Abstract
Context: Ostrich (Struthio camelus) egg possesses a high amount of food proteins and thus plays an important role in nutrition.
Objective: Ostrich egg white proteins were hydrolyzed with pepsin and pancreatin to examine its antioxidant properties and further characterized the most active peptide.
Materials and methods: Ostrich egg white protein hydrolysate (OEWPH) was fractionized using reversed phase high-pressure liquid chromatography (HPLC). The antioxidant activity of OEWPH and its HPLC fraction were investigated based on their scavenging capacity1,1-diphenyl-2-picrylhydrazyl (DPPH) radical, 2,2′-azinobis (3-ethylbenzothiazoline-6-sulphonic acid) diammonium salt (ABTS), superoxide (), hydroxyl (OH•−) radicals, and Cu+2 chelating. In a wound healing assay, paravertebral excision (1 cm diameter) was made on the skin and the percentage of wound closure was measured at defined intervals (0, 3, 7, and 14 d).
Results: A potent antioxidant peptide named DG-10 with the sequence DAESLSRLLG (MW: 1060.18 ± 0.5 Da) was identified from OEWPH. The peptide DG-10 showed DPPH (IC50 = 0.0085 mg/ml), ABTS•+ (IC50 = 0.56 mg/ml), superoxide (IC50 = 0.36 mg/ml), and hydroxyl (IC50 = 0.4 mg/ml) radical scavenger and copper chelating activity (IC50 = 0.28 mg/ml). In vitro cultured HFLF-pI 5, the cell model, also revealed that DG-10 could protect HFLF-pI 5 cells against H2O2-treated necrosis. Ointment composed of DG-10 peptide exhibited wound-healing properties on adult rats (Wistar strain). The percentage of wound closure in peptide-treated group was 98% by day 14.
Discussion and conclusion: Our results suggested that DG-10 is a natural agent obtained from ostrich egg possessing considerable antioxidant and wound-healing properties.
Introduction
Oxidative stress is lack of equilibrium between the production of free radicals and reactive metabolites. Oxidant compounds or reactive oxygen species (ROS) must be eliminated by protective mechanisms since ROS can react with different cellular components (Bedard & Krause, Citation2007). Enhanced oxidative stress demonstrates a pathogenic mechanism that can lead to tissue damage and cell death (Bedard & Krause, Citation2007; Leung & Chan, Citation2009). It has been shown that oxidative stress is brought about by the occurrence of chronic diseases, including diabetes mellitus, osteoporosis, cancer, atherosclerosis, coronary heart disease, neurological malfunctioning, and weakening of the immune system (Halliwell, Citation1994; Harman, Citation2001; Kim & Wijesekara, Citation2010; Shahidi & Zhong, Citation2010).
Nowadays, many synthetic antioxidants like butylated hydroxyl anisole (BHA), butylated hydroxyl toluene (BHT), proppylgallate (PG), and tert-butylhydroquinone (TBHQ) represent strong antioxidant activities and, therefore, are being used to remove free radicals in food and biological systems (Haenen et al., Citation1996). However, because of the potential side effects, their applications are strictly controlled (Chen et al., Citation1992). In contrast, due to lower side effects of natural antioxidants, they are commonly isolated from food resources. Bioactive peptides as natural antioxidants are extracted from a large number of food hydrolysates such as dairy, egg, soy, and meat (Chen et al., Citation1995; Davalos et al., Citation2004; Saiga et al., Citation2003). These antioxidant peptides have been isolated from different proteins (Hook et al., Citation2001) including egg-yolk protein (Sakanaka & Tachibana, Citation2006), porcine proteins (Saiga et al., Citation2003), fish (Morimura et al., Citation2002; Shahidi & Amarowicz, 1996). In addition, the isolated peptides exhibited various biological activities such as antihypertension (Byun & Kim, Citation2001), immunomodulation (Tsuruki et al., Citation2003), hypocholesterolemic (Zhong et al., Citation2007), anti-cancer, anti-diabetic (Elias et al., Citation2008), and antimicrobial action (Boman, Citation1991) representing multifunctional activity of bioactive peptides.
Egg white, or albumen, constitutes almost 90% water and 10% proteins. The predominant proteins in egg white are ovalbumin, ovotransferrin, ovomucoid, ovomucin, lysozyme, and globulin (Li-Chan et al., Citation1995). In comparison with other eggs, ostrich (Struthio camelus) egg possesses a high amount of food proteins, and thus plays an important role in nutrition. Some antioxidant peptides have been reported in lecithin-free egg yolk hydrolysate (Park et al., Citation2001). Four peptides possessed high radical scavenging activity and ACE inhibitory effect has been reported from ovalbumin hydrolysate (Davalos et al., Citation2004). The objective of the present study was to investigate antioxidant activity of the ostrich egg white hydrolysate and peptide isolated from enzymatic hydrolysis, and also the ability of peptide in repairing wounds.
Materials and methods
Samples and chemicals
Pepsin (porcine gastric mucosa, EC 3.4.23.1), pancreatin (porcine pancreas), 1,1-diphenyl-2-picrylhydrazyl (DPPH), glutathione (GSH), 2,2′-azino-bis(3-ethylbenzothiazoline-6-sulphonicacid) (ABTS), potassium persulfate, potassium phosphate, trichloroaceticacid (TCA), ferric chloride, 3-(4,5-dimethylthia-zol-2-yl)-2,5-diphenyltetrazolium bromide (MTT), ethylenediaminetetraacetic acid (EDTA), and trifluoroacetic acid (TFA; for spectroscopy) were purchased from Sigma Chemicals Co. (St. Louis, MO). Ultrafiltration membranes with a 3 kDa cut off were procured from Millipore (Bedford, MA).
Preparation of egg white protein hydrolysates
Ostrich egg white (100 ml) was carefully mixed with 400 ml of an appropriate buffer described below and then homogenized for 10 min at room temperature. The mixture was separately hydrolyzed using various enzymes at optimum pH values (2.5 for pepsin and 7.5 for pancreatin). Pepsin at ratio of 1:20 (w/w, enzyme to substrate) was added and incubated for 120 min at 37 °C. Thereafter, the pH was adjusted to 7.5 and pancreatin at the same ratio was added for further digestion.
During the hydrolysis process, an aliquot was withdrawn at different intervals (0, 0.5, 1, 2, 4, 6, 8, and 10 h). In order to inactivate the enzymes, the aliquot of the reaction mixtures was heated at 90 °C for 10 min. An aliquot at onset of digestion was taken as non-hydrolyzed sample. Hydrolysates were clarified by centrifugation at 10 000 g for 15 min and then kept at −20 °C for subsequent analyses.
Ultrafiltration and RP-HPLC
The resultant hydrolysates were passed through an ultrafiltration membrane with a cut-off of 3 kDa. The filtrated sample was fractionated using RP-HPLC (reverse-phase high-performance liquid chromatography) with a semi-preparative C8 column (10 × 250 mm, manufactured by Macherey-Nagel GmbH & Co., Duren, Germany). The mobile phase included eluent A, which was composed of 0.1% TFA (trifluoroacetic acid) in distilled water (v/v), and eluent B, which was composed of 0.098% TFA in acetonitrile. The elution was accomplished by a linear gradient of 5–50% eluent B with a flow rate of 2 ml/min for 45 min. The absorbance of the eluted peaks was recorded at 214 nm using a UV detector. The most active fraction was further purified by an analytical RP-HPLC column (4.6 mm × 250 mm) using a linear gradient of 0.8% eluent B/min.
Peptide sequencing
The peptide with the highest antioxidant activity was characterized to identify its amino acid sequence. The sample was analyzed by the MALDI-TOF mass spectrometer using a 5800 Proteomics Analyzer (Applied Biosystems at Proteomics International Pty. Ltd., Nedlands, Western Australia). The amino acid sequence was determined by the de novo sequencing method. The PEAKS Studio Version 4.5 SP2 (Bioinformatics Solutions Inc., Waterloo, ON, Canada) was used for analysis of the obtained MS/MS spectra.
Determination of antioxidant activity
DPPH radical scavenging
The DPPH free radical, which is stable in ethanol, shows a maximum absorbance at 517 nm. When the DPPH radical encounters a proton-donating substance such as an antioxidant, the radical is scavenged and the absorbance is reduced (Zhang et al., Citation2010). The DPPH free radical scavenging activity was performed according to the method described by Chen et al. (Citation1996). Different concentrations of hydrolysate and peptide (0–1 mg/ml) were prepared using distilled water. 0.5 ml of DPPH solution (40 µM in ethanol) was added to 0.5 ml of sample and incubated at 25 °C in the dark for 30 min and then measured for absorbance at 517 nm. Glutathione (GSH) was used as a positive control and a sample mixture composing of 0.5 ml water and 0.5 ml DPPH was used as a control. All measurements were carried out in triplicate and then averaged. The scavenging capacity (SC50) value of radical DPPH was calculated according to the following equation:
where AS is the absorbance of sample at 517 nm, and AC is the absorbance of control at 517 nm. This indicates that the lower the SC50, the higher the free radical scavenging ability becomes.
Superoxide radical scavenging
The superoxide anion scavenging was measured by Pownall et al. (Citation2010). In a 96-well plate, an aliquot (80 µl) of peptide and protein hydrolysate sample (at an initial concentration of 1 mg/ml) was mixed directly with 80 µL of solution 1 mM EDTA and 50 mM Tris-HCl buffer (pH 8.3). Then, 40 µl of 1.5 mM pyrogallol (dissolved in 10 mM HCl) was added to each well and the plate was placed in the dark. The reaction rate (ΔA/min) was monitored by the absorbance of 420 nm for 4 min at room temperature. A sample containing the aforementioned buffer without peptide or hydrolysate was used as a control. GSH was used as a positive control (PC) and the superoxide radical scavenging activity was calculated using the equation below:
Hydroxyl radical scavenging activity
The hydroxyl radical scavenging assay was performed by a method described by Girgih et al. (Citation2011) with slight modification, in which a component of peptide (1 mg/ml), GSH (1 mg/ml), and 1,10-phenanthroline (3 mM) were separately dissolved in 0.1 M phosphate buffer (pH 7.4), while FeSO4 (3 mM) and 0.01% hydrogen peroxide were dissolved in distilled water. An aliquot (50 μl) of peptide or GSH or buffer (control) was added to a 96-well plate followed by the addition of 50 μl of 1,10-phenanthroline and 50 μl of FeSO4. To initiate reaction, 50 μl of hydrogen peroxide (H2O2) solution was added to the mixture and incubated at 37 °C for 1 h. Subsequently, the absorbance of the mixtures was measured at 536 nm for a period of 1 h. The hydroxyl radical scavenging activity was calculated as follows based on the absorbance (ΔA):
ABTS radical scavenging
ABTS radical scavenging activity of the peptide and protein hydrolysate was determined according to the method of Tironi and Añón (Citation2010) with slight modification. Briefly, the ABTS+ solution was produced by the reaction of 7 mM of ABTS solution in 2.45 mM potassium per sulfate (final concentration). The mixture was kept in the dark at room temperature for 16 h before use. The solution was diluted with distilled water and equilibrated at room temperature to give an absorbance of 0.70 ± 0.02 at 734 nm. Afterwards, 200 μl of the sample (1 mg/ml) was added to 2 ml of ABTS+ solution and absorbance at 734 nm was measured after 0 and 20 min. An appropriate solvent blank (negative control NC) was run for each assay with GSH used as a positive control (PC). The scavenging percentage was calculated using the equation below:
where Abst and Abs0 are the absorbance of the sample at t = 20 min and t = 0 min, respectively and Abct and Abc0 are absorbance of the negative control at t = 20 min and t = 0 min, respectively.
Copper-chelating activity
The ability of peptide and protein hydrolysate to chelate pro-oxidative Cu2+ was investigated according to Zhu et al. (Citation2008). In practice, 1 ml of 2 mM CuSO4 was mixed with 1 ml of pyridine (pH 7.0) and 20 µl of 0.1% (w/v) pyrocatechol violet. After adding 1 ml of samples (2 mg/ml), the disappearance of the blue color due to dissociation of Cu2+ was recorded by measuring the absorbance at 632 nm for 5 min. An equivalent volume of distilled water instead of the sample was used as blank. EDTA (ethylenediaminetetraacetic acid) was used as a positive control (PC) and the Cu2+ chelating activity was calculated as follows:
where AS is the absorbance of the sample and A0 is the absorbance of blank solution.
Cell culture and cytotoxicity assay
The HFLF-PI 5 cell line (C-169) derived from human lung tissue was purchased from the National Cell Bank of Iran (Pasteur Institute of Iran, Tehran, Iran). Cells were cultured in RPMI containing 10% (v/v) fetal bovine serum and 100 U/ml penicillin and streptomycin in a fully humidified atmosphere at 5% CO2 and 37 °C.
The cytotoxicity assay was performed according to the method of Gu et al. (Citation2012). In order to determine the radical-induced cytotoxicity, nearly 5 × 103 cells per well in RPMI medium were poured into a transparent 96-well plate and pre-incubated at 5% CO2 and 37 °C for 24 h. The cells were treated at different concentrations (0, 0.015, 0.03, 0.06, 0.125, 0.25, 0.5, and 1 mg/ml) of peptide and protein hydrolysate dissolved in the medium, and then both incubated for 24 h, 48 h, and 72 h. Cell viability was determined using 3-(4,5-dimethylthiazol-2-yl)-2,5-diphenyl tetrazolium bromide (MTT) in which the cell’s succinate dehydrogenase was able to convert MTT into visible formazan crystals (Mosmann, Citation1983). Cells in each well were rinsed with phosphate-buffered saline (PBS) and the supernatant was discarded, then 90 µL RPMI and 10 µl MTT (0.5 mg/ml final concentration) were added and incubated at 37 °C in the dark for 4 h. Eventually, the medium with MTT was replaced with 150 µl dimethyl sulfoxide (DMSO) to solubilize the formazan crystals, and the absorbance was read at 570 nm using a 96-well ELISA plate reader (ELX800 ELISA reader; Bio-Tek Instruments, Winooski, VT).
H2O2-induced cytotoxicity
To determine the radical-induced cytotoxicity of synthetic peptide, cells were seeded in a transparent 96-well plate of 5 × 103 cells/well and pre-incubated with RPMI for 24 h, then cells were separately treated with synthetic peptide at different concentrations (0–1 mg/ml) in the presence and absence of 700 µM H2O2 (final concentration). A similar test was performed for cells treated with GSH (at 0–1 mg/ml) as control in which the cells were subjected to the presence/absence of 700 µM H2O2 (final concentration). The treated cells were incubated at 37 °C for 2 h. Cell viability was then determined using the MTT method.
Wound-healing assay
Adult Wistar male rats (n = 36) were obtained from Razi Vaccine and Serum Research Institute (Mashhad, Iran). They were randomized into three groups (n = 12): animals without any treatment considered as the control group (C group), animals which were treated with ointment (O group), and the experimental group, which were animals treated with peptide (P group). The animals were anesthetized with intraperitoneal injection of ketamine (100 mg/kg) and the hair on the dorsal part of the rats were shaved and cleaned with 70% alcohol. A paravertebral excision (1 cm diameter) was made with a sterile surgical blade through the full thickness of the skin. In the O group, animals were treated with ointment alone by applying ointment on each wound with a sterile swab. Animals in the P group were treated with a mixture of ointment and peptide with a sterile swab. To prepare the sample, 1 mg of DG-10 peptide was added to 1 mg of ointment. The ointment was prepared according to the method described by Mashreghi et al. (Citation2013). Treatments were performed in 24 h intervals. To evaluate wound-healing properties of DG-10 peptide, wounds were investigated macroscopically. In macroscopic methods, the percentage of wound closure was measured at defined intervals (0, 3, 7, and 14 d) after creation of the wounds. Images were taken from each wound with digital camera (Powershot S2IS Canon, Canon Corp., Tokyo, Japan). Subsequently, the selected wound area of images was calculated with the imaging analysis program Image J NIH. The method of calculation of wound contraction was as follows:
All experimental protocols were approved by the local institutional committee for animal ethics and also were performed according to the international rules considering animal rights.
Statistical analysis
All assays, except the wound healing assay, were carried out in triplicate. Data were analyzed using the SPSS 13.0 software (SPSS Inc., Chicago, IL). One-way analysis of variance (ANOVA) was done to determine the significance of the main effects using least significant difference (LSD) procedures. The level of significance was set at p < 0.05.
Results
The present study was carried out to evaluate antioxidant activity of ostrich egg white hydrolysate (OEWPH) with pepsin and pancreatin and its isolated peptide as well. As shown in , HPLC analysis of OEWPH showed only one major peak which appeared in the 26th min (31% solvent B). The major peak was collected and identified by MALDI-TOF (matrix-assisted laser desorption/ionization-time of flight). As shown in and , tandem mass analysis showed that the peptide had the sequence of DAESLSRLLG (MW: 1060.18 ± 0.5 Da).
Figure 1. (a) Semi-preparation of OEWPH on C8 column (10 × 250 mm). Nearly 15 mg of OEWPH was dissolved in solvent A (water containing 0.1 % TFA) and was injected on the column. By monitoring the absorbance at 214 nm, the elution was accomplished at a flow rate of 2 ml/min using a linear gradient of acetonitrile as solvent B (5–45 % for 40 min). The major peak which appeared in the 26th min was picked out to be identified by tandem mass sequencing. The axis Y indicates milli-absorbance unit (mAU). (b) Identification of the molecular mass and amino acid sequence of the major peak of HPLC peptide using MALDI-TOF (matrix-assisted laser desorption/ionization-time of flight) spectrometer. MS/MS spectra of the major peak and the interpretation of the obtained spectrum (c).
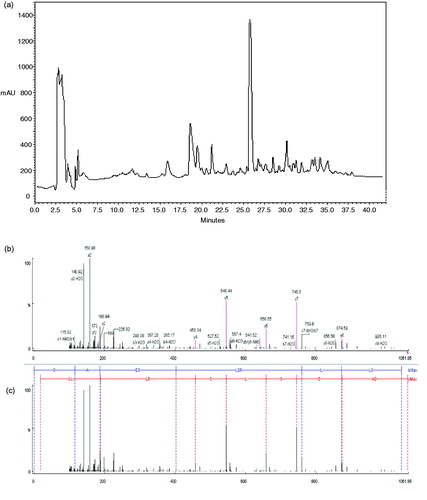
DPPH radical-scavenging activities of the peptide and OEWPH were tested. shows the degree of DPPH scavenging of protein hydrolysate and synthetic peptide. Both samples were capable of scavenging DPPH radicals. DPPH scavenging activity of peptide, protein hydrolysate, and GSH as control (at 1 mg/ml) were found to be 85.35, 88.3, and 100%, respectively. The DPPH radical scavenging activity of protein hydrolysate was higher than the peptide.
Figure 2. Antioxidant activity assays of the peptide and OEWPH and GSH. (a) DPPH radical scavenging activities. (b) Superoxide radical scavenging activities. (c) Hydroxyl radical scavenging activities. (d) ABTS radical scavenging activities. (e) Metal chelating effects. Values were means ± SE of triplicate experiments. Data were significantly different from the control at p < 0.05 with the one-way ANOVA test.
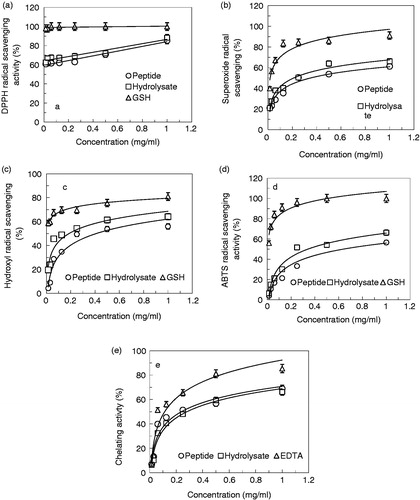
Superoxide radical-scavenging activity of the peptide and OEWPH demonstrated that although both samples are effective in superoxide scavenging activity, OEWPH has a stronger activity than peptide (). The scavenging effects of GSH, peptide, and protein hydrolysate (at 1 mg/ml) on superoxide radical were 91, 61.29, and 66.12%, respectively. The IC50 values of superoxide radical scavenging activity of peptide and hydrolysate were 0.36 mg/ml and 0.24 mg/ml, respectively.
shows the hydroxyl radical scavenging effects of peptide and OEWPH. Results showed that although both samples have antioxidant activity, the protein hydrolysate has more antioxidant activity compared with the peptide. Hydroxyl radical scavenging effects of GSH, peptide, and OEWPH (each at 1 mg/ml) were 80.97, 55.97, and 66.13%, respectively. The IC50 values of hydroxyl radical scavenging activity peptide and OEWPH were 0.4 and 0.22 mg/ml, respectively, whereas this parameter for GSH was lower than 0.015 mg/ml.
As depicted in , both peptide and OEWPH had potent antioxidant activity against ABTS radicals. There was a significant difference between ABTS radical scavenging of OEWPH in comparison with the peptide (p < 0.05). The scavenging values of GSH, peptide, and protein hydrolysate (at 1 mg/ml) on ABTS radical scavenging were 100, 56.59, and 66.25%, respectively. Furthermore, the IC50 values of hydroxyl radical scavenging activity peptide and hydrolysate were 0.56 and 0.36 mg/ml, respectively. GSH as a positive control showed an IC50 value lower than 0.015 mg/ml.
To further evaluate antioxidant activity of peptide and OEWPH, Cu2+ chelating was performed. As shown in , the chelating activity of OEWPH and identified peptide decreased in both samples although there is no significant difference between the two samples (p < 0.05). The antioxidant activities of EDTA, peptide, and OEWPH at 1 mg/ml on Cu2+ chelating were obtained to be 85.45, 68.91, and 66.21%, respectively. The peptide and OEWPH displayed IC50 values of 0.28 and 0.32 mg/ml, respectively.
As shown in , the influence of peptide on HFLF-PI 5 cell viability was evaluated by MTT assay at 24, 48, and 72 h. The results showed that DG-10 peptide significantly has no cytotoxicity effect on HFLF-PI 5 cell line (p < 0.05) over a period of time. HFLF-PI 5 cell line treated with H2O2 showed that hydrogen peroxide has concentration-dependent decrease on cell viability, while the synthetic peptide exhibited an effective protection on H2O2-induced cell line oxidation. The identified peptide had a dose-dependent protective effect on cell survival enhancement ( and ).
Figure 3. Influence of DG-10 peptide on HFLF cell viability by the MTT assay. Control consists of non-treated cells.
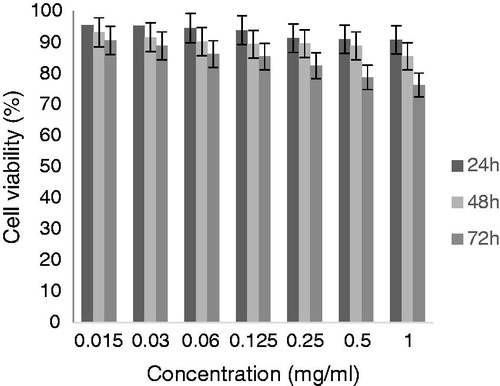
Figure 4. The cytotoxicity of H2O2 on viability in normal cells. Cells were treated with H2O2 at the indicated concentrations (from 0.2 to 1 mM) and after 2 h, the cell viability was assessed by MTT assay. Values were means ± SE of triplicate experiments. Data were significantly different from the control at p < 0.05 with repeated measure tests.
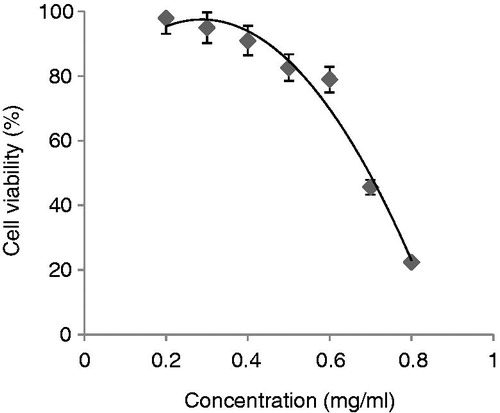
Figure 5. Protective effect of DG-10 peptide on H2O2-induced oxidative damage in normal cells. The viability of cells after H2O2 treatment was assessed by the MTT assay.
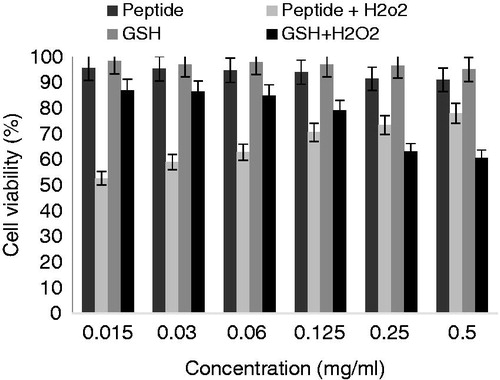
To evaluate the wound repair ability of peptide, we calculated the percentage of wound closure by comparing wounds of each group on days 3, 5 and 11 post-injuries with the time of wound induction. However, the differences in wound contraction were significantly increased in wounds that were treated with peptide compared with control and O groups. As shown in , the percentage of wound closure on day 3 was 34% compared with control and O groups that were 25.5 and 30.1%, respectively. On day 7 post-injury, the percentage of wound closure for control, O, and P groups were 34, 44.3, and 65%, respectively. The percentage of wound closure in the P group at day 14 was 98%. In all three days of study, comparison between the P group and the control group was significant.
Discussion
As reported previously, an antioxidant peptide with the sequence of LTEQESGVPVMK (MW: 1317.65 Da) was obtained from enzymatic hydrolysis of ostrich egg white (Tanzadehpanah et al., Citation2012). However, the hydrolysis procedure used in current study was different from that of the previous study in which α-chymotrypsin, pepsin, trypsin, and papain were used. Up to now, no other peptide has been reported for OEWPH in literature.
The identified peptide was synthesized by solid phase procedure and pure peptide was obtained by C8 reverse phase-HPLC using a semi-preparative column (data not shown). The purified synthetic peptide as well as OEWPH was investigated to examine its antioxidant activity.
DPPH is a stable free radical and accepts an electron or hydrogen radical to become a stable diamagnetic molecule. The hydroxyl radical possessed the strongest chemical activity among the reactive oxygen species (ROS), and easily reacted with biomolecules such as amino acids, proteins, and DNA (Peñta-Ramos & Xiong, Citation2002; Rajapakse et al., Citation2005).
Chelation of metal ions has an antioxidant effect because copper catalyzes the generation of reactive oxygen species, including hydroxyl radical and superoxide radical, leading to oxidation of unsaturated lipids and promoting oxidative damage at different levels (Saiga et al., Citation2003). The copper chelating activity in these fractions might be related to their content of negatively charged amino acids (Asp and Glu). Various amino acid residues may be responsible for the antioxidant activity in peptides, which is usually due to chelation of transition metals and scavenging of free radicals (Chen et al., Citation1996).
Generally, it may be concluded that the efficiency of protein hydrolysate versus peptide can be the result of other existing peptides in hydrolysate and/or can be due to the existence of synergism between peptides.
It has been shown that the presence of acidic amino acids such as aspartic and glutamic acid and basic amino acids such as arginine are related with peptide antioxidant activity (Qian et al., Citation2008; Suetsuna et al., Citation2000). Moreover, it is reported that hydrophobic amino acids such as alanine, leucine, and glycine enhance the solubility of peptide in lipid and increase its radical scavenging activity (Chen et al., Citation1998; Qian et al., Citation2008). The DG-10 peptide exhibits antioxidant activity properties due to the presence of the aforementioned amino acids in its sequence.
Radical scavenging activities were also evaluated in radical-mediated cellular injuries as a system (Li et al., Citation2008). Hence, the protection effect of synthetic peptide on HFLF-PI 5 cell line under H2O2 oxidative stress was examined. The cell culture was exposed to H2O2-inducing oxidative damage and cytotoxicity in the presence and absence of peptide was determined by the MTT assay. Our results indicate that H2O2 has concentration-dependent decrease on cell viability. Although, hydrogen peroxide (H2O2) was able to induce significantly decreased cell viability as compared with the control, the synthetic peptide exhibited an effective protection on H2O2-induced cell line oxidation. The identified peptide had a dose-dependent protective effect on cell survival enhancement ( and ). Previous reports showed that small di- and tripeptides can easily enter the cell and prevent cell death by enzymatic pathway or scavenging free radicals (Gu et al., Citation2012; Vermeirssen et al., Citation2002).
It has been shown that YECG demonstrated the best resistance to H2O2-induced cells necrosis. The presence of antioxidant amino acids such as His or Tyr, and their position in the peptide chain (C-/N- terminal) could affect the antioxidant capacity of the peptides (Gu et al., Citation2012).
Wound repair is a complex process that is involved in cellular and molecular events. It has three phases: inflammation, tissue formation, and tissue rearrangement (Singer & Clark, Citation1999). Healing effects of this peptide can be indicated by its antioxidant effects.
Conclusions
Results of the present study revealed that OEWPH and the identified peptide had antioxidant activity. Our in vitro study represented that OEWPH and the derived peptide could scavenger DPPH, ABTS, superoxide, and hydroxyl radicals. Owing to the presence of hydrophobic amino acids in the sequence peptide and hydrolysate, DPPH radical scavenging was more potent than that of ABTS. Results clearly demonstrated that the identified peptide had a protective effect against H2O2-induced cytotoxicity and also displayed a wound healing property. In conclusion, the isolated peptide from OEWPH exhibited potent and effective antioxidant agent. Further studies will be required to define mechanisms involved in the wound healing feature as well as protective effects of peptide against cellular oxidative stress.
Declaration of interest
All authors declare that there is no conflict of interest. This work was supported by Science and Research Branch, Islamic Azad University, Tehran, Iran and Ferdowsi University of Mashhad, Mashhad, Iran and, therefore, is greatly appreciated by the authors.
References
- Bedard K, Krause KH. (2007). The NOX family of ROS-generating NADPH oxidases: Physiology and pathophysiology. Physiol Rev 87:245–313
- Boman HG. (1991). Antibacterial peptides: Key components needed in immunity. Cell 65:205–7
- Byun HG, Kim SK. (2001). Purification and characterization of angiotensin I converting enzyme (ACE) inhibitory peptides from Alaska pollack (Theragra chalcogramma) skin. Process Biochem 36:1155–62
- Chen C, Pearson AM, Gray JI. (1992). Effects of synthetic antioxidants (BHA, BHT and PG) on the mutagenicity of IQ-like compounds. Food Chem 43:177–83
- Chen HM, Muramoto K, Yamauchi F. (1995). Structural analysis of antioxidative peptides from soybean beta-conglycinin. J Agric Food Chem 43:574–8
- Chen HM, Muramoto K, Yamauchi F, Nokihara K. (1996). Antioxidant activity of designed peptides based on the antioxidative peptide isolated from digests of a soybean protein. J Agric Food Chem 44:2619–23
- Chen HM, Muramoto K, Yamauchi F, et al. (1998). Antioxidative properties of histidine-containing peptides designed from peptide fragments found in the digests of a soybean protein. J Agric Food Chem 46:49–53
- Davalos A, Miguel M, Bartolome B, Lopez-Fandino R. (2004). Antioxidant activity of peptides derived from egg white proteins by enzymatic hydrolysis. J Food Protect 67:1939–44
- Elias RJ, Kellerby SS, Decker EA. (2008). Antioxidant activity of proteins and peptides. Crit Rev Food Sci 48:430–41
- Girgih A, Udenigwe C, Aluko R. (2011). In vitro antioxidant properties of hemp seed (Cannabis sativa L.) protein hydrolysate fractions. J Am Oil Chem Soc 88:381–9
- Gu L, Zhao M, Li W, et al. (2012). Chemical and cellular antioxidant activity of two novel peptides designed based on glutathione structure. Food Chem Toxicol 50:4085–91
- Haenen HE, Bleijlevens E, Elzerman H, et al. (1996). Cytotoxicity of 2-tert-butyl hydroquinone glutathione conjugates after apical and basolateral exposure of rat renal proximal tubular cell monolayers. Toxicol In Vitro 10:141–8
- Halliwell B. (1994). Free radicals, antioxidants, and human disease: Curiosity, cause, or consequence?. Lancet 344:721–4
- Harman D. (2001). Aging: Overview. Ann NY Acad Sci 928:1–21
- Hook VY, Burton D, Yasothornsrikul S, et al. (2001). Proteolysis of ProPTHrP(1-141) by “prohormone thiol protease” at multibasic residues generates PTHrP-related peptides: Implications for PTHrP peptide production in lung cancer cells. Biochem Biophys Res Co 285:932–8
- Kim SK, Wijesekara I. (2010). Development and biological activities of marine-derived bioactive peptides: A review. J Funct Foods 2:1–9
- Leung PS, Chan YC. (2009). Role of oxidative stress in pancreatic inflammation. Antioxid Redox Signal 11:135–65
- Li-Chan ECY, Powrie WD, Nakai S. (1995). The chemistry of eggs and egg products. In: Stadelman WJ, Cotterill OJ, eds. Egg Science and Technology, 4th ed. New York: Haworth Press
- Li Y, Jiang B, Zhang T, et al. (2008). Antioxidant and free radical-scavenging activities of chickpea protein hydrolysate (CPH). Food Chem 106:444–50
- Mashreghi M, Rezazade Bazaz M, Mahdavi Shahri N, et al. (2013). Topical effects of frog “Rana ridibunda” skin secretions on wound healing and reduction of wound microbial load. J Ethnopharmacol 145:793–7
- Morimura S, Nagata H, Uemura Y, et al. (2002). Development of an effective process for utilization of collagen from livestock and fish waste. Process Biochem 37:1403–12
- Mosmann T. (1983). Rapid colorimetric assay for cellular growth and survival: Application to proliferation and cytotoxicity assays. J Immunol Methods 65:55–63
- Park PJ, Jung WK, Nam KS, et al. (2001). Purification and characterization of antioxidative peptides from protein hydrolysate of lecithin-free egg yolk. J Am Oil Chem Soc 78:651–6
- Peñta-Ramos EA, Xiong YL. (2002). Antioxidant activity of soy protein hydrolysates in a liposomal system. J Food Sci 67:2952–6
- Pownall TL, Udenigwe CC, Aluko RE. (2010). Amino acid composition and antioxidant properties of pea seed (Pisum sativum L.) enzymatic protein hydrolysate fractions. J Agric Food Chem 58:4712–18
- Qian ZJ, Jung WK, Kim SK. (2008). Free radical scavenging activity of a novel antioxidative peptide purified from hydrolysate of bullfrog skin, Rana catesbeiana Shaw. Bioresour Technol 99:1690–8
- Rajapakse N, Mendis E, Jung WK, et al. (2005). Purification of a radical scavenging peptide from fermented mussel sauce and its antioxidant properties. Food Res Int 38:175–82
- Saiga A, Tanabe S, Nishimura T. (2003). Antioxidant activity of peptides obtained from porcine myofibrillar proteins by protease treatment. J Agric Food Chem 51:3661–7
- Sakanaka S, Tachibana Y. (2006). Active oxygen scavenging activity of egg-yolk protein hydrolysates and their effects on lipid oxidation in beef and tuna homogenates. Food Chem 95:243–9
- Shahidi F, Zhong Y. (2010). Novel antioxidants in food quality preservation and health promotion. Eur J Lipid Sci Technol 112:930–40
- Singer AJ, Clark RA. (1999). Cutaneous wound healing. New Engl J Med 341:738–46
- Suetsuna K, Ukeda H, Ochi H. (2000). Isolation and characterization of free radical scavenging activities peptides derived from casein. J Nutr Biochem 11:128–31
- Tanzadehpanah H, Asoodeh A, Chamani J. (2012). An antioxidant peptide derived from Ostrich (Struthio camelus) egg white protein hydrolysates. Food Res Int 49:105–11
- Tironi VA, Añón MC. (2010). Amaranth proteins as a source of antioxidant peptides: Effect of proteolysis. Food Res Int 43:315–22
- Tsuruki T, Kishi K, Takahashi M, et al. (2003). Soymetide, an immunostimulating peptide derived from soybean beta-conglycinin, is an fMLP agonist. FEBS Lett 540:206–10
- Vermeirssen V, Van Camp J, Verstraete W. (2002). Optimisation and validation of an angiotensin-converting enzyme inhibition assay for the screening of bioactive peptides. J Biochem Biophys Methods 51:75–87
- Zhang J, Zhang H, Wang L, et al. (2010). Isolation and identification of antioxidative peptides from rice endosperm protein enzymatic hydrolysate by consecutive chromatography and MALDI-TOF/TOF MS/MS. Food Chem 119:226–34
- Zhong F, Liu J, Ma J, Shoemaker CF. (2007). Preparation of hypocholesterol peptides from soy protein and their hypocholesterolemic effect in mice. Food Res Int 40:661–7
- Zhu L, Chen J, Tang X, Xiong YL. (2008). Reducing, radical scavenging, and chelation properties of in vitro digests of alcalase-treated zein hydrolysate. J Agric Food Chem 56:2714–21