Abstract
Context: Thunbergia laurifolia Lindl. (Acanthaceae) is a Thai medicinal plant used for the detoxification of poison which is likely to be beneficial for the treatment of cognitive deficits including Alzheimer's disease.
Objective: To elucidate the effects of Thunbergia laurifolia leaf extract (TLL) on cognitive dysfunction and depression-like behavior in olfactory bulbectomized mice (OBX).
Materials and methods: OBX mice were treated daily with TLL at the dose of 250 and 500 mg/kg, tacrine, and imipramine, on the day after 10 d of OBX operation. The effects of TLL on cognitive and depression-like behavior of the animals were analyzed. After completing behavioral experiments, the expression levels of cholinergic marker genes encoding ChAT and muscarinic M1 receptor were quantitatively analyzed.
Results: TLL and tacrine reduced OBX-induced cognitive deficits in the object recognition test (ORT) with the time spent for the novel object two times longer than that of the familiar object. Moreover, TLL at the dose of 500 mg/kg and imipramine ameliorated depression-like behavior in the tail suspension test (TST) by reducing the duration of immobility from 25.18% to 3.16% and from 25.18% to 6.48%, respectively. TLL at the dose of 250 and 500 mg/kg reversed the OBX-induced down-regulation of ChAT mRNA expression in the hippocampus from 0.12 to 0.17 and 0.24, respectively, while the down-regulation of mRNA expression of muscarinic M1 receptor was also reversed by TLL from 0.23 to 0.38 and 0.48, respectively.
Conclusions: TLL ameliorates non-spatial short-term memory deficits in OBX mice, and has the potential to exhibit an antidepressant-like action.
Introduction
Dementia is a problematic disease, with an explosive increase in the number of patients worldwide. Alzheimer's disease (AD), the most common form of dementia, is a neurodegenerative disease characterized by progressive memory loss and cognitive impairment (Wancata et al., Citation2003). AD is pathologically characterized by the extracellular deposition of senile plaques, formation of intracellular neurofibrillary tangles, and lesions of cholinergic neurons together with synaptic alterations in the cerebral cortex, hippocampus, and other brain regions (Tayeb et al., Citation2012). However, current approved treatment with anti-dementia drugs such as donepezil, an acetylcholinesterase inhibitor, and memantine, an N-methyl-d-aspartate receptors (NMDA) antagonist, has provided only marginal therapeutic benefits without affecting the progression of the disease (Shoji, Citation2010). Therefore, it is essential to explore novel and effective medications for dementia including AD.
Thunbergia laurifolia Lindl. (Acanthaceae) is a medicinal herb known as Rang Jurd (RJ) in Thailand. An aqueous extract from the leaves and root of this plant has been used in traditional Thai medicine as an anti-inflammatory and antipyretic agent as well as for the detoxification of poison including insecticide, ethyl alcohol, arsenic, and strychnine (Thongsaard & Marsden, Citation2002; Thongsaard et al., Citation2005). An extract of T. laurifolia dried leaves in boiling water contains chemicals with radical scavenging activity, such as apigenin and its glycosides, and phenolic acids such as caffeic acid, gallic acid, and protocatechuic acid (Oonsivilai et al., Citation2007). Moreover, a leaf extract of T. laurifolia reportedly alleviated the adverse effects of lead on spatial cognitive performance in mice (Tangpong & Satarug, Citation2010). These features have been attracting considerable interest regarding the potential usefulness of this plant for the treatment of various diseases including cognitive deficits.
Studies have indicated that impairment of the olfactory system leads to memory and cognitive disturbance in rodents. For example, impaired performance in learning and memory tasks such as passive avoidance, the modified Y-maze, and object recognition has been observed in olfactory bulbectomized (OBX) mice and rats (Borre et al., Citation2012; Shioda et al., Citation2010; Sithisarn et al., Citation2013; Yamada et al., Citation2011). In addition, an elevated level of β-amyloid peptide (Aleksandrova et al., Citation2004) and degeneration of cholinergic neurons (Hozumi et al., Citation2003) in the brain are also caused by OBX. Interestingly, the impairment of olfactory perceptual acuity is observed not only in the early stages of AD (Makowska et al., Citation2011; Serby, Citation1987) but also in a transgenic mouse model of AD (Wesson et al., Citation2010), suggesting that OBX animals provides a beneficial model of AD that is independent from transgenic animal models. Therefore, in this study, we investigated the effects of an aqueous T. laurifolia leaf extract (TLL) on cognitive and emotional deficits observed in an animal model of OBX to clarify the possible usefulness of TLL for AD prevention/therapy.
Materials and methods
Plant material
Leaves of Thunbergia laurifolia were collected from the Wangnamyen district, Sa Kaeo Province, Thailand, in May 2011. The plant samples were identified by Mr. Pinit Chinsoi, a Pharmacist of Wangnamyen Hospital, Sa Kaeo province, Thailand. Voucher specimens (TLL01001) were deposited in the Department of Pharmaceutical Chemistry, Faculty of Pharmacy, Mahidol University, Thailand.
Extract preparation
The leaves were cleaned, dried in a hot air oven (60 °C) for 6 h, and powdered with an electronic mill (20 mesh sieve). The powdered leaves (200 g) were boiled with 2 L of distilled water three times, each for 8 h, and then filtered through Whatman Filter paper no. 1. The combined filtrate was dried by lyophilization to give a dried leaf decoction extract (TLL) at a yield of 20.80%w/w.
Chemical analysis of the extract by LC-MS
LC-MS analyses were performed with a Shimadzu LC-IT-TOF mass spectrometer (Shimadzu, Canby, OR) equipped with an ESI interface. A Waters Atlantis T3 column (Waters Corporation, Milford, MA) (2.1 mm i.d. × 100 mm) was used with a column temperature of 40 °C. The mobile phase consisted of (A) 5 mM ammonium acetate solution and (B) CH3CN with the following gradient conditions: 0–30 min linear gradient from 10 to 100% B, 30–40 min isocratic gradient at 100% B. The flow rate was 0.15 mL/min. The ESI parameters were as follows: source voltage −3.5 kV, capillary temperature 200 °C, and nebulizer gas 1.5 L/min. The mass spectrometer was operated in positive and negative ion scanning modes from m/z 200 to 2000.
Experimental animals
The study was conducted according to the experimental protocols described in . Male ddY mice (Japan SLC Inc., Shizuoka, Japan) were obtained at the age of 9 weeks old and housed under a constant humidity (65%) and 12-h light/dark cycle (lights on: 07:00–19:00) at 22 ± 1 °C. Food and water were given ad libitum. The animals were habituated to the laboratory animal room for at least 1 week before surgery. The behavioral experiments were performed during the light phase from 9:00 to 18:00. The study was conducted in accordance with the Guiding Principles (NIH publication #85-23, revised in 1985) for the Care and Use of Animals and approved by the Institutional Animal Use and Care Committee of the University of Toyama.
Figure 1. Schematic drawing of the experimental schedule. Protocol 1: after 1 week of acclimatization, the ddY mice were randomly divided into four groups of 10 mice. All mice (except mice in the sham group) were subjected to OBX surgery. Ten days after the surgery, drug administration was started. Modified Y-maze and object recognition tests were performed 1 and 3 weeks after starting drug administration, respectively. Quantitative real-time polymerase chain reaction and neurochemical studies were carried out after the decapitation of all mice. Protocol 2: after 1 week of acclimatization, the mice were randomly divided into four groups of six mice. All mice (except mice in the sham group) were subjected to OBX surgery. Three days after the surgery, drug administration was started. The tail suspension test was performed 7 d after starting drug administration.
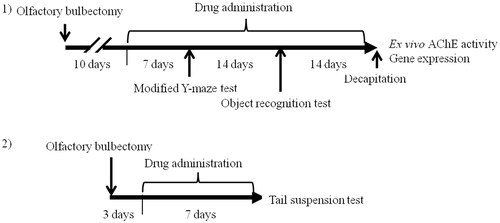
Surgical operation
Mice were anesthetized with sodium pentobarbital (60 mg/kg, i.p.) and fixed on stereotactic instruments (Narishige, Tokyo, Japan). The skull covering the bulbs was exposed by skin incision with 1% lidocaine solution as local anesthetic and then a 1-mm burr hole was drilled. The bilateral bulbs were aspirated through a syringe and the cavity of the bulbs was filled with hemostatic gelatin sponge. The animals were sacrificed after completing behavioral studies, and the surgical lesion was verified visually. The data from animals with less than 70% removal or without an intact cortex were excluded from the analysis. Sham operation was conducted in a similar way without the removal of the bulb. At the end of experiments, the olfactory bulbs of the sham group mice were confirmed to be intact.
Drug administration
Vehicle water or test drugs were administered daily from 10 d after the surgical operation unless otherwise stated (Protocol 2, ). The sham group of mice and the OBX control group mice received water by oral gavage daily at the dose of 10 mL/kg. Reference standard drugs, tacrine HCl (2.5 mg/kg) and imipramine HCl (10 mg/kg) (Nacalai Tesque, Kyoto, Japan), were dissolved in 0.9% normal saline solution and administered intraperitoneally. TLL was dissolved in water and given per os daily by oral gavage at the doses of 250 and 500 mg/kg, being almost equivalent to the daily doses used for human therapy. TLL at this dose range caused no toxic responses in the animals during a 14-d administration period. On a behavioral experiment day, the administration of test drugs was conducted 1 h before testing.
Locomotor activity
One hour after the last administration, the locomotor activity of each animal in the open field (35 × 35 × 50 cm) was video-recorded. The total distance that an animal moved in the area during a 10-min observation period was measured using the SMART® system ver. 2.5 (PanLab, S.L., Barcelona, Spain) as an index of locomotor activity.
Modified Y-maze test
The apparatus used for the modified Y-maze test consists of black polypropylene walls with three arms, each being 40 cm long, 12 cm wide at the top, 3 cm wide at the bottom, and 18 cm high. This test is a two-trial task with a sample phase trial and a test phase trial that are separated by a 30-min inter-trial interval. In the sample phase trial, each mouse was individually placed in the maze with one of the three arms closed. The animals were allowed to freely explore the other two arms for 5 min. Thirty minutes after the sample phase trial, the animal was again placed in the maze with all three arms opened, and allowed to explore the arms freely. The closed arm that was opened in the test phase trial was defined as the new arm. Animal behavior was video-recorded for later analysis. The percent time spent in the new arm and total numbers of arm entries were analyzed with the SMART® system ver. 2.5 (PanLab, S.L., Barcelona, Spain).
Novel object recognition test
The test was conducted as previously described (Zhao et al., Citation2011). The animals were individually habituated to an observation chamber (35 × 35 × 50 cm) for 10 min on the day before the experiment. The object recognition test (ORT) consists of a sample phase trial and a test phase trial. In the sample phase trial, each mouse was placed in the chamber where two identical objects, objects O1 and O2, were placed separately, and allowed to explore the arena freely for 5 min. Thirty minutes after the sample phase trials, one of the objects was replaced by a novel object, and exploratory behavior was again analyzed for 5 min. After each session, the objects were thoroughly cleaned with 70% ethanol to prevent odor recognition. Exploration of an object was defined as rearing on the object or sniffing it at a distance of less than 2 cm. Successful recognition of a previously explored object was reflected by preferential exploration of the novel object. Discrimination of spatial novelty was assessed by comparing the difference between the times of exploration of the novel and familiar objects. The time spent exploring each of the two objects was analyzed using SMART® system ver. 2.5 (PanLab, S.L., Barcelona, Spain) with a tri-wise module to detect the head, center mass, and base-tail (PanLab, S.L., Barcelona, Spain).
Tail suspension test (TST)
The animals were subjected to the short-term inescapable stress of being suspended by the tail, leading to the development of an immobile posture. Using another group of mice as indicated in protocol 2 (), three mice were separately suspended 50 cm above the floor in a chamber by adhesive tape placed approximately 2 cm from the tip of the tail. The animal behavior in the test was video-recorded for later analysis. Immobility was defined as a state with movement speed of not more than 0.05 cm2/s using the SMART® system ver. 2.5 (PanLab, S.L., Barcelona, Spain), and the immobility time was recorded during an 8-min period.
Ex vivo measurement of cholinesterase activity in the brain
After completing the behavioral experiments, mice were decapitated and the frontal cortices were dissected out and kept at −80 °C until use. Determination of cholinesterase activity was performed on the basis of the colorimetric method by slightly modifying previous methods (Ellman et al., Citation1961; Mizuki et al., Citation2014; Zhao et al., Citation2007). Briefly, the frozen cortex was weighed and homogenized in 10 volumes of 0.1 M phosphate buffer (pH 7.4) containing 1% Triton X-100. After centrifugation at 15 000 × g at 4 °C for 20 min, the clear supernatants were collected and served as the enzyme source. Cholinesterase activity was determined in 10-μL aliquots of the homogenates (run as triplicates) in a 96-well flat-bottomed microplate. The reaction was started by adding 8 μL of 10 mM 5,5′-dithiobis-2-nitrobenzoic acid (DTNB), 16 μL of 7.5 mM acetylthiocholine (ATCI), and 201 μL of 0.1 M sodium phosphate buffer (pH 8.0). The spectrophotometric absorption at 405 nm during a 3-min incubation period at 25 °C was quantitatively measured using a microplate reader (Sunrise Classic; TECAN Japan, Kawasaki), and the activity is expressed as nmol of acetylthiocholine hydrolyzed/min/mg tissue.
Quantitative real-time polymerase chain reaction (PCR)
The animals were decapitated after completing the behavioral studies to analyze changes in the expression levels of choline acetyltransferase (ChAT) and muscarinic M1 receptor mRNAs in the brain. The hippocampi were immediately dissected out and kept at −80 °C until use. The total RNA was extracted from the tissue using Sepazol® (Nacalai Tesque, Kyoto, Japan) according to the manufacturer’s instructions. First-strand cDNA was synthesized using oligo (dT) primers and M-MLV Reverse Transcriptase® (Invitrogen, Rockville, MD) in a total volume of 20 μL. DNA corresponding to the RNA was used as a template for PCR. Quantitative real-time PCR was carried out using Fast SYBR Green Master Mix (Applied BioSystems, Foster City, CA) in a StepOne Real-time PCR System (Applied BioSystems, Foster City, CA). The following primer sets were obtained from Nippon EGT Co. (Toyama, Japan): ChAT mRNA (NM-009891): 5′-CCTGTACAAGCTTCTAGCTGTGAG-3′ (forward) and 5′-GTAGCTAAGCACACCAGAGATGAG-3′ (reverse); muscarinic M1 receptor mRNA (M16406): 5′-ACTGTCTTGGCACCAGGAAA-3′ (forward) and 5′-TGCTAGGCCAATCATCAGAG-3′ (reverse); and β-actin mRNA (NM-007393): 5′-CATCCGTAAAGACCTCTATGCCAAC-3′ (forward) and 5′-ATGGAGCCACCGATCCACA-3′ (reverse). Melting curve analysis of each gene was performed each time after amplification. Standard curves of the log concentration of each gene versus cycle threshold were plotted to verify negative linear correlations.
Data analysis
The results are expressed as the mean ± SEM. The data obtained from the behavioral tests and neurochemical experiments were analyzed by paired and unpaired Student’s t-tests or one-way analysis of variance (ANOVA) followed by a post-hoc multiple comparison test (Dunnett’s method) as appropriate. Differences of p < 0.05 were considered significant. The analysis was conducted using SigmaStat® ver. 3.5 (SYSTAT Software Inc., Richmond, CA).
Results
Chemical analysis of the extract by LC-MS
Mass spectrometry data obtained from the aqueous extract of TLL have been listed in MassBank database and stored in the Wakan-Yaku DataBase system (http://wakandb.u-toyama.ac.jp/wiki/LCMS:Thunbergia_INM-532), Institute of Natural Medicine, University of Toyama, Japan. Total ion chromatograms in LC-MS (TOF) of the aqueous extract of TLL showed peaks which corresponded to schaftoside and vitexin at retention times of 10.41 and 11.60 min, respectively ().
The effect of TLL and tacrine on OBX-induced elevation of motor activity
As summarized in , no significant difference in locomotor activity was found between vehicle-treated sham and OBX groups. Moreover, the daily administration of tacrine (2.5 mg/kg, i.p.) or TLL (250 and 500 mg/kg, p.o.) for 1 week before the experiments had no effect on the activity.
Table 1. Effect of TLL and tacrine on locomotor activity of OBX mice.
Effects of TLL and tacrine on OBX-induced spatial working memory deficits in the modified Y-maze test
As shown in , the percentage time spent by the vehicle-treated sham group exploring the new arm was significantly greater than the chance level of 33.3%, indicating a preference for the new arm over the familiar arms. In contrast, the vehicle-treated OBX mice spent a significantly shorter time exploring the new arm than the vehicle-treated sham group, indicating that OBX caused impairment of spatial working memory in mice. The tacrine-treated OBX mice (2.5 mg/kg, i.p.) spent significantly longer time visiting the new arm than the vehicle-treated OBX group, while TLL-treated groups (250 and 500 mg/kg. p.o.) spent a non-significantly longer time visiting the new arm than the vehicle-treated OBX group.
Figure 3. Evaluation of the modified Y-maze test of OBX-induced spatial working memory deficit mice using tacrine or T. laurifolia leaf extract (TLL). Sham-operated mice were orally administered distilled water 60 min before the sample phase trial, while tacrine (2.5 mg/kg) and TLL (250 and 500 mg/kg) were administered daily by intra-peritoneal injection and oral administration, respectively (n = 10). Each column represents the mean ± S.E.M. *p < 0.05 compared with the vehicle-treated OBX group (one-way ANOVA, Dunnett’s method).

TLL and tacrine improve OBX-induced non-spatial memory deficits in the novel ORT
In the sample phase trial in which two identical objects were used, none of the animal groups exhibited significant differences in the time spent exploring each object. No significant difference was found between the sham and OBX groups in the total time spent exploring the two objects (). In the test phase trial, the sham group spent a significantly longer time exploring the new object than the familiar one (p < 0.05), while the vehicle-treated OBX group showed a deficit in recognition performance for the novel object (). However, in the tacrine (2.5 mg/kg per day, i.p.)-treated and TLL (250 and 500 mg/kg per day, p.o.)-treated OBX groups, the time spent for the novel object was significantly longer than for the familiar object (p < 0.01).
Figure 4. Effects of tacrine (2.5 mg/kg per day, i.p.) and TLL (250–500 mg/kg per day, p.o.) on object recognition deficits in OBX mice in the sample and test phases. The time each mouse spent exploring objects in the sample (a) and test (b) phases was recorded using the SMART system (PanLab, S.L., Barcelona, Spain). Each value represents the mean ± S.E.M. (n = 10). Each column represents the mean ± S.E.M. *p < 0.05 and **p < 0.01 compared with the time spent exploring a familiar object (paired t-test).
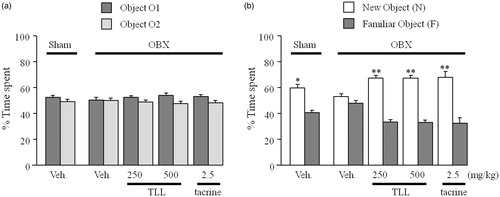
TLL improves OBX-induced depression-like behavior in the TST
The TST was conducted 10 d after OBX according to protocol 2. The duration of immobility measured as an index of depression-like behavior was significantly longer in the vehicle-treated OBX mice than in the sham-operated group. In this OBX model, the daily administration of imipramine (10 mg/kg per day, i.p.) and TLL (500 mg/kg per day, p.o.) significantly reduced the duration of immobility ().
Figure 5. Effects of TLL (500 mg/kg per day, p.o.) and imipramine (10 mg/kg per day, i.p.) on OBX-induced depressive behavior in the tail suspension test. The experiment was conducted according to protocol 2. Each column represents the mean ± S.E.M. *p < 0.05 versus % resting time in vehicle-treated OBX mice (one-way ANOVA, Dunnett’s method).
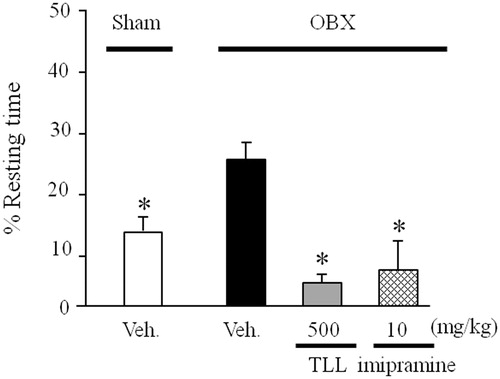
Effects of TLL on expression levels of cholinergic marker genes in the hippocampi of OBX mice
As shown in , the vehicle-treated OBX mice had significantly reduced levels of ChAT and muscarinic M1 mRNAs in the hippocampus compared with the vehicle-treated sham group. However, down-regulated expression levels of ChAT and M1 mRNAs were significantly reversed in the OBX groups treated with tacrine (2.5 mg/kg, i.p.) and TLL (250 and 500 mg/kg, p.o.).
Figure 6. Effects of tacrine (2.5 mg/kg per day, i.p.) and TLL (250–500 mg/kg per day, p.o.) on the expression levels of choline acetyltransferase (a) and muscarinic M1 receptor mRNAs (b) in the hippocampus of OBX mice. Each column represents the mean ± S.E.M. *p < 0.05 versus mRNA expression levels in vehicle-treated OBX mice (one-way ANOVA, Dunnett’s method).
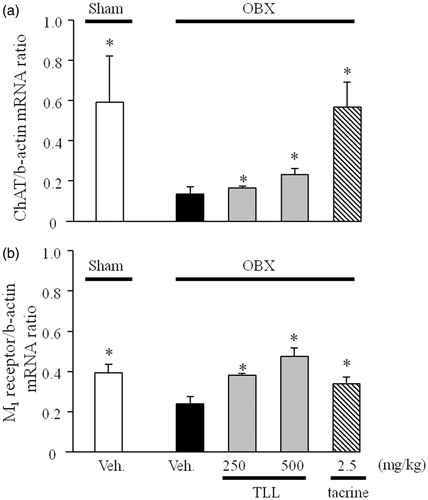
Effects of TLL on ex vivo activity of cholinesterase
We examined if the administration of TLL led to inhibitory activity against cholinesterase similarly to tacrine using the cortical brain homogenate as an enzyme source. The present ex vivo analysis revealed that the activity of cholinesterase in the brain homogenate prepared from tacrine-treated groups was significantly lower than the activity measured in the brain homogenate prepared from vehicle-treated OBX mice. However, the administration of TLL at a daily dose of 500 mg/kg (p.o.) had no effect on the ex vivo activity of cholinesterase in the brain homogenate ().
Discussion
In this study, to clarify if a leaf extract of the Thai medicinal plant Thunbergia laurifolia, namely TLL, can be used for dementia therapy or prevention, we investigated the effects of TLL on impaired cognitive function and emotional behavior in a mouse model of OBX. Our findings revealed that TLL treatment could ameliorate the short-term memory deficit and depression-like behavior in this model, and suggested that the effect on the cognitive deficits is attributable to the restoration of the central cholinergic function down-regulated by OBX.
First, we showed that OBX treatment slightly but non-significantly increased locomotor activity compared with the sham operation, and that the activity was not affected by TLL or tacrine administration. The failure of OBX to elevate ambulatory activity in mice conflicts with the findings reported by Harkin et al. (Citation1999) who demonstrated that OBX induces hyperactivity in rats in a manner reversed by antidepressant, leading to the concept that OBX-induced hyperactivity can be used as an index to screen for the antidepressant-like activity of test drugs (Kelly et al., Citation1997; Wang et al., Citation2007). The reasons for this conflict between the present and previous results are unclear; however, since our previous study also showed a non-significant increase in locomotor activity caused by OBX, it may be due to differences in experimental protocols to measure motor activity and/or the animal species used in the experiments. Nevertheless, the fact that spontaneous motor activity was not affected by TLL in a mouse model of OBX indicates that TLL, at the dose range tested in this study, induces no sedative effect in this model.
Employing a modified version of the Y-maze test and a novel ORT (Han et al., Citation2008; Sithisarn et al., Citation2013; Yamada et al., Citation2011; Zhao et al., Citation2007), we subsequently elucidated the effects of TLL on short-term spatial and non-spatial working memory performance of OBX animals, respectively. In agreement with our previous reports (Mizuki et al., Citation2014; Sithisarn et al., Citation2013; Yamada et al., Citation2011), OBX clearly impaired spatial and non-spatial working memory performance in the modified Y-maze test and novel ORT in a manner reversible by the daily administration of tacrine, supporting the concept that facilitation of the central cholinergic function is beneficial for improving hippocampus-dependent spatial memory deficits and hippocampus-independent non-spatial memory deficits of OBX animals. Moreover, the present study demonstrated that although the daily administration of TLL tended to improve OBX-induced spatial working memory deficits in the modified Y-maze test, the same treatment as well as tacrine administration significantly ameliorated OBX-induced non-spatial working memory deficits identified in the novel ORT. These findings allow us to consider that TLL is useful for the treatment of cognitive deficits related to dementia.
In the present study, the extent to which TLL induced the amelioration of cognitive deficits in OBX mice differed depending on the tasks used. The reasons for this difference are unclear. However, in our previous study using the same animal model, experimental protocols, and administration period, we found that a medicinal plant extract from Acanthopanax trifoliatus could improve the spatial and non-spatial cognitive performance of OBX animals (Sithisarn et al., Citation2013). Taken together, the present results allow us to speculate that the TLL administration period may be important in ameliorating cognitive dysfunction and that it needs to be more than 1 week to improve cognitive performance.
Evidence indicates that OBX causes the degeneration of septal cholinergic neurons projecting to the hippocampus and reduces the expression level of some cholinergic marker genes such as ChAT and muscarinic M1 receptor mRNA (Hozumi et al., Citation2003; Sithisarn et al., Citation2013; Yamada et al., Citation2011) and that OBX-induced cognitive deficits and septal cholingeric degeneration take at least 1 week after the OBX operation to become evident (Hozumi et al., Citation2003; Sithisarn et al., Citation2013). On the basis of these findings, we then elucidated the possible involvement of central cholinergic systems in its mechanisms of action by analyzing the expression levels of central cholinergic marker genes. The results indicated that the daily administration of TLL, which was started 10 d after OBX, as well as of tacrine, ameliorated the expression levels of these marker genes in OBX mice. Recently, Inada et al. (Citation2013) demonstrated that the elevation of the endogenous acetylcholine level rescues N-methyl-d-aspartate-induced long-lasting hippocampal cell damage via the stimulation of muscarinic M1 receptors. Moreover, Kakinuma et al. (Citation2005) demonstrated using cardiomyocytes and an ischemic hindlimb model that endogenous acetylcholine enhances the expression of hypoxia-inducible factor 1α-vascular and endothelial growth factor (VEGF) via stimulating muscarinic M1 receptors, and thereby, elevates ChAT expression. Therefore, a plausible explanation for the underlying mechanisms of the action of TLL is that it may act like tacrine and enhance central cholinergic neurotransmission. However, this possibility seems unlikely since the present ex vivo analysis showed that, in contrast to tacrine, TLL treatment failed to alter the activity of cholinesterase in the brain. Therefore, the present findings lead us to suggest that the TLL-induced amelioration of cognitive dysfunction and cholinergic systems in OBX animals is mediated by a mechanism different from that of tacrine.
This study further elucidated whether TLL administration affects depression-like behavior, an emotional deficit that can be distinctively elicited in OBX animals (Cryan & Mombereau, Citation2004; Harkin et al., Citation1999). Indeed, a depression model of OBX has been validated in rats and mice by its behavioral, neuro-endocrinological, and neurochemical similarities to human depression (Song & Leonard, Citation2005). For this aim, imipramine was used as a standard reference drug and a TST was used as a task to elucidate depression-like behavior induced by escapable stress stimuli. Consistent with previous reports (Shioda et al., Citation2010; Sithisarn et al., Citation2013), OBX mice showed significantly prolonged immobility, which was reflected by an increase in the percent resting time during the observation period, compared with that of sham-operated animals. Interestingly, TLL- and imipramine-treated but not tacrine-treated OBX mice exhibited a significantly reduced level of immobility compared with the vehicle-treated OBX group. The exact mechanism involved in the action of TLL needs to be clarified; however, considering the failure of tacrine treatment to affect the immobility of OBX animals, the present results raise the possibility that TLL administration is also beneficial for the improvement of depression-like symptoms.
From previous reports and our chemical analysis of TLL by LC-MS, it was found that T. laurifolia composed of various phytochemicals including some flavonoids such as apigenin, apigenin glycosides including schaftoside and vitexin, and phenolic compounds such as caffeic acid, gallic acid, and protocatechuic acid. The results of antidepressant and the ameliorative effects to cognitive and emotional deficits in OBX mice from this experiment corresponded to the previous researches which indicated that some constituents in T. laurifolia promoted related biological activities. Apigenin was reported as an active compound in various plants that promoted anxiolytic effects in animal models (Viola et al., Citation1995; Zanoli et al., Citation2000). This compound also exhibited the neuroprotection against Aβ-induced toxicity in the presence of copper in an AD cell model (Zhao et al., Citation2013). Apigenin and caffeic acid were also reported as possible active components in plants with antidepressant effects (Takeda, Citation2002; Nakazawa et al., Citation2003). Schaftoside was reported as a major compound in sugarcane juice which exerted free radical-scavenging activity against DPPH radicals (Asikin et al., Citation2013). To standardize TLL extract, the total phenolic content and HPLC analysis of vitexin and caffeic acid in TLL extract was also conducted. Total phenolic content of the extract was 3.57 ± 0.42 g gallic acid equivalent (GAE) per 100 g dried extract tested by the Folin–Ciocalteu method (Sanguansataya et al., Citation2013). Vitexin and caffeic acid contents were 0.10 ± 0.00 and 0.12 ± 0.08% w/w in dried extract, respectively (Sanguansataya, Citation2013). Thin-layer chromatography was also performed to demonstrated TLC fingerprint of the TLL extract (Sanguansataya et al., Citation2013).
Conclusions
TLL showed improving effects on non-spatial short-term memory in OBX mice, which could be mediated by the normalization of central cholinergic systems. Moreover, TLL also has the potential to exhibit an antidepressant-like action. Therefore, TLL administration is likely to be beneficial for the treatment of dementia patients such as those with AD with depression-like symptoms.
Declaration of interest
The authors declare that they have no conflicts of interest to disclose. This work was in part supported by a Grant-in-Aid for the 2011 Cooperative Research Project II from the Institute of Natural Medicine, University of Toyama, Japan, to Drs. Rojsanga P. and Kinzo Matsumoto.
References
- Aleksandrova IY, Kuvichkin VV, Kashparov IA, et al. (2004). Increased level of beta-amyloid in the brain of bulbectomized mice. Biochemistry (Moscow) 69:176–80
- Asikin Y, Takahashi M, Mishima T, et al. (2013). Antioxidant activity of sugarcane molasses against 2,20-azobis(2-amidinopropane) dihydrochloride-induced peroxyl radicals. Food Chem 141:466–72
- Borre Y, Bosman E, Lemstra S, et al. (2012). Memantine partly rescues behavioral and cognitive deficits in an animal model of neurodegeneration. Neuropharmacology 62:2010–7
- Cryan JF, Mombereau C. (2004). In search of a depressed mouse: Utility of models for studying depression-related behavior in genetically modified mice. Mol Psychiatr 9:326–57
- Ellman GL, Courtney KD, Andres V Jr, Feather-Stone RM. (1961). A new and rapid colorimetric determination of acetylcholinesterase activity. Biochem Pharmacol 7:88–95
- Han F, Shioda N, Moriguchi S, et al. (2008). Spiro[imidazo[1,2-a]pyridine-3,2-indan]-2(3H)-one (ZSET1446/ST101) treatment rescues olfactory bulbectomy-induced memory impairment by activating Ca2+/calmodulin kinase II and protein kinase C in mouse hippocampus. J Pharmacol Exp Ther 326:127–34
- Harkin A, Kelly JP, McNamara M, et al. (1999). Activity and onset of action of reboxetine and effect of combination with sertraline in an animal model of depression. Eur J Pharmacol 364:123–32
- Hozumi S, Nakagawasai O, Tan-No K, et al. (2003). Characteristics of changes in cholinergic function and impairment of learning and memory-related behavior induced by olfactory bulbectomy. Behav Brain Res 138:9–15
- Inada C, Thi Le X, Tsuneyama K, et al. (2013). Endogenous acetylcholine rescues NMDA-induced long-lasting hippocampal cell damage via stimulation of muscarinic M(1) receptors: Elucidation using organic hippocampal slice cultures. Eur J Pharmacol 699:150–9
- Kakinuma Y, Ando M, Kuwabara M, et al. (2005). Acetylcholine from vagal stimulation protects cardiomyocytes against ischemia and hypoxia involving additive non-hypoxic induction of HIF-1alpha. FEBS Lett 579:2111–18
- Kelly JP, Wrynn AS, Leonard BE. (1997). The olfactory bulbectomized rat as a model of depression: An update. Pharmacol Ther 74:299–316
- Makowska I, Kloszewska I, Grabowska A, et al. (2011). Olfactory deficits in normal aging and Alzheimer's disease in the Polish elderly population. Arch Clin Neuropsychol 26:270–9
- Mizuki D, Qi Z, Tanaka K, et al. (2014). Butea superba induced amelioration of cognitive and emotional deficits in olfactory bulbectomized mice and putative mechanisms underlying its actions. J Pharmacol Sci 124:457–67
- Nakazawa T, Yasuda T, Ueda J, Ohsawa K. (2003). Antidepressant-like effects of apigenin and 2,4,5-trimethoxycinnamic acid from Perilla frutescens in the forced swimming test. Biol Pharm Bull 26:474–80
- Oonsivilai R, Cheng C, Bomser J, et al. (2007). Phytochemical profiling and phase II enzyme-inducing properties of Thunbergia laurifolia Lindl. (RC) extracts. J Ethnopharmacol 114:300–6
- Sanguansataya T. (2013). Chemical standardization of Thunbergia laurifolia leaf extract (Master's thesis). Bangkok, Thailand: Faculty of Pharmacy, Mahidol University
- Sanguansataya T, Suntornsuk L, Sithisarn P, Rojsanga P. (2013). Antioxidants and total phenolic contents of Thunbergia laurifolia leaf extract collected at different locations. Proceedings of the Eighth Indochina Conference on Pharmaceutics Science; Ho Chi Minh City, Vietnam, 899–903
- Serby M. (1987). Olfactory deficits in Alzheimer's disease. J Neural Transm Suppl 24:69–77
- Shioda N, Yamamoto Y, Han F, et al. (2010). A novel cognitive enhancer, ZSET1446/ST101, promotes hippocampal neurogenesis and ameliorates depressive behavior in olfactory bulbectomized mice. J Pharmacol Exp Ther 333:43–50
- Shoji M. (2010). Drug therapy for Alzheimer's disease. Brain Nerve 62:777–85
- Sithisarn P, Rojsanga P, Jarikasem S, et al. (2013). Ameliorative effects of Acanthopanax trifoliatus on cognitive and emotional deficits in olfactory bulbectomized mice: An animal model of depression and cognitive deficits. Evid Based Complement Alternat Med 2013:701956
- Song C, Leonard BE. (2005). The olfactory bulbectomised rat as a model of depression. Neurosci Biobehav Rev 29:627–47
- Takeda H, Tsuji M, Inazu M, et al. (2002). Rosmarinic acid and caffeic acid produce antidepressive like effect in the forced swimming test in mice. Eur J Pharmacol 449:261–7
- Tangpong J, Satarug S. (2010). Alleviation of lead poisoning in the brain with aqueous leaf extract of the Thunbergia laurifolia (Linn.). Toxicol Lett 198:83–8
- Tayeb HO, Yang HD, Price BH, Tarazi FI. (2012). Pharmacotherapies for Alzheimer's disease: Beyond cholinesterase inhibitors. Pharmacol Ther 134:8–25
- Thongsaard W, Marsden CA. (2002). A herbal medicine used in the treatment of addiction mimics the action of amphetamine on in vitro rat striatal dopamine release. Neurosci Lett 329:129–32
- Thongsaard W, Marsden CA, Morris P, et al. (2005). Effect of Thunbergia laurifolia, a Thai natural product used to treat drug addiction, on cerebral activity detected by functional magnetic resonance imaging in the rat. Psychopharmacology (Berl) 180:752–60
- Viola H, Wasowski C, Levi de Stein M, et al. (1995). Apigenin, a component of Matricaria recutita flowers, is a central benzodiazepine receptors-ligand with anxiolytic effects. Planta Med 61:213–6
- Wancata J, Musalek M, Alexandrowicz R, Krautgartner M. (2003). Number of dementia sufferers in Europe between the years 2000 and 2050. Eur Psychiatr 18:306–13
- Wang D, Noda Y, Tsunekawa H, et al. (2007). Behavioural and neurochemical features of olfactory bulbectomized rats resembling depression with comorbid anxiety. Behav Brain Res 178:262–73
- Wesson DW, Levy E, Nixon RA, Wilson DA. (2010). Olfactory dysfunction correlates with amyloid-beta burden in an Alzheimer's disease mouse model. J Neurosci 30:505–14
- Yamada M, Hayashida M, Zhao Q, et al. (2011). Ameliorative effects of yokukansan on learning and memory deficits in olfactory bulbectomized mice. J Ethnopharmacol 135:737–46
- Zanoli P, Avallone R, Baraldi M. (2000). Behavioral characterisation of the flavonoids apigenin and chrysin. Fitoterapia 71:S117–23
- Zhao L, Wang JL, Wang YR, Fa XZ. (2013). Apigenin attenuates copper-mediated β-amyloid neurotoxicity through antioxidation, mitochondrion protection and MAPK signal in activation in an AD cell model. Brain Res 1492:33–45
- Zhao Q, Matsumoto K, Tsuneyama K, et al. (2011). Diabetes-induced central cholinergic neuronal loss and cognitive deficit are attenuated by tacrine and a Chinese herbal prescription, kangen-karyu: Elucidation in type 2 diabetes db/db mice. J Pharmacol Sci 117:230–42
- Zhao Q, Murakami Y, Tohda M, et al. (2007). Chotosan, a kampo formula, ameliorates chronic cerebral hypoperfusion-induced deficits in object recognition behaviors and central cholinergic systems in mice. J Pharmacol Sci 103:360–73