Abstract
Context: Boerhavia diffusa L. (Nyctaginaceae) roots are used in Ayurveda for treating inflammatory diseases. Generally poor oral bioavailability is a major problem associated with herbal drugs.
Objective: To develop a phospholipid complex of rotenoid-rich fraction (RRF) and evaluate its in vivo anti-inflammatory activity and pharmacokinetic study.
Materials and methods: RRF was prepared from a 70% ethanol extract of B. diffusa roots. This RRF was complexed with phosphatidylcholine by refluxing in 70% ethanol. In vivo anti-inflammatory activity of RRF-PC and RRF was determined using the carrageenan-induced rat paw edema method, at a dose equivalent to 100 mg/kg p.o. of RRF. Edema volume was calculated at 3 and 5 h. The plasma concentration of boeravinone B was estimated in rats at a same dose level. Blood samples were collected at 1, 2, 4, 6, 8, 10, 12, 24, and 36 h.
Results: 1H and 31P NMR spectra of RRF-PC showed up-field shift of protons of the +N(CH3)3 group (3.37 → 3.23) and the phosphorus atom (−1.26 → −1.57 ppm), respectively, which confirmed phospholipid complex formation between phosphatidylcholine [PO4 and +N(CH3)3 groups] and phytoconstituents by hydrogen bonding. The RRF-PC showed significantly enhanced in vivo anti-inflammatory activity (64%) as compared with RRF (48%) and ibuprofen (50%) at 5 h (p < 0.001). Furthermore, detected plasma concentration of boeravinone B was two times higher in RRF-PC (75 ng/mL) as compared with RRF (40 ng/mL).
Conclusion: The present study demonstrated an increased anti-inflammatory potential and higher plasma level of boeravinone B in lipid-based formulation (RRF-PC) as compared with RRF.
Introduction
Herbal medicines are being used by about 80% of the world population, primarily in the developing countries for their primary health care. These are relatively safer than synthetic drugs and have been gaining popularity in recent years. Phytoconstituents present in herbal drugs are part of physiological functions of living flora. Therefore, they are supposed to have better compatibility with the human body (Kamboj et al., Citation2000). However, poor oral bioavailability of phytoconstituents has become a concern for investigators. Some reasons for poor oral bioavailability of these plant actives are high molecular weight, low aqueous or lipid solubility, degradation of some phytoconstituents in the presence of gastric fluids, and poor permeability across plasma membrane (Khan et al., Citation2013).
To overcome these hurdles and to increase the efficacy of herbal medicine, these have been formulated into various novel delivery systems such as phytosome, liposome, and nanoparticles. Phytosome formulation is better alternative for herbal medicines that contain a mixture of several phytoconstituents. It is the complex of a phytoconstituent with phospholipid in a stoichiometric ratio (Khan et al., Citation2013). Phospholipid is the principal component of the eukaryotic cell membrane and has a good solubilizing property; hence, it can easily cross the cell membrane along with the complexed phytoconstituents, thereby increasing the bioavailability of phytoconstituents. Further, as the bioavailability increases, the dose requirement is reduced, which has an added advantage where toxicity arises due to high doses of phytoconstituents (Semalty et al., Citation2010). The phospholipid complex with the polar group of phytoconstituents is formed by some physicochemical interactions like hydrogen bonding between two polar groups: one from phosphatidylcholine (quaternary nitrogen or oxygen atom attached to the phosphate group) and another from flavonoids or polyphenolics (Jain et al., Citation2010). Several phosphatidylcholine complexes with herbal drug extracts or actives have been reported in the literature, namely phytosome formulation of Ginkgo biloba L. (Ginkgoaceae), ginseng, curcumin, quercetin, silybin, etc. (Ajazuddin & Saraf, Citation2010).
Boerhavia diffusa Linn. (Nyctaginaceae) is a perennial herbaceous plant, found in tropical parts of India, South America, and Africa. In Ayurveda, B. diffusa roots are used for the treatment of various diseases including jaundice, dyspepsia, nephrotic syndrome, convulsion, enlargement of spleen, abdominal pain, stress, and inflammation (Kirtikar & Basu, Citation1956). Punarnavashtak kwath, an Ayurvedic formulation of B. diffusa, was also reported to possess hepatoprotective activity against CCl4-induced hepatotoxicity in rats and on HepG2 cell line (Shah et al., Citation2011). The methanol extract of B. diffusa (whole plant) showed anticonvulsant activity (Adesina et al., Citation1979), antiproliferative and antiestrogenic effects (Sreeja, Citation2009). The corresponding ethanol extract was reported to exhibit anti-inflammatory (Bhalla, Citation1971), hepatoprotective (Rawat, Citation1997), immunomodulatory (Mehrotra, Citation2002), and antidepressant activities (Dhingra et al., Citation2014). An aqueous extract of B. diffusa (leaves) exhibited anti-diabetic (Pari, Citation2004) and antioxidant activities (Pareta et al., Citation2011).
Earlier phytochemical investigations of B. diffusa reported that it contains diverse classes of chemical constituents including phenolics: quercetin 3-O-rhamnosyl(1 → 6)galactoside (quercetin 3-O-robinobioside), quercetin 3-O-(2″-rhamnosyl)-robinobioside, quercetin, kaempferol 3-O-(2″-rhamnosyl)-robinobioside, kaempferol 3-O-robinobioside, kaempferol, 3,4-dihydroxy-5-methoxycinnamoylrhamnoside, 3,5,4′-trihydroxy-6,7-dimethoxyflavone 3-O-galactosyl (1 → 2) glucoside [eupalitin 3-O-galactosyl (1 → 2) glucoside], caffeoyltartaric acid, and eupalitin 3-O-galactoside (Ferreres, Citation2005); punarnavoside (Jain, Citation1989); terpenoids: ursolic acid (Misra, Citation1971); and alkaloid: punarnavine (Saraswati, Citation2013). In addition, boeravinones A–S, a group of rotenoids, have been reported from 70% ethanol and methanol extracts of B. diffusa roots (Ahmed-Belkacem, Citation2007; Bairwa, Citation2013a,Citationb; Borrelli, Citation2005, Citation2006; Kadota, Citation1989; Lami, Citation1990, Citation1991a,Citationb). Boeravinone B, a major rotenoid of B. diffusa, has been reported to exhibit anticancer (Ahmed-Belkacem, Citation2007) and COX-1 and -2 inhibitory activities in vitro and anti-inflammatory activity in vivo (Bairwa, Citation2013a,Citationb).
Recently, we have prepared a rotenoid-rich fraction (RRF) from 70% ethanol extract of B. diffusa roots and both the extract and the RRF were subjected to in vivo anti-inflammatory activity. The RRF showed better anti-inflammatory activity when compared with the corresponding 70% ethanol extract (Bairwa, Citation2013a,Citationb). Keeping in mind of importance of B. diffusa in Ayurveda and a general problem of poor bioavailability of herbal extract, we herein wish to develop a lipid-based formulation (phospholipid complex) of RRF and evaluate it for in vivo anti-inflammatory activity and pharmacokinetic study.
Materials and methods
General
The hydrogenated soy phosphatidylcholine (PHOSPHOLIPON® 90H) was provided as a gift sample from Lipoid GmbH (Ludwigshafen am Rhein, DE, Germany); Ibuprofen was purchased from Sigma-Aldrich (St. Louis, MO); ethanol was obtained from Changshu Yangyuan Chemical, China; Diaion HP-20 resin was purchased from Sigma-Aldrich (St. Louis, MO); HPLC grade methanol was obtained from Merck KGaA (Darmstadt, Germany). 1H NMR spectra were recorded on Bruker 400 Ultra ShieldTM NMR spectrometer (Bruker Biospin AG, Faellanden, Switzerland) with TMS as an internal standard. FTIR spectra with ATR were recorded on a PerkinElmer FrontierTM spectrometer (PerkinElmer, Waltham, MA). The content of boeravinone B in the phospholipid complex was determined on Waters ACQUITY ultra-performance liquid chromatography (UPLC) H-Class system (Millipore, Billerica, MA), equipped with binary pump, auto sampler, photodiode array detector (PDA), and EmpowerTM3 software (Waters, Milford, MA). Separation was carried out on BEH Shield RP-18 column (2.1 × 100 mm, 1.7 µM) (Waters, Milford, MA). Pharmacokinetic study was performed on a Waters 600 HPLC system equipped with a Delta 600 quaternary solvent pump (Waters, Milford, MA), an in-line degasser, a 717 plus auto sampler, a temperature control module, and 2996 photodiode array (PDA) detector (Waters, Milford, MA). The rat paw volume was measured using a plethysmometer (model 7140, Ugo Basile, Comerio, Varese, Italy).
Animals
Female Sprague–Dawley rats (180–210 g) were used for the in vivo study. The Institute’s Animal Ethics Committee (IAEC), NIPER, approved the experimental protocol (IAEC/12/37), and the experiments were carried out in accordance with the Committee for the Purpose of Control and Supervision of Experiments on Animals (CPCSEA) guidelines. The animals were housed in plastic cages, and maintained under standard laboratory condition with a natural 12 h light and dark cycle, and were fed with standard pellet diet and water ad libitum.
Preparation of RRF
RRF was prepared as described by us (Bairwa, Citation2013a,Citationb). In brief, dried, powdered root material (1 kg) of B. diffusa was macerated with 70% ethanol, 3 × 1 L at room temperature each for 24 h. The extracts were collected, filtered, and then concentrated on a rotary evaporator under vacuum to 90 mL volume and finally freeze-dried to give 82 g dried extract (8.20% w/w). The dried 70% ethanol extract (40 g) of B. diffusa was subjected to column chromatography over Diaion HP-20 resin using gradient elution with H2O–MeOH. The fractions collected on elution with 90% and 100% MeOH were concentrated on a rotary evaporator under reduced pressure to give the 2.21 g of RRF.
Preparation of phosphatidylcholine complex (PC)
The RRF-PC was prepared by a simple solvent evaporation method by refluxing the RRF and PHOSPHOLIPONE® 90 H (phospholipid) in a ratio of 1:1 (w/w) as per the reported method (Yanyu, Citation2006). In brief, a weighed amount of Phospholipon® 90 H (200 mg) was dissolved in 10 mL of absolute ethanol and refluxed with continuous stirring for 30 min at 50 °C, in 50 mL RBF. In a separate vial, RRF (200 mg) was dissolved in 10 mL of 70% ethanol and kept at 50 °C for 15 min. Then the RRF solution was added drop-wise in the phospholipid solution and refluxed for another 3 h. The final solution was concentrated to precipitate phospholipid complex; obtained precipitates were washed with hexane and dried under reduced pressure. The prepared RRF-PC was transferred into a glass vial, wrapped with aluminum foil, and stored in refrigerator.
Determination of boeravinone B content in RRF and RRF-PC
The content of boeravinone B in RRF and RRF-PC was determined by the UPLC method (Bairwa et al., Citation2014). In brief, 5 mg of RRF-PC was dissolved in 1 mL methanol and filtered by syringe filter 0.2 µm (Advance Micro devices Private Limited, Ambala, India) and 2 µL was injected into the UPLC system. The mobile phase consisting of 0.1% acetic acid in water and methanol was used with a gradient elution. The separation was achieved on a BEH Shield RP-18 column and the flow rate was set to 0.35 mL/min. The peak area of boeravinone B obtained from RRF and RRF-PC samples was used in the linear regression equation (y = 14 840 x + 5942) to determine the content of boeravinone B in the respective samples.
Characterization of PC
The prepared PC was characterized by using 1H and 31P NMR spectroscopies (Gabetta, Citation1989). In brief, a weighed amount (20 mg) of RRF and RRF-PC (equivalent to 20 mg of RRF) were dissolved separately in CDCl3, and their 1H NMR spectra were recorded. Trimethylphosphine was used as an internal standard for 31P NMR. Furthermore, FTIR spectroscopy was used for confirmation of the phospholipid complex formation.
Scanning electron microscopy
The surface morphology of RRF-PC was determined by scanning electron microscopy. The analysis was carried out by placing RRF-PC on aluminum stubs using both sided adhesive tape and was made electrically conductive by coating with a thin film of gold, at 10 Torr vacuum, using the Hitachi Ion Sputter (JFC-1100, JEOL, Tokyo, Japan). The SEM images were analyzed with an electron beam of an acceleration potential of 1.2 kV and the images were collected as the secondary electron mode, for particle size analysis.
Particle size, PDI, and Zeta potential of RRF-PC
Mean particle size and polydispersity index (PDI) of RRF-PC were determined using the Zeta Sizer (Nano ZS, Malvern, UK); the Zeta potential was also determined by the Zeta Sizer (Nano ZS, Malvern, UK).
In vivo anti-inflammatory activity
Anti-inflammatory activity of RRF and RRF-PC was evaluated using the carrageenan-induced paw edema method described by Winter et al. (Citation1962). In brief, rats were fasted overnight before the start of the experiment. The animals were divided into four groups having six animals each (n = 6). Standard and test samples were prepared by suspending in freshly prepared 0.5% hydroxypropyl methyl cellulose solution. The control group was treated with vehicle (normal saline) only, and the positive control group was treated with the reference standard, ibuprofen at a dose of 100 mg/kg p.o. The remaining two groups were treated orally with RRF (100 mg/kg) and RRF-PC (equivalent to 100 mg/kg of RRF). Edema was induced by subcutaneous injection of 100 µL of 1% carrageenan in normal saline solution, into the left hind paw of rats 1 h after the drug administration. The paw volume was measured immediately before (basal) and after (3 h and 5 h) carrageenan injection using plethysmometer. Edema rate was calculated by subtracting initial paw volume from paw volume measured at 3 h and 5 h time points; and the % inhibition was calculated from this edema rate of the control and treated groups using following equations:
(1)
(2)
Here, Vt is the mean paw volume after carrageenan injection; V0 is the mean paw volume before carrageenan injection; Ec is the edema rate of the control group; Et is the edema rate of the treated group.
In vivo pharmacokinetic study
The plasma concentration of boeravinone B was determined by the HPLC method. Briefly, female Sprague–Dawley rats (180–210 g) were divided into two groups (n = 5) and fasted overnight before starting the experiment. Group A was treated with RRF at a dose of 100 mg/kg and group B was treated with RRF-PC at a dose equivalent to 100 mg/kg p.o. of RRF.
Extraction, sample preparation, and HPLC analysis
Blood samples (400 µL) were collected from the retro-orbital plexus under mild anesthesia into the centrifuge tubes (1 mL), containing 30 IU/mL of heparin, at an interval of 1, 2, 4, 6, 8, 10, 12, 24, and 36 h. Collected blood samples were centrifuged for 10 min at 10 000 rpm to obtain blood plasma, which was immediately stored at −80 °C until further use. A plasma volume of 200 µL was taken and mixed with 40 µL of 4-hydroxyacetophenone (internal standard, 2.5 µg/mL in methanol) and vortex for 10 min. Complete precipitation of plasma proteins was carried out by adding 400 µL methanol and vortex for 10 min. Precipitated proteins were removed by centrifugation at 10 000 rpm for 10 min. The supernatant was dried in vacuum centrifuge (Centri Vac, Inc., Hampton Bays, NY), and the drug was extracted by adding 100 µL of methanol and vortex for 10 min followed by centrifugation at 10 000 rpm for 10 min. From this, a volume of 70 µL was injected into the HPLC system. Separation was carried out on the C18 Xbridge column (4.6 × 250 mm, 5.0 µm) (Waters, Milford, MA), a mobile phase consisting of 0.1% acetic acid in water (solvent A) and methanol (solvent B) was used with a gradient elution profile as 0 min, 45% B; 5 min, 60% B; 12 min, 75% B; 25 min, 75% B; 30 min, 90% B; 50 min; 90% B, the flow rate was set to 1 mL/min, the detection was made at 273 nm, and the column temperature was set to 30 °C.
Pharmacokinetic parameters: Total area under the curve (AUC)0-∞, peak plasma concentration (Cmax), and maximum time required to reach the peak plasma concentration (Tmax) were determined by the one compartmental model, using a Kinetica software (Thermo Scientific, Waltham, MA).
Statistical analysis
In vivo activity data are expressed as mean% ± standard error mean (S.E.M). Statistical differences between the treatment and control groups were evaluated by one-way ANOVA followed by Dunnett's tests, **p < 0.01 was significant from the control; ***p < 0.001 was extremely significant from the control group.
Results
So far, there are no reports on universal and systematic preparation conditions for phospholipid complexes of any drug, because each drug has its own particular physicochemical properties. Therefore, a particular set of reaction condition cannot be applied universally for preparing phospholipid complexes. However, most published work on phospholipid complex formulation have used some general variables like drug–phospholipid ratio of 1:1, soya phosphatidylcholine as the most commonly used phospholipid, and refluxing with stirring for few hours as reaction conditions (Lu, Citation2009). In the present work, we have adopted these common variables and developed the phospholipid formulation of RRF.
Determination of boeravinone B in RRF-PC
RRF was found to contain 29.1 mg/g of boeravinone B. The % content of boeravinone B in RRF-PC was determined by using the following equation:
Here, the amount of boeravinone B in RRF-PC was multiplied by 2 because RRF-PC contains only 50% RRF (RRF/phosphatidylcholine = 1:1). The content of boeravinone B in phospholipid complex was found to be 71% w/w.
Characterization of RRF-PC
The complex formation between phytoconstituents and phosphatidylcholine was confirmed by measuring FTIR, 1H, and 31P NMR spectra. As shown in , a significant up-field shift was observed for the proton signals of the N(CH3)3 group (3.37 → 3.23 ppm), and there was no change in the chemical shift of protons of other groups of phosphatidylcholine. Similarly, the 31P NMR spectrum of RRF and RRF-PC also showed significant up-field shift (from δp −1.26 → δp −1.57 ppm) of phosphorous atom of phosphatidylcholine. The FTIR spectra of PC, RRF-PC, and RRF are shown in . The aliphatic phosphate (P = O) stretching vibration was observed at 1255 cm−1 with a strong absorption in the spectrum of phosphatidylcholine, but it is almost disappeared in the spectrum of RRF-phosphatidylcholine.
Morphology study of PC
The average particle size of PC was measured as 532 nm with the PDI value of 0.652. The surface morphology of RRF-PC was determined by SEM and observed as a spherical shape ( and ).
Figure 3. Scanning electron micrographs (SEM) of rotenoid-rich fraction-phospholipid complex (RRF-PC).
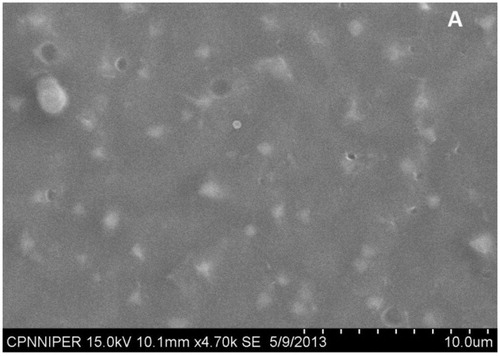
Table 1. Particle size, PDI, and Zeta potential of phospholipid formulation.
In vivo anti-inflammatory activity
The in vivo anti-inflammatory activity of the RRF and its phospholipid complex (RRF-PC) was studied using a carrageenan-induced rat paw edema assay. The result showed that RRF-PC exhibited enhanced anti-inflammatory effect when compared with RRF, at the same dose level of 100 mg/kg p.o. ().
Table 2. In vivo anti-inflammatory activity of phospholipid formulation.
In vivo pharmacokinetic study
Among several rotenoids, boeravinone B is a major one and a marker constituent of B. diffusa. It is also responsible for anti-inflammatory activity exhibited by RRF (Bairwa, Citation2013a,Citationb). Therefore, the plasma concentration of boeravinone B, after a single dose (100 mg/kg p.o.) administration of RRF and its PC, was determined and summarized in and . The data were analyzed by the one compartmental model, using the Kinetica software 4.4.1 (Thermo Scientific, Waltham, MA).
Figure 4. Pharmacokinetic study of rotenoid-rich fraction (RRF) and rotenoid-rich fraction-phospholipid complex (RRF-PC) in rat plasma.
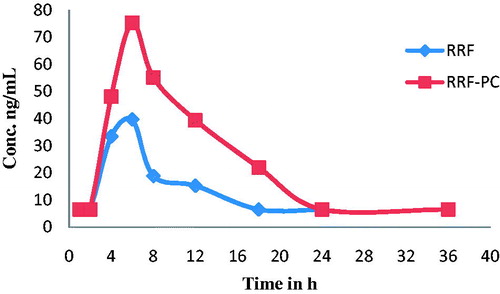
Table 3. Pharmacokinetic study of boeravinone B.
Discussion
The findings of the present work demonstrated an enhanced in vivo anti-inflammatory effect of lipid-based formulation (PC) of RRF, which was prepared from 70% ethanol extract of B. diffusa by fractionation over Diaion HP-20 resin column eluted with water–methanol (Bairwa, Citation2013a,Citationb). The PC was prepared by a simple reflux and evaporation of the solvent method. The formation of PC takes place by intermolecular hydrogen bonding or some other physicochemical interaction between the polar head group of phosphatidylcholine and the polar groups (hydroxyl or phenolic–OH) of phytoconstituents (Bhattacharya, Citation2009; Yanyu, Citation2006).
The PC was characterized by employing 1H and 31P NMR spectroscopies. Methyl protons of the +N(CH3)3 group in phosphatidylcholine are de-shielded due to high electronegativity of the nitrogen atom (+N) when compared with the carbon atom. While in PC of RRF, the quaternary nitrogen of the choline group shares its electronegativity with polar atoms of phytoconstituents to form the hydrogen bond or some other physicochemical interactions like van der Waals forces. Due to this sharing of electronegativity, the net electronegativity of nitrogen atom towards N-methyl atoms was reduced, which causes release of electrons towards methyl groups (shielding effect) and thus the up-field shift was observed for methyl protons of the +N(CH3)3 group in RRF-PC (δH 3.23 ppm) when compared with the chemical shift of this group in phosphatidylcholine (δH 3.37 ppm) ( and ). Similarly, an up-field shift (from δp −1.26 to δp −1.57 ppm) observed in the 31P NMR spectrum of RRF-PC, further confirmed that in RRF-PC, there is a formation of hydrogen bond with oxygen atoms that are directly attached with the phosphorus atom of phosphatidylcholine () (Bombardelli, Citation1991; Cheung, Citation1990; Gabetta, Citation1989). Formation of RRF-PC was further confirmed by FTIR. Disappearance of −(P = O) stretching vibration band at 1255 cm−1 in the IR spectrum of RRF-PC revealed that the phenolic or alcoholic OH group of phytoconstituents present in RRF may interact with the P = O group of phosphatidylcholine by the formation of new hydrogen bonds in RRF-PC (Hou, Citation2013). Apart from this, no new significant peaks were observed in the RRF-PC when compared with FTIR spectra of RRF and phosphatidylcholine, which confirms the formation of RRF-PC.
Phospholipids are the building blocks of biomembranes and have both hydrophilic and lipophilic moieties in their structure that causes significant increase in solubility of hydrophilic as well as lipophilic phytoconstituents. Further, the phospholipid complexes get passively absorbed through biomembrane resulting in increased oral bioavailability of respective phytoconstituents. The results from the pharmacokinetic study of boeravinone B demonstrated that in case of RRF-PC, its plasma concentration increases about two times when compared with that in RRF. This, in turn, enhanced in vivo anti-inflammatory activity of RRF-PC when compared with RRF and supports above findings (Khan et al., Citation2013; Mahady, Citation2001; Semalty et al., Citation2010).
Conclusion
Phospholipid formulation of RRF prepared from 70% ethanol extract of B. diffusa roots was developed and studied for anti-inflammatory activity in vivo. The enhanced anti-inflammatory activity exhibited by phospholipid formulation (RRF-PC) could be attributed to an increased oral bioavailability, observed in pharmacokinetic studies.
Declaration of interest
National Institute of Pharmaceutical Education and Research (NIPER) provided financial assistance and necessary facilities for carry out this work. The authors declare that the institute has no conflicts of interest.
References
- Adesina SK. (1979). Anticonvulsant properties of the roots of Boerhaavia diffusa Linnaeus. Pharm Biol 17:84–6
- Ahmed-Belkacem A, Macalou S, Borrelli F, et al. (2007). Nonprenylated rotenoids, a new class of potent breast cancer resistance protein inhibitors. J Med Chem 50:1933–8
- Ajazuddin, Saraf S. (2010). Applications of novel drug delivery system for herbal formulations. Fitoterapia 81:680–9
- Bairwa K, Singh IN, Roy SK, et al. (2013a). Rotenoids from Boerhaavia diffusa as potential anti-inflammatory agents. J Nat Prod 76:1393–8
- Bairwa K, Singh IN, Roy SK, et al. (2013b). Correction to rotenoids from Boerhaavia diffusa as potential anti-inflammatory agents. J Nat Prod 76:2364
- Bairwa K, Srivastava A, Jachak SM. (2014). Quantitative analysis of boeravinones in the roots of Boerhaavia diffusa by UPLC/PDA. Phytochem Anal 25:415--20
- Bhalla TN, Gupta MB, Bhargava KP. (1971). Anti-inflammatory activity of Boerhaavia diffusa. J Res Indian Med 6:11–15
- Bhattacharya S, Ghosh A. (2009). Phytosomes: The emerging technology for enhancement of bioavailability of botanicals and nutraceuticals. Int J Aesthetic Antiaging Med 2:87–91
- Bombardelli E, Spelta M, Della Loggia R, et al. (1991). Aging skin: Protective effect of silymarin phytosome. Fitoterapia 62:115–22
- Borrelli F, Ascione V, Capasso R, et al. (2006). Spasmolytic effects of nonprenylated rotenoid constituents of Boerhaavia diffusa roots. J Nat Prod 69:903–6
- Borrelli F, Milic N, Ascione V, et al. (2005). Isolation of new rotenoids from Boerhaavia diffusa and evaluation of their effect on intestinal motility. Planta Med 71:928–32
- Cheung AP, Olson LL. (1990). 1H NMR assay of phosphatidylcholine and phosphatidylethanolamine in AL721. J Pharm Biol Anal 8:729–34
- Dhingra D, Valecha R. (2014). Evidence for involvement of the monoaminergic system in antidepressant-like activity of an ethanol extract of Boerhaavia diffusa and its isolated constituent, punarnavine, in mice. Pharm Biol 52:767–74
- Ferreres F, Sousa C, Justin M, et al. (2005). Characterisation of the phenolic profile of Boerhaavia diffusa L. by HPLC-PAD-MS/MS as a tool for quality control. Phytochem Anal 16:451–8
- Gabetta B, Zini GF, Pifferi G. (1989). Spectroscopic studies on IdB 1016, a new flavolignan complex. Planta Med 55:615–17
- Hou Z1, Li Y, Huang Y, et al. (2013). Phytosomes loaded with mitomycin C-soybean phosphatidylcholine complex developed for drug delivery. Mol Pharm 10:99–101
- Jain GK, Khanna NM. (1989). Punarnavoside: A new antifibrinolytic agent from Boerhaavia diffusa Linn. Ind J Chem B 28:163–6
- Jain N, Gupta BP, Thakur N, et al. (2010). Phytosome: A novel drug delivery system for herbal medicine. Int J Pharm Sci Drug Res 2:224–8
- Kadota S, Lami N, Tezuka Y, Kikuchi T. (1989). Constituents of roots of Boerhaavia diffusa L. I. Examination of sterols and structure of new rotenoids, boeravinone A and B. Chem Pharm Bull 37:3214–20
- Kamboj VP. (2000). Herbal medicine. Curr Sci 78:35–9
- Khan J, Alexander A, Ajazuddin, et al. (2013). Recent advances and future prospects of phyto-phospholipid complexation technique for improving pharmacokinetic profile of plant actives. J Control Release 168:50–60
- Kirtikar KR, Basu BD. (1956). Indian Medicinal Plants. Allahabad, India: Lalit Mohan Basu, 2045–8
- Lami N, Kadota S, Kikuchi T, Momose Y. (1991b). Constituents of the roots of Boerhaavia diffusa L. III. Identification of Ca2+ channel antagonistic compound from the methanol extract. Chem Pharm Bull 39:1551–5
- Lami N, Kadota S, Kikuchi T. (1991a). Constituents of roots of Boerhaavia diffusa L. IV. Isolation and structure determination of boeravinone D, E, and F. Chem Pharm Bull 39:1863–5
- Lami N, Kadota S, Tezuka Y, Kikuchi T. (1990). Constituents of roots of Boerhaavia diffusa L. II. Structure and stereochemistry of a new rotenoid, boeravinone C. Chem Pharm Bull 38:1558–62
- Lu Y, Zhang Y, Yang Z, Tang X. (2009). Formulation of an intravenous emulsion loaded with a clarithromycin–phospholipid complex and its pharmacokinetics in rats. Int J Pharm 366:160–9
- Mahady GB. (2001). Global harmonization of herbal health claims. J Nutr 131:11205–35
- Mehrotra S, Mishra KP, Maurya R, et al. (2002). Immunomodulation by ethanolic extract of Boerhaavia diffusa roots. Int Immunopharmacol 2:987–96
- Misra AN, Tiwari HP. (1971). Constituents of roots of Boerhaavia diffusa. Phytochemistry 10:3318–19
- Pareta SK, Patra KC, Mazumder PM, Sasmal D. (2011). Aqueous extract of Boerhaavia diffusa root ameliorates ethylene glycol-induced hyperoxaluric oxidative stress and renal injury in rat kidney. Pharm Biol 49:1224–33
- Pari L, Amarnath Satheesh M. (2004). Antidiabetic activity of Boerhaavia diffusa L. effect on hepatic key enzymes in experimental diabetes. J Ethnopharmacol 91:109–13
- Rawat AKS, Mehrotra S, Tripathi SC, Shome U. (1997). Hepatoprotective activity of Boerhaavia diffusa L. roots a popular Indian ethnomedicine. J Ethnopharmacol 56:61–6
- Saraswati S, Alhaider AA, Agrawal SS. (2013). Punarnavine, an alkaloid from Boerhaavia diffusa exhibits anti-angiogenic activity via downregulation of VEGF in vitro and in vivo. Chem-Biol Interact 206:204–13
- Semalty A, Semalty M, Singh M, et al. (2010). Supramolecular phospholipids-polyphenolics interactions: The PHYTOSOME® strategy to improve the bioavailability of phytochemicals. Fitoterapia 81:306–14
- Shah VN, Shah MB, Bhatt PA. (2011). Hepatoprotective activity of punarnavashtak kwath, an Ayurvedic formulation, against CCl4-induced hepatotoxicity in rats and on the HepG2 cell line. Pharm Biol 49:408–15
- Sreeja S, Sreeja S. (2009). An in vitro study on antiproliferative and antiestrogenic effects of Boerhaavia diffusa L. extracts. J Ethnopharmacol 126:221–5
- Winter CA, Risley EA, Nuss GW. (1962). Carrageenin-induced edema in hind paw of the rat as an assay for antiinflammatory drugs. Proc Soc Exp Biol Med 3:544–7
- Yanyu X, Yunmei S, Zhipeng C, Qineng P. (2006). The preparation of silybin-phospholipid complex and the study on its pharmacokinetics in rats. Int J Pharm 307:77–82