Abstract
Context: meso-Zeaxanthin (MZ) is a xanthophyll carotenoid with profound antioxidant activity.
Objective: Oxidative stress plays a decisive role in numerous degenerative diseases including cancer. The present study evaluates anti-inflammatory effect of MZ.
Materials and methods: Balb/c mice were treated with different doses of MZ (50 and 250 mg/kg b.wt, orally) 5 d before subcutaneous injection of carrageenan (1%), dextran (1%), and formalin (2%). Paw edema formation in MZ-treated and -untreated animals was measured using vernier calipers. Anti-inflammatory activity of MZ against lipopolysaccharide (LPS)-induced inflammatory model was studied by culturing macrophages in the presence and absence of LPS (5 μg/ml) and different concentrations of MZ (5, 10, and 25 μg/ml). After 24 h, the effect of MZ on pro-inflammatory cytokine levels in macrophages was analyzed by ELISA and its effect on various inflammatory genes was studied by RT-PCR.
Results: MZ administration at different doses significantly (p < 0.001) inhibited paw edema induced by carrageenan, dextran, and formalin in mice. MZ also exhibited profound anti-inflammatory effect against LPS-induced inflammation in macrophages. Increased production of nitric oxide, C-reactive proteins, and various pro-inflammatory cytokines (TNF-α, interleukin-1β, and interleukin-6) in LPS-stimulated macrophages was significantly reduced by MZ treatment. Moreover, LPS-stimulated up-regulated mRNA expression of various inflammatory mediator genes like COX-2, TNF-α, and iNOS was down-regulated by MZ administration.
Discussion and conclusion: MZ has potent anti-inflammatory effect which can be due to its down-regulated expression of various inflammatory mediator genes. Since cancer is considered as an inflammatory disease, the present study points towards the importance of MZ in chemo-preventive strategy.
Introduction
The link between inflammation and cancer is generally accepted. Inflammation is known as a part of host's innate immune defense mechanism and it occurs in response to tissue damage resulting from microbial pathogen infection, chemical irritation, and/or wounding (Grivennikov et al., Citation2010). Chronic inflammatory responses play a major role in the progression of several diseases like cancer, atherosclerosis, diabetes, obesity, and Alzheimer's disease (Licastro et al., Citation2005). About 20% of all human cancers are caused by chronic inflammatory states. Biochemical processes which are altered during chronic inflammation have been implicated in tumorigenesis. These include shifting of cellular redox balance towards oxidative stress, increased DNA damage, genomic instability, stimulation of cell proliferation, angiogenesis, and metastasis. Chronically inflamed tissue is infiltrated with a variety of inflammatory cells that produce a wide variety of bioactive chemicals such as cytokines, growth factors, reactive oxygen, and nitrogen species and products formed from cyclooxygenase, and lipoxygenase activities. Generally, there is a high risk of cancer if the inflammation persists for a long time (Coussens & Werb, Citation2002). A growing interest is seen in the pharmacological evaluation of various phytochemicals to assess their anti-inflammatory activity.
Carotenoids, which belong to the chemical group known as isoprenoid polyenes, are lipid-soluble yellow–orange–red pigments found in all higher plants and in some animals. Light capture, photosynthesis photoprotection, excess light dissipation, and quenching of singlet oxygen are among the key biological functions of carotenoids relevant for life on earth. Carotenoids exhibit various pharmacological activities including antioxidant activity, immuno enhancement, inhibition of mutagenesis, and regression of premalignant lesions. Hence, recent interest in carotenoids has been mainly for their nutraceutical properties. The biological properties of carotenoids have a wide range of commercial applications.
meso-Zeaxanthin [(3R, 3′S)-β, β-carotene-3, 3′-diol, MZ] is one of the macular carotenoids with profound antioxidant activity. MZ is present in the Henle fibers (receptor axons) of primates' retina. Previous studies on MZ reported that its supplementation reduced age-related macular degeneration. Commercially, MZ is prepared from marigold flower extract. It is non-toxic (Chang, Citation2006) and, in 2011, FDA acknowledged that MZ synthesized from marigold flower extract is Generally Regarded as Safe (GRAS) and is eligible for use in dietary supplements. Our previous studies have shown that MZ synthesized from marigold flower extract has antioxidant (Firdous et al., Citation2010), anti-mutagenic (Firdous et al., Citation2011), chemoprotective (Firdous & Kuttan, Citation2012), radioprotective (Firdous et al., Citation2013a), and chemopreventive properties (Firdous et al., Citation2013b). The present study has been undertaken with the aim to determine anti-inflammatory activity of carotenoid MZ and its possible mechanism of action.
Materials and methods
Chemicals
Rosewell Park Memorial Institute medium (RPMI-1640), dextran, and carrageenan were purchased from Sigma Aldrich (St. Louis, MO). Lipopolysaccharide (LPS) was bought from Difco Laboratories, Abbott Park, IL. Fetal calf serum (FCS) was obtained from Biological Industries, Kibbutz, Israel. Oligo nucleotide primer sequences () were purchased from Ambion Inc, Austin, TX. Specific quantitative sandwich ELISA kits for mouse tumor necrosis factor alpha (TNF-α), interleukin 1β (IL-1β), interleukin 6 (IL-6), and C-reactive protein were purchased from Endogen Inc, Rockford, IL. Cell-to-cDNA Kit was bought from Ambion Inc, Austin, TX. All other chemicals and reagents used were of analytical grade.
Table 1. Oligonucleotide primer sequences.
Animals
Male Balb/c mice (6–8 week old, 25 ± 3 g b.wt) were purchased from Small Animal Breeding Station, Kerala Agricultural University, Thrissur, Kerala, India. They were housed in well-ventilated cages with controlled conditions of light and humidity. They were provided with normal mouse chow (Sai Durga Food and Feeds, Bangalore, India) and water ad libitum. All the animal experiments were performed as per the instructions prescribed by the Committee for the Purpose of Control and Supervision of Experiments on Animals (CPCSEA), Ministry of Environment and Forest, Government of India and implemented through the Institutional Animal Ethical Committee of Amala Cancer Research Centre (Registration number: 149/1999/CPCSEA).
Sample
meso-Zeaxanthin used in this study was supplied by Omni Active Health Technologies Ltd, Mumbai, India. A 5% suspension of MZ was prepared in sunflower oil and was stored in dark bottles at 4 °C to prevent oxidation.
Determination of anti-inflammatory activity of MZ against carrageenan-induced paw edema model
Male Balb/c mice were divided into six groups consisting of six animals in each group:
Group I: Carrageenan alone treated (control)
Group II: Carrageenan + sunflower oil (vehicle control)
Group III: Carrageenan + MZ (50 mg/kg b.wt)
Group IV: Carrageenan + MZ (100 mg/kg b.wt)
Group V: Carrageenan + MZ (250 mg/kg b.wt)
Group VI: Carrageenan + diclofenac-10 mg/kg b.wt (standard)
MZ at different concentrations or diclofenac (10 mg/kg b.wt) was administered orally for 5 consecutive days. On fifth day, 1 h after the administration MZ acute inflammation was induced in the hind paw of mice by sub-plantar injection of 0.02 ml of freshly prepared 1% suspension of carrageenan in 0.1% carboxy methyl cellulose (CMC) (Winter et al., Citation1962). The paw thickness was measured using vernier calipers and recorded every hour up to sixth hour. The percentage of inhibition was calculated according to the following formula:
where tCn is the paw thickness of control animal at particular time point after injection of inflammatory agent. tC0 is the paw thickness of control animal before injection of inflammatory agent. tTn is the paw thickness of treated animal at particular time point after injection of inflammatory agent. tT0 is the paw thickness of treated animal before injection of inflammatory agent.
Determination of anti-inflammatory activity of MZ against dextran-induced paw edema model
Male Balb/c mice were divided into six groups consisting of six animals in each group:
Group I: Dextran alone treated (control)
Group II: Dextran + sunflower oil (vehicle control)
Group III: Dextran + MZ (50 mg/kg b.wt)
Group IV: Dextran + MZ (100 mg/kg b.wt)
Group V: Dextran + MZ (250 mg/kg b.wt)
Group VI: Dextran + Diclofenac-10 mg/kg b.wt (standard)
MZ or diclofenac was administered orally for 5 consecutive days. On fifth day, 1 h after MZ administration acute inflammation was induced in the hind paw of mice by sub-plantar injection of 0.02 ml of freshly prepared 1% suspension of dextran in 0.1% carboxy methyl cellulose (CMC) (Maity et al., Citation1988). The paw thickness was measured using vernier calipers and recorded every hour up to sixth hour. The percentage of inhibition was calculated according to the above formula.
Determination of anti-inflammatory activity of MZ against formalin-induced chronic inflammatory model
Male Balb/c mice were divided into six groups (six animals per group):
Group I: formalin alone treated (control)
Group II: formalin + sunflower oil (vehicle control)
Group III: formalin + MZ (50 mg/kg b.wt)
Group IV: formalin + MZ (100 mg/kg b.wt)
Group V: formalin + MZ (250 mg/kg b.wt)
Group VI: formalin + diclofenac-10 mg/kg b.wt (standard)
MZ or diclofenac was administered orally for 5 consecutive days. On the fifth day, 1 h after MZ treatment chronic inflammation was induced in the right hind paw of mice by sub-plantar injection of 0.02 ml of freshly prepared 2% formalin (Chau, Citation1989). The paw thickness of all the animals was measured using vernier calipers for 6 consecutive days after the injection of formalin. The percentage of inhibition was calculated according to the formula given above.
Determination of the effect of MZ on the production of C-reactive protein and various pro-inflammatory cytokines in LPS-stimulated macrophages
Macrophages were elicited by injecting 5% sodium caseinate intraperitoneally in Balb/c mice. Macrophages were washed with PBS and resuspended in RPMI 1640 with 10% FCS. The cells were plated in 96-well culture plates and incubated for 2 h at 37 °C in a 5% CO2 atmosphere. After incubation, non-adherent cells were removed and adherent macrophages were incubated (2 × 106 cells/well) in complete medium [RPMI 1640, 10% FCS, streptomycin (100 μg/ml), penicillin (100 U/ml), and 2 mM glutamine]. The macrophages were cultured with or without LPS (5 μg/ml) in the presence and absence of MZ (5, 10, and 25 μg/ml) for 24 h at 37 °C in 5% CO2 atmosphere. After 24 h, the plates were centrifuged and the supernatant was collected. The supernatant was used for the estimation of C-reactive protein and various pro-inflammatory cytokines like TNF-α, IL-1β, and IL-6 by the ELISA method. The experiment was repeated three times with each concentration in triplicate.
Determination of the effect of MZ on the production of nitric oxide in LPS-stimulated macrophages
The supernatant obtained in the above experiment was used for the estimation of nitric oxide (NO) using the Griess reagent (Green et al., Citation1982). In this method, 100 μl of supernatant was mixed with an equal amount of the Griess reagent (1% sulfanilamide, 2% ortho-phosphoric acid, and 0.1% naphthyl ethylene diamine dihydrochloride) and incubated for 10 min at room temperature. The absorbance was estimated at 540 nm.
Determination of the effect of MZ on the expressions of inducible nitric oxide synthase (iNOS), cyclooxygenase 2 (COX-2), and TNF-α genes in LPS-stimulated macrophages
Macrophages obtained from the above experiment were used to study the expression of inflammatory mediators at the mRNA level. cDNA was synthesized from the macrophages using cell to cDNA kit. Briefly, cells from tissue culture plates were washed in PBS. Ice cold cell lysis buffer (100 μl) was added to the cells and incubated for 15 min at 75 °C in a water bath. The cell lysates were then transferred to nuclease-free microcentrifuge tubes. DNase-1 (2 μl) was added to these tubes and incubated for 15 min at 37 °C. DNase was inactivated by heating at 75 °C for 5 min. Then the cell lysate was mixed with dNTP mix, Oligo (dT)18, M-MLV reverse transcriptase, and RNase inhibitor. The reaction mixture was then subjected to reverse transcription reaction by using a thermocycler. The cDNA prepared by this method was amplified using specific primers for TNF-α, iNOS, COX-2, and the house keeping gene GAPDH in RT-PCR. The experiment was repeated thrice to check the reproducibility of the results.
Statistical analysis
The values were expressed as mean ± standard deviation (SD). The mean values were statistically analyzed by one-way analysis of variance (ANOVA) followed by the appropriate post hoc test (Dunnett's multiple comparison test) using Graphpad Instat 3 Software (Graph Pad Software, Inc., La Jolla, CA). p Value < 0.05 was considered to be statistically significant.
Results
Effect of MZ on the carrageenan-induced inflammatory model
Carrageenan injection leads to a progressive increase in paw thickness of mice. Carrageenan-induced paw edema was significantly reduced by MZ administration. At the third hour, there was 62% of inhibition of paw edema formation in animals treated with MZ (250 mg/kg b.wt). Administration of MZ at doses 50 and 100 mg/kg b.wt produced 33 and 43% of inhibition, respectively, at the third hour (). The inhibition of paw edema formation in animals treated with standard drug, i.e., diclofenac (10 mg/kg b.wt) was 51%.
Effect of MZ on the dextran-induced inflammatory model
Administration of MZ significantly reduced dextran-induced paw edema formation. In mice treated with MZ at doses of 50, 100, and 250 mg/kg b.wt, the reduction of the paw thickness was 40, 48, and 59%, respectively, at the third hour (). The percentage of inhibition in the diclofenac-treated group was 57%.
Effect of MZ on the formalin-induced chronic inflammatory model
Chronic inflammation induced by formalin was also found to be inhibited by MZ administration (). The control animals showed a maximum paw thickness of 0.419 cm on the third day after formalin administration. However, in MZ-treated animals, there was a significant reduction in paw edema. On the third day after oral administration of MZ, the percentage of inhibition of paw edema was 40% (50 mg/kg b.wt), 44% (100 mg/kg b.wt), and 58% (250 mg/kg b.wt). The percentage of inhibition of standard drug, i.e., diclofenac was 53%. Moreover, there was a decrease in the erythema produced by formalin injection by administration of MZ.
Effect of MZ on the production of various pro-inflammatory cytokines in LPS-stimulated macrophages
MZ significantly inhibited the production of C-reactive protein and pro-inflammatory cytokines like TNF-α, IL-1β, and IL-6 in LPS-stimulated macrophages. The levels of TNF-α, IL-1β, C-reactive protein, and IL-6 were markedly increased by treatment with LPS. The increased levels of pro-inflammatory cytokines and C-reactive protein were significantly (p < 0.001) reduced by the administration of 25 μg/ml of MZ ().
Table 2. Effect of MZ on the production of C-reactive protein and various proinflammatory cytokines by LPS-stimulated macrophages.
Effect of MZ on NO production in LPS-stimulated macrophages
MZ inhibited NO production in LPS-stimulated macrophages in a statistically significant manner. The production of NO in macrophages was found to be markedly elevated after 24 h of LPS treatment when compared with the normal macrophages. The elevated levels were significantly reduced by MZ treatment in a concentration-dependent manner ().
Table 3. Effect of MZ on the production of NO by LPS-stimulated macrophages.
Effect of MZ on the expression of TNF-α, iNOS and COX-2 genes in LPS-stimulated macrophages
RT-PCR analysis was performed to determine the effect of MZ on the expression of inflammatory mediators such as iNOS and COX-2, and pro-inflammatory cytokine TNF-α. In unstimulated macrophages, TNF-α, iNOS, and COX-2 mRNA were expressed in low levels. In response to LPS, the expression of TNF-α, iNOS, and COX-2 genes were markedly up-regulated. Treatment with MZ significantly down-regulated the expressions TNF-α, iNOS, and COX-2 in LPS-stimulated macrophages ().
Figure 4. Effect of MZ on the expression of TNF-α, iNOS and COX-2 genes in LPS-stimulated macrophages. Lane 1 – Expression of genes in macrophages; Lane 2 – up-regulated expression of genes in LPS-stimulated macrophages; Lane 3 – Down-regulated expression of proinflammatory genes in MZ-treated LPS-stimulated macrophages.
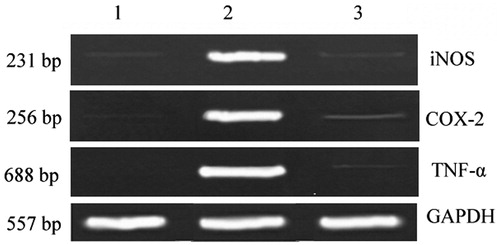
Discussion
Inflammation acts at all stages of tumorigenesis. It may contribute to tumor initiation through mutation, genomic instability, and epigenetic modifications. Inflammation activates tissue repair responses, induces proliferation of premalignant cells, and enhances their survival. Inflammation also stimulates angiogenesis, causes localized immunosuppression, and promotes metastatic spread as well. The key molecular mediators that link inflammation to genetic alterations are prostaglandins, cytokines, chemokines, and angiogenic factors (Yoshimura, Citation2006).
Presently, the anti-inflammatory effect of MZ was studied using carrageenan, dextran, and formalin-induced paw edema models in mice. Carrageenan is a mucopolysaccharide isolated from the Irish Sea moss Chondruscrispus. Carrageenan-induced edema formation is biphasic. The early phase (1–2 h) is mainly mediated by histamine, serotonin, and an increasing synthesis of prostaglandins in the damaged tissue surroundings. The later phase is sustained by prostaglandin release (Brito & Antonio, Citation1998). Dextran is a polysaccharide of high molecular weight that induces anaphylactic reaction when injected on the extremities of mice and rats. This is characterized by extravasation and edema formation due to mast cell degranulation (Ankier & Neat, Citation1972). Liberation of histamine and serotonin from mast cells after the sub-plantar injection of dextran is considered to mediate the rapid production of paw edema (Merlos et al., Citation1990). In the present study, MZ showed significant anti-inflammatory activity against both carrageenan- and dextran-induced paw edema of mice.
Formalin-induced paw edema is one of the most suitable test procedures to screen anti-inflammatory agents against chronic inflammation as it closely resembles human arthritis. The nociceptive effect of formalin is also biphasic; an early neurogenic component mediated by substance P and bradykininis followed by a later tissue mediated response where histamine, prostaglandins, and bradykinin are known to be involved (Wheeler & Cowan, Citation1991). The first phase of nociception starts immediately after formalin injection, extending for the following 5 min, and is believed to occur due to direct chemical stimulation of nociceptors, predominantly afferent C fibers and partly Aδ fibers. The second phase of this model takes place 15–30 min after formalin injection and is related to the release of several pro-inflammatory mediators. It has been demonstrated that the nociception produced in phase 2 of the formalin test is a result of chemical insult resulting in tissue damage. Tissue destruction produces mediators of inflammation such as histamine, bradykinines, prostaglandins, and serotonin. MZ showed significant anti-inflammatory activity against formalin-induced chronic paw edema with mice.
Prostaglandins derived from the metabolism of arachidonic acid in inflammatory cells can contribute to the development of cancer (Baron & Sandler, Citation2000). Prostaglandins induce the expression of certain inflammatory cytokines, which can in turn enhance the production of reactive oxygen intermediates (ROI) and reactive nitrogen intermediates (RNI). ROI and RNI are produced under the stimulus of pro-inflammatory cytokines in phagocytic and non-phagocytic cells through the activation of protein-kinases signaling. For example, TNF-α enhances the formation of ROI by neutrophils and other cells, while IL-1β, TNF-α, and interferon (IFN)-γ stimulate the expression of iNOS in inflammatory and epithelial cells. Expression of iNOS, an enzyme catalyzing NO production, was found to be up-regulated in chronic inflammatory diseases and various types of cancer. NO is an important regulatory molecule in both inflammatory response and cancer development. Under the circumstances of chronic inflammation, the continuous generation of NO may lead to DNA damage, disruption of DNA repair, and cancer-prone post-translational modification. NO also regulates angiogenesis, leukocyte adhesion and infiltration, and metastasis. COX-2 expression is induced by a wide range of stimuli including LPS, pro-inflammatory cytokines such as IL-1β and TNF-α and growth factors such as epidermal growth factor. The products of COX-2 enzyme are prostaglandins, which are the key mediators of inflammation (Steele et al., Citation2003). Expression of the COX-2 gene was found to be up-regulated in many cancer types including carcinomas of colon, breast, lung, pancreas, esophagus, and head and neck (Gupta & DuBois, Citation2001; Lin et al., Citation2002).
Macrophages are important in non-specific host resistance to microbial pathogens and they serve as central regulators of the specific immune response. Pro-inflammatory cytokines such as TNF-α, IL-1β, and IL-6 are among the various protein mediators produced by macrophages. In the present study, macrophage activation by LPS, the major component of Gram-negative bacterial cell wall, results in the massive release of several inflammatory mediators such as NO, C-reactive protein, and pro-inflammatory cytokines. But all these were significantly decreased by MZ treatment, signifying the anti-inflammatory potential of MZ.
Modulation of chronic inflammatory response at molecular level by anti-inflammatory agents represents an important strategy in chemoprevention and cytoprotection (Kundu & Surh, Citation2008). RT-PCR analysis revealed the up-regulated expression of inflammatory mediator genes like COX-2, iNOS, and TNF-α in LPS-alone-treated macrophages. These up-regulated genes were significantly down-regulated when the macrophages were cultured along with MZ and LPS, confirming anti-inflammatory activity of carotenoid MZ. The anti-inflammatory activity of MZ can be attributed to its efficacy in down-regulating these inflammatory genes. As chronic inflammation has been linked to various steps involved in carcinogenesis, the present study indicates the usefulness of MZ – a non-toxic carotenoid in the cancer prevention strategies.
Acknowledgements
We express our gratitude to Dr. Jayant Deshpande and Mr. Abhijit Bhattacharya for their interest in this work. A. P. Firdous expresses gratitude to ICMR for providing a Senior Research Fellowship to do the work.
Declaration of interest
The authors report that they have no declarations of interest. We are thankful to M/s. Omni Active Health Technologies Pvt. Ltd., for providing funds to carry out this work.
References
- Ankier SI, Neat ML. (1972). Some studies on acute inflammation induced by dextran in the mouse. Int Arch Allergy Appl Immunol. 42:264–77
- Baron JA, Sandler RS. (2000). Nonsteroidal anti-inflammatory drugs and cancer prevention. Annu Rev Med. 51:511–23
- Brito AR, Antonio MA. (1998). Oral anti-inflammatory and antiulcerogenic activities of a hydroalcoholic extract and partitioned fractions of Turnera ulmifolia (Turneraceae). J Ethnopharmcol. 61:215–28
- Chang GJ. (2006). Thirteen week oral (gavage) toxicity of meso-zeaxanthin in Hans Wistar rats with a four-week recovery. Cambridge, UK: Howard Foundation, Study no. 1567--–4370
- Chau TT. (1989). Analgesic testing in animals models. In: Chang JY, Lewis AJ, ed. Pharmacological Methods in the Control of Inflammation. New York: Alan R. Liss, Inc., 195–212
- Coussens LM, Werb Z. (2002). Inflammation and cancer. Nature. 420:860–7
- Firdous AP, Preethi KC, Kuttan R. (2010). Antioxidant potential of meso-zeaxanthin a semi-synthetic carotenoid. Food Chem. 119:1096–101
- Firdous AP, Sindhu ER, Ramnath V, Kuttan R. (2011). Anti-mutagenic and anti-carcinogenic potential of the carotenoid meso-zeaxanthin. Asian Pac J Cancer Prev. 11:1795–800
- Firdous AP, Kuttan R. (2012). Amelioration of cisplatin-induced toxicity in mice by carotenoid meso-zeaxanthin. Hum Exp Toxicol. 31:710–17
- Firdous AP, Sindhu ER, Ramnath V, Kuttan R. (2013a). Amelioration of radiation-induced damages in mice by carotenoid meso-zeaxanthin. Int J Radiat Biol. 89:171–81
- Firdous AP, Sindhu ER, Ramnath V, Kuttan R. (2013b). Anti-carcinogenic activity of meso-zeaxanthin in rodents and its possible mechanism of action. Nutr Cancer. 65:850–6
- Green LC, Wagner DA, Glogowski J, et al. (1982). Analysis of nitrate and nitrite and (15-N) nitrite in biological fluids. Anal Biochem. 126:131–8
- Grivennikov SI, Greten FR, Karin M. (2010). Immunity, inflammation and cancer. Cell. 140:883–99
- Gupta RA, DuBois RN. (2001). Colorectal cancer prevention and treatment by inhibition of cyclooxygenase-2. Nat Rev Cancer. 1:11–21
- Kundu JK, Surh YJ. (2008). Inflammation: Gearing the journey to cancer. Mutat Res. 659:15–30
- Licastro F, Candore G, Lio D, et al. (2005). Innate immunity and inflammation in ageing: A key for understanding age-related diseases. Immun Ageing. [Epub ahead of print]. doi:10. 1186/1742-4933-2-8
- Lin DT, Subbaramaiah K, Shah JP, et al. (2002). Cyclooxygenase-2: A novel molecular target for the prevention and treatment of head and neck cancer. Head Neck. 24:792–9
- Maity TK, Mandal SC, Mukherjee PK. (1988). Studies on anti-inflammatory effect of Cassia tora leaf extract (Fam. Leguminosae). Phytother Res. 12:221–4
- Merlos M, Gomez LA, Vericat L, et al. (1990). Comparative study of the effect of CV-6209, a specific PAF antagonist, on paw edema caused by different phlogogen agents. Pharmacology. 40:211–17
- Steele VE, Hawk ET, Viner JL, Lubert RA. (2003). Mechanisms and applications of non-steroidal anti-inflammatory drugs in the chemoprevention of cancer. Mutat Res. 523:137–44
- Wheeler AH, Cowan A. (1991). Neurogenic and tissue mediated components of formalin-induced edema: Evidence for supraspinal regulation. Agents Actions. 34:264–9
- Winter CA, Risly EA, Nuss CW. (1962). Carrageenan induced paw edema in hind paw of the rats – An assay for its anti-inflammatory drugs. Proc Soc Exp Biol Med. 11:544–7
- Yoshimura A. (2006). Signal transduction of inflammatory cytokines and tumor development. Cancer Sci. 97:439–47