
- Faizi S, Fayyaz S, Bano S, et al. (2011a). Isolation of nematicidal compounds from Tagetes patula L. yellow flowers: Structure–activity relationship studies against cyst nematode Heterodera zeae infective stage larvae. J Agric Food Chem 59:9080–93
- Faizi S, Siddiqi H, Naz A, et al. (2008). Antibacterial and antifungal activities of different parts of Tagetes patula: Preparation of patuletin derivatives. Pharm Biol 46:309–20
- Faizi S, Siddiqi H, Naz A, et al. (2010). Specific deuteration in patuletin and related flavonoids via keto-enol tautomerism: Solvent and temperature dependent 1H-NMR studies. Helv Chim Acta 93:466–81
- Fiuza SM, Gomes C, Teixeira LJ, et al. (2004). Phenolic acid derivatives with potential anticancer properties – A structure-activity relationship study. Part 1: Methyl, propyl and octyl esters of caffeic and gallic acids. Bioorg Med Chem 12:3581–9
- Halliwell B, Gutteridge JMC, Aruoma OL. (1987). The deoxyribose method: A simple test tube assay for determination of rate constants for reaction of hydroxyl radicals. Anal Biochem 165:215–19
- Heim KE, Tagliaferro AR, Bobilya DJ. (2002). Flavonoid antioxidants: Chemistry, metabolism and structure–activity relationships. J Nutr Biochem 13:572–84
- Helfand S, Werkmeister J, Roder JC. (1982). Chemiluminescence response of human natural killer cells. The relationship between target cell binding, chemiluminescence and cytolysis. J Exp Med 156:492–505
- Heney M, Alipour M, Vergidis D, et al. (2010). Effectiveness of liposomal paclitaxel against MCF-7 breast cancer cells. Can J Physiol Pharmacol 88:1172–80
- Hincapie CA, Monsalve Z, Seigler DS, et al. (2011). Antioxidant activity of Blechnum chilense (Kaulf.) Mett Curcuma domestica Valeton and Tagetes verticillata Lag and Rodriguez. Plant Med Aromat 10:315–24
- Houghton PJ, Zarka R, de la Heras B, Hoult JRS. (1995). Fixed oil of Nigella sativa and derived thymoquinone inhibit eicosanoid generation in leukocytes and membrane lipid peroxidation. Planta Med 61:33–6
- Huang WW, Chiu YJ, Fan MJ, et al. (2010). Kaempferol induced apoptosis via endoplasmic reticulum stress and mitochondria-dependent pathway in human osteosarcoma U-2 OS cells. Mol Nutr Food Res 54:1585–95
- Ickes GR, Fong HHS, Perdude RE, Farnsworth NR. (1973). Antitumor activity and preliminary phytochemical examination of Tagetes minuta (Compositae). J Pharm Sci 62:1009–11
- Joona K, Sowmia C, Dhanya KP, Divya MJ. (2013). Preliminary phytochemical investigation of Mangifera indica leaves and screening of antioxidant and anticancer activity. Res J Pharm Biol Chem Sci 4:1112–18
- Kanadaswami C, Lee LT, Lee PPH, et al. (2005). The antitumor activities of flavonoids. In vivo 19:895–910
- Kasahara Y, Yasukawa K, Kitanaka S, et al. (2002). Effect of methanol extract from flower petals of Tagetes patula L. on acute and chronic inflammation model. Phytother Res 16:217–22
- Kawaii S, Tomono Y, Katase E, Ogawa K. (1999). Antiproliferative activity of flavonoids on several cancer cell lines. Biosci Biotechnol Biochem 63:896–9
- Kelly MG, Mor G, Husband A, et al. (2011). Phase II evaluation of phenoxodiol in combination with cisplatin or paclitaxel in women with platinum/taxane-refractory/resistant epithelial ovarian, fallopian tube, or primary peritoneal cancers. Int J Gynecol Cancer 21:633–9
- Kilic U, Kilic E, Tuzcu Z, et al. (2013). Melatonin suppresses cisplatin-induced nephrotoxicity via activation of Nrf-2/HO-1 pathway. Nutr Metab 10:7
- Kobayashi CI, Suda T. (2012). Regulation of reactive oxygen species in stem cells and cancer stem cells. J Cell Physiol 227:421–30
- Liu X, Wei G, Yang D, et al. (2012). Possibility to partly win the war against cancer. In: Liu X, Pestka S, Shi Y, eds. Recent Advances in Cancer Research and Therapy. London: Elsevier Science, 617–71
- Livneh E, Fishman DD. (1997). Linking protein kinase C to cell-cycle control. Eur J Biochem 248:1–9
- Loo G. (2003). Redox-sensitive mechanisms of phytochemical-mediated inhibition of cancer cell proliferation (review). J Nutr Biochem 14:64–73
- Masaki H, Okamoto N, Sakaki S, Sakurai H. (1997). Protective effects of hydroxybenzoic acids and their esters on cell damage induced by hydroxyl radicals and hydrogen peroxides. Biol Pharm Bull 20:304–8
- Mensor LL, Menezes FS, Leitaõ GG, et al. (2001). Screening of Brazilian plant extracts for antioxidant activity by the use of DPPH free radical method. Phytother Res 15:127–30
- Mori A, Nishino C, Enoki N, Tawata S. (1988). Cytotoxicity of plant flavonoids against HeLa cells. Phytochemistry 27:1017–20
- Muller JM, Cahill MA, Rupec RA, et al. (1997). Antioxidants as well as oxidants activate c-fos via Ras-dependent activation of extracellular-signal-regulated kinase 2 and Elk-1. Eur J Biochem 244:45–52
- Newcomb EW. (2004). Flavopiridol: Pleiotropic biological effects enhance its anti-cancer activity. Anticancer Drugs 15:411–19
- Nishizuka Y. (1992). Intracellular signaling by hydrolysis of phospholipids and activation of protein kinase C. Science 258:607–14
- Parejo I, Bastida J, Viladomat F, Codina C. (2005). Acylated quercetagetin glycosides with antioxidant activity from Tagetes maxima. Phytochemistry 66:2356–62
- Parekh KK, Patel AM, Modi AJ, Chandrashekhar HR. (2012). Antioxidant and cytotoxic activities of few selected Ipomoea species. Pharmacologia 3:377–86
- Prados J, Melguizo C, Ortiz R, et al. (2012). Doxorubicin-loaded nanoparticles: New advances in breast cancer therapy. Anticancer Agents Med Chem 12:1058–70
- Prasad KN, Hao J, Shi J, et al. (2009). Antioxidant and anticancer activities of high pressure assisted extract of longan (Dimocarpus longan Lour.) fruit pericap. Innov Food Sci Emerg 10:413–19
- Qamar KA, Dar A, Siddiqui BS, et al. (2010). Anticancer activity of Ocimum basilicum and the effect of ursolic acid on the cytoskeleton of MCF-7 human breast cancer cells. Lett Drug Des Discov 7:726–36
- Rahman S, Salehin F, Iqbal A. (2011). In vitro antioxidant and anticancer activity of young Zingiber officinale against human breast cancer cell lines. BMC Complement Altern Med 11:76
- Reuter S, Gupta SC, Chaturvedi MM, Aggarwal BB. (2010). Oxidative stress, inflammation, and cancer: How are they linked? Free Radic Biol Med 49:1603–16
- Rhama S, Madhavan S. (2011). Antibacterial activity of the flavonoid, patulitrin isolated from the flowers of Tagetes erecta L. Int J Pharm Tech Res 3:1407–9
- Rondon M, Velasco J, Hernandez J, et al. (2006). Chemical composition and antibacterial activity of the essential oil of Tagetes patula L. (Asteraceae) collected from the Venezuela Andes. Rev Latinoam Quim 34:32–6
- Schmeda-Hirschmann G, Tapia A, Theoduloz C, et al. (2004). Free radical scavengers and antioxidants from Tagetes mendocina. J Biosci 59:345–53
- Shao H, Jing K, Mahmoud E, et al. (2013). Apigenin sensitizes colon cancer cells to antitumor activity of ABT-263. Mol Cancer Ther 12:2640–50
- Shoeb M. (2006). Anticancer agents from medicinal plants. Bangladesh J Pharmacol 1:35–41
- Skehan P, Storeng R, Scudiero D, et al. (1990). New colorimetric cytotoxicity assay for anti-cancer drug screening. J Natl Cancer Inst 82:1107–12
- Valant-Vetschera KM, Wollenweber E, Faure R, Gaydou E. (2003). New exudate flavonoids of species from the Chrysanthemum complex (Asteraceae–Anthemideae). Biochem Syst Ecol 31:545–8
- Valyova MS, Stoyanov S, Markovska Y, Ganeva Y. (2012). Evaluation of in vitro antioxidant activity and free radical scavenging potential of variety of Tagetes erecta L. flowers growing in Bulgaria. Int J Appl Res Nat Prod 5:19–25
- Vasudevan P, Kashyap S, Sharma S. (1997). Tagetes: A multipurpose plant. Bioresour Technol 62:29–35
- Vega-Avila E, Espejo-Serna A, Alarcon-Aguilar F, Valasco-Lezama R. (2009). Cytotoxic activity of four Mexican medicinal plants. Proc West Pharmacol Soc 52:78–82
- Vilpo JA, Koski T, Vilpo LM. (2000). Selective toxicity of vincristine against chronic lymphocytic leukemia cells in vitro. Eur J Haematol 65:370–8
- Vuong QV, Hirun S, Phillips PA, et al. (2014). Fruit-derived phenolic compounds and pancreatic cancer: Perspectives from Australian native fruits. J Ethnopharmacol 152:227–42
- Wang B, Zhang X. (2011). Inhibitory effects of Brocoli leaf flavonoids on human cancer cells. Scanning 33:1–5
- Wesolowska O, Wisniewski J, Sroda-Pomianek K, et al. (2012). Multidrug resistance reversal and apoptosis induction in human colon cancer cells by some flavonoids present in citrus plants. J Nat Prod 75:1896–902
- Wymann MP, von Tscharner V, Deranleau DA, Baggiolini M. (1987). Chemiluminescence detection of H2O2 produced by human neutrophils during the respiratory burst. Anal Biochem 165:371–8
- Yanez J, Vicente V, Alcaraz M, et al. (2004). Cytotoxicity and antiproliferative activities of several phenolic compounds against three melanocytes cell lines: Relationship between structure and activity. Nutr Cancer 49:191–9
- Yen WJ, Chang LW, Duh PD. (2005). Antioxidant activity of peanut seed tests and its antioxidative component, ethyl protocatechuate. LWT 38:193–200
- Zagouri F, Sergentanis TN, Chrysikos D, et al. (2012). Molecularly targeted therapies in cervical cancer. A systematic review. Gynecol Oncol 126:291–303