Abstract
Context: Convolvulus pluricaulis Chois. (Convolvulaceae), a well-known Ayurvedic “Medhya Rasayana” (nervine tonic), is extensively used for different central nervous system (CNS) disorders.
Objective: The objective of this study was to evaluate the protective effect of standardized hydro-methanol extract of C. pluricaulis (CPE) and its fractions, namely ethyl acetate (EAE), butanol (BE), and aqueous (AE), against 3-nitropropionic acid (3-NP) induced neurotoxicity in rats.
Materials and methods: The extract of the whole plant was standardized on the basis of scopoletin content (0.014%) using thin layer chromatography densitometric analysis. CPE (100 and 200 mg/kg) and its fractions, namely EAE (15 and 30 mg/kg), BE (25 and 50 mg/kg), and AE (50 and 100 mg/kg) were administered orally for 20 d. Their protective effect against 3-NP (10 mg/kg, i.p. for 14 d) was assessed by the effect on various behavioral parameters, namely body weight, locomotor activity, grip strength, gait pattern, and the effect on cognitive dysfunction. Biochemical parameters for oxidative damage were also assessed in the striatum and cortex regions of the brain.
Results: Administration of 3-NP induced HD-like symptoms that were indicated by reduced body weight, locomotor activity, memory, grip strength, and oxidative defense. CPE (200 mg/kg), EAE (30 mg/kg), and BE (50 mg/kg) significantly (p < 0.001) attenuated 3-NP induced reduction in locomotor activity, grip strength, memory, body weight, and oxidative defense in comparison with 3-NP-treated animals on 10 and 15 d.
Conclusion: The present study suggested that CPE has a protective action against 3-NP-induced neurotoxicity and can be further explored for its efficacy against Huntington’s disease.
Introduction
Huntington’s disease (HD) is an autosomal dominant inherited progressive neurodegenerative disorder, characterized by the progressive development of involuntary choreiform movements, cognitive impairment, neuropsychiatric symptoms, and premature death (Kumar et al., Citation2009). The pathogenesis of HD is unknown, but various reports suggest the involvement of mitochondrial dysfunction, excitotoxicity, and oxidative damage in the development of this disorder (Fatokun et al., Citation2008; Kumar & Kumar, Citation2010; Kumar et al., Citation2011). Impairment in the mitochondrial electron transport chain results in intracellular oxidative stress leading to generation of free radicals that further cause macromolecular changes in vulnerable areas of brain, behavioral impairment, and memory deficits (Beal, Citation1998; Butterfield et al., Citation2001). 3-Nitropropionic acid (3-NP) is a fungal neurotoxin, irreversibly inhibits succinate dehydrogenase (SDH) enzyme in the tricarboxylic acid cycle (TCA) and electron transport chain (Ludolph et al., Citation1991). Systemic 3-NP administration produces striatum-specific lesions, delayed dystonia, and abnormal choreiform movements in both animals and human beings, symptoms similar to those in HD (Coles et al., Citation1979). ATP exhaustion by the 3-NP intoxications is also accompanied by oxidative damage in different brain areas, particularly in the striatum (Pang & Geddes, Citation1997). 3-NP-induced selective striatal damage is very much similar to that of HD (Kalonia et al., Citation2010; Kumar et al., Citation2007c). It is, therefore, considered as a good experimental model, which closely resembles some of its human pathological and behavioral aspects (Beal et al., Citation1993; Borlongan et al., Citation1997).
Convolvulus pluricaulis Chois. (Convolvulaceae), also known as Shankhpushpi, has been used traditionally for its memory enhancing, anticonvulsant, antianxiety, and sedative properties (Chunekar & Pandey, Citation2002). The plant is mentioned as one of the best Medhya Rasayana (nervine tonic) in Ayurveda. The plant contains alkaloid (Shankhapushpine), volatile oil, flavonoids (kaempferol derivatives), phytosterol (β-sitosterol), carbohydrates, 20-oxodotriacontanol, tetra-triacontanoicacid, 29-oxodotriacontanol and scopoletin (Bisht et al., Citation1978; Chowdhary & Kapoor, Citation1986; Deshpande & Srivastava, Citation1969). The plant has also well reported for its memory enhancing (Bihaqi et al., Citation2012; Malik et al., Citation2011; Nahata et al., Citation2008), neuroprotective (Bihaqi et al., Citation2009), antidepressant (Dhingra & Valecha, Citation2007), and antioxidant (Joshi et al., Citation2004) activities. Therefore, the present study was designed to explore the effect of CP against 3-NP-induced behavioral and oxidative stress alterations in striatum and cortex regions in rat brain.
Materials and methods
Plant material
Whole plant of C. pluricaulis (CP) was collected in May 2011 from Risalia Khera, Distt. Sirsa, Haryana, and authenticated by Dr. H. B. Singh, National Institute of Science Communication and Information Resources (NISCIR), New Delhi, vide certificate no. 2011/1540/138. The voucher specimen was kept at the departmental Herbarium, ISF College of Pharmacy, Moga.
Animals
A total of 80 male Wistar rats (250–300 g) 4–5-month old procured from Animal House of ISF College of Pharmacy, Moga, were used. Animals were acclimatized to laboratory conditions prior to experimentation. The animals were kept under standard conditions of 12 h artificial light/dark cycle with food and water ad libitum. Room temperature was maintained at 20–22 °C with a relative air humidity of approximately 50%. All experiments were carried out between 09:00 and 17:00 h. The experimental protocol was reviewed and approved by the Institutional Animal Ethics Committee (IAEC) vide certificate no. IAEC/M4/CPCSEA/P63/2012 dated 3 March 2012 and carried out in accordance with the Indian National Science Academy Guidelines (INSA) for the use and care of animals.
Extraction of plant material and its fractionation
The whole plant material was dried in shade and ground to a coarse powder. The powdered plant material (500 g) was defatted with petroleum ether (60–80 °C) using Soxhlet for 6 h. The marc was then air dried and extracted with 80% aqueous methanol using Soxhlet for 8 h. A portion of hydro-methanol extract was concentrated under vacuum and was subjected to lyophilization for 48 h. This lyophilized 80% aqueous methanol extract (CPE) was further used for animal testing. The remaining portion was suspended in distilled water (500 ml) and was sequentially partitioned with petroleum ether, ethyl acetate and n-butanol (5 × 500 ml each solvent). The partitioned fractions were made free from solvent using the rotary vacuum evaporator. The dried fractions were also lyophilized and were further used for animal testing.
Phytochemical screening of extract
The hydro-methanol extract of the plant was tested for the presence of alkaloids, flavonoids, sterols, tannins, triterpenes, anthraquinone glycosides, cardiac glycosides, cyanogenetic glycosides, and carbohydrates. The extract showed the presence of flavonoides, sterols, coumarins, and carbohydrates.
Standardization of hydro-methanol extract using TLC densitometry (CAMAG) on the basis of scopoletin content
Preparation of stock solution of standard scopoletin
Scopoletin (10 mg), from Sigma-Aldrich (St. Louis, MO), was weighed accurately and was dissolved in 10 ml of methanol to obtain a concentration of 1 mg/ml. The stock solution was further diluted with methanol to obtain the final concentration of 0.02 mg/ml.
Preparation of test solution
The shade dried coarsely powdered drug (5 g) was extracted with 50 ml 80% aqueous methanol for 8 h using Soxhlet. The extract was filtered through the Whatman fine paper, and the final volume was made up to 50 ml. The sample stock solution (10 µl) was applied on pre-coated aluminum based-TLC plate (Merck Pvt. Ltd., Darmstadt, Germany) followed by development and scanning. All the sample applications were done in triplicate.
A developed and validated HPTLC method (Kapadia et al., Citation2006) was used for the determination of scopoletin in the extract. A calibration curve was developed for scopoletin using the mobile phase toluene:ether (1:1; saturated with 20% acetic acid) with a saturation time of 10 min. The calibration curve was developed using a concentration range of 40–240 ng band−1 which showed a good correlation coefficient (r2 = 0.9990) with a regression equation (y = 215.6 + 20.77 x) showing a low intercept value.
Content of scopoletin in C. pluricaulis
A 10 µl band of both standard scopoletin solution and extract of C. pluricaulis was applied on the TLC plate, developed as per the conditions and procedures described previously (Kapadia et al., Citation2006). The area under curve (AUC) of the scopoletin peak in the extract was recorded and the scopoletin content was calculated using the regression equation derived from its calibration curve. The scopoletin content was found to be 0.014% w/w in the extract of C. pluricaulis.
Drugs and treatment schedule
3-NP (Sigma Chemicals, St. Louis, MO) was diluted with saline (adjust pH 7.4) and administered at a dose of 10 mg/kg, i.p. to animals. CPE (100 and 200 mg/kg), ethyl acetate (EAE): 15 and 30 mg/kg; n-butanol (BE): 25 and 50 mg/kg and remaining aqueous (AE): 50 and 100 mg/kg, fractions were suspended in 0.5% w/v sodium carboxy-methyl-cellulose (CMC) solution and administered by oral route in a constant volume of 0.5 ml/100 g of body weight. Animals were randomly divided into 10 groups with eight animals in each group. The doses of fractions were calculated on the basis of their respective yield (%w/w). CPE and various fractions at different dose levels were administered orally for 20 d, starting 6 d prior to first 3-NP injection (10 mg/kg, i.p.), and continued along with 3-NP treatment for the next 14 d. CPE and various fractions namely EAE, BE, and AE, at respective dose levels were administered 1 h prior to 3-NP administration. The dosing regimen was selected on the basis of previous studies (Kumar et al., Citation2006a, Citation2007b).
Group 1 was the vehicle-treated group; Group 2 animals received 3-NP (10 mg/kg) for 14 d, Groups 3 and 4 received CPE (100 and 200 mg/kg) + 3-NP (10 mg/kg); Groups 5 and 6 received EAE (15 and 30 mg/kg) + 3-NP (10 mg/kg); Groups 7 and 8 received BE (25 and 50 mg/kg) + 3-NP (10 mg/kg); Groups 9 and 10 received AE (50 and 100 mg/kg) + 3-NP (10 mg/kg), respectively.
Measurement of body weight
Body weight was recorded on the first and last day of the experiment and the change in body weight (%) was calculated.
Assessment of locomotor activity
The locomotor activity was monitored using the actophotometer (IMCORP, Ambala, India). The motor activity in actophotometer was detected by infrared photocells installed in the instrument. Animals were placed individually in the activity chamber for 3 min as a habituation period before making actual recording. Each animal was observed over a period of 10 min and expressed as counts per 10 min (Kumar & Kumar, Citation2010).
Rotarod activity
Motor incoordination and grip strength were assessed using the rotarod apparatus (Techno, Ambala, India). Animals were exposed to prior training session to acclimatize them on rotarod before starting the actual assessing of drug treatment. Animals were placed on the rotating rod with a diameter of 7 cm (speed 25 rpm). The cut-off time was 300 s. Three separate trials after 5 min gap were given to each rat. The average fall off time was recorded (Kumar & Kumar, Citation2010).
Beam-crossing task
This task requires an animal to walk on across a narrow wooden beam, measuring its motor coordination ability and gait pattern. The beam consisted of two platforms (8 cm in diameter) connected by a wooden beam (1.0 cm in width and 100 cm in length). The beam was elevated 100 cm above ground. A box filled with sawdust was placed below the beam, serving as a protection for a falling rat. All rats must be trained to walk over a beam for 5 d before testing. A ceiling of 120 s is employed at the end of which the rat is removed and placed in the cage. Time taken to traverse rat from start platform to their home cage was measured along with their number of slipping errors (Kalonia et al., Citation2011).
Morris water maze test
The acquisition and the retention of a spatial navigation task were examined by performance in Morris water maze test (MWM). The animals were trained to swim to a platform in a circular pool (180 cm diameter × 60 cm height) located in a test room. The pool was filled with water (28 ± 2 °C) to a depth of 40 cm and water was made opaque by adding a non-toxic water dispersible emulsion to prevent the visibility of the platform. A movable circular platform, 9 cm in diameter, mounted on a column, was placed in the pool 1 cm below the water level for the maze acquisition test. The tank was located in a large room where there were several brightly colored cues external to the maze; these were visible from the pool and could be used by the rats for spatial orientation. The position of the cues remained unchanged throughout the study. The platform was fixed in the centre of one of the four quadrants and remained in that location for the duration of the experiment. The rats were exposed to four consecutive daily training trials starting from the first day of 3-NP administration, with each trial having a ceiling time of 120 s, and a trial interval of approximately 5 min. For each trial, each rat was put into the water at one of the four starting positions, the sequence of which being selected randomly. During the test trials, the rats were placed in the tank at the same starting point, with their heads facing the wall. The rat was made to swim before climbing onto the platform. After climbing onto the platform, the animal remained there for 20 s, before the commencement of the next trial. The escape platform was kept in the same position relative to the distal cues. If the rat failed to reach the escape platform within the maximally allowed time of 90 s, it was gently placed on the platform and allowed to remain there for the same amount of time. The time to reach the platform (transfer latency in seconds) was measured on days 5, 10, and 15. On day 16, the platform was removed and the animal was randomly released at any one of the edges (N, S, E, and W) facing the wall of the pool and tested for the retention of the memory. The time spent in the target quadrant (where the platform was placed) was recorded (Kumar & Kumar, Citation2009a; Malik et al., Citation2013). The higher value of the time spent in quadrant indicates better retention ability as the animal remembered the position of the platform.
Dissection and homogenization
After behavioral assessments, animals were killed by decapitation immediately for biochemical analysis. The brains were removed, and cortex and striatum were separated by putting on ice. A 10% (w/v) tissue homogenate was prepared in 0.1 M phosphate buffer (pH 7.4). The homogenate was centrifuged at 10 000 g for 15 min. Aliquots of the supernatant were separated and used for biochemical estimations.
Measurement of lipid peroxidation
The quantitative measurement of lipid peroxidation was performed according to the method of Wills (Citation1966). The amount of malondialdehyde (MDA), a measure of lipid peroxidation, was measured by reacting with thiobarbituric acid at 532 nm using the UV-1700 Spectrophotometer, Shimadzu, Japan. The values were calculated using the molar extinction coefficient of chromophore (1.56 × 105 M−1 cm−1) and expressed as nmole of malondialdehyde per milligram of protein.
Estimation of nitrite
The accumulation of nitrite in the supernatant, an indicator of the production of nitric oxide (NO), was determined with a colorimetric assay with the Greiss reagent [0.1% N-(1-naphthyl) ethylenediamedihydrochloride, 1% sulfanilamide, and 2.5% phosphoric acid] (Green et al., Citation1982). Equal volumes of supernatant and Greiss reagent were mixed; the mixture was incubated for 10 min at room temperature in the dark, and the absorbance at 540 nm was determined with UV-1700 Spectrophotometer, Shimadzu, Japan. The concentration of nitrite in the supernatant was determined from a sodium nitrite standard curve and expressed as micromole per milligram of protein.
Catalase estimation
Catalase activity was assayed by the method of Luck (Citation1971), wherein the breakdown of hydrogen peroxides (H2O2) is measured at 240 nm. Briefly, assay mixture consisted of 3 ml of H2O2 phosphate buffer and 0.05 ml of supernatant of tissue homogenate (10%), and a change in the absorbance was recorded at 240 nm. The results were expressed as micromole H2O2 decomposed per milligram of protein per minute.
Superoxide dismutase activity
Superoxide dismutase (SOD) activity was assayed according to the method of Kono (Citation1978), wherein the reduction of nitrazo-bluetetrazolium (NBT), inhibited by the superoxide dismutase, is measured at 560 nm using a spectrophotometer. Briefly, the reaction was initiated by the addition of the hydroxylamine hydrochloride to the mixture containing NBT and sample. The results were expressed as unit per milligram protein, where one unit of enzyme is defined as the amount of enzyme inhibiting the rate of reaction by 100%.
Estimation of glutathione levels
Reduced glutathione (GSH) was measured (Ellman’s method) in the striatum and cortex of the rat brain (Ellman, Citation1959). Results were calculated using the molar extinction coefficient of chromophore (1.36 × 104 M−1 cm−1) and expressed as micromoles of GSH per milligram protein.
Protein estimation
The protein content was measured by the biuret method using bovine serum albumin as a standard (Gornall et al., Citation1949).
Statistical analysis
The data were analyzed using two-way analysis of variance (ANOVA) followed by the Bonferroni test for behavioral analysis and one-way ANOVA followed by Tukey’s test for biochemical analysis. All the values are expressed as mean ± SD. In all the tests, p < 0.05 was the criterion for statistical significance.
Results
Extraction of plant material and its fractionation
The hydro-methanol extraction of plant material (500 g) yields 75 g (15% w/w) of the extract out of which 25 g was lyophilized to get 14 g of the lyophilized hydro-methanol extract of C. pluricaulis (CPE) that was kept in a desiccator for biological activity. The remaining portion (50 g) was suspended in distil water (500 ml) and was sequentially portioned with ethyl acetate (EAE) and n-butanol (BE) to get 21 and 23% w/w of their respective soluble fractions. The remaining was the aqueous soluble fraction (AE).
Effect of CPE and its various fractions on body weight in the 3-NP-treated rats
3-NP (10 mg/kg, i.p.) treatment caused significant decrease in the body weight on the 15th day as compared with the vehicle-treated group (). Further, pretreatment with CPE, EAE, and BE significantly attenuated the loss in the body weight on the 15th day as compared with the 3-NP-treated group (). CPE and EAE (200 and 30 mg/kg, respectively) exhibited maximum protection (p < 0.001), followed by EAE and BE (15 and 50 mg/kg, p < 0.01), against 3-NP-induced decrease in the body weight. AE only at 100 mg/kg, p.o. showed improvement in the body weight on the 15th day as compared with the 3-NP treated group.
Figure 1. Effect of hydromethanol extract of C. pluricaulis and its fractions on the body weight of 3-NP-treated rats. Results are expressed as mean (%) change in body weight ± SD (n = 8); #p < 0.05 versus control; *p < 0.05, **p < 0.01, ***p < 0.001 versus 3-NP-treated rats. Results are compared using one-way analysis of variance followed by Tukey’s post hoc test. CPE, EAE, BE, and AE: hydromethanol, ethyl acetate, butanol, and remaining aqueous extract of C. pluricaulis, respectively.
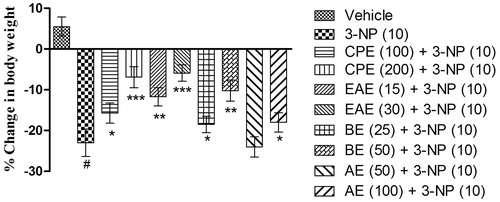
Effect of CPE and its various fractions on locomotor activity in the 3-NP-treated rats
A significant decrease in the locomotor activity was observed on the 5th, 10th, and 15th day in 3-NP-treated animals in comparison with vehicle-treated animals (). Further, CPE (100 and 200 mg/kg, p.o.) pretreatment significantly improved the locomotor activity (). Among various fractions of CPE, EAE and BE showed significant restoration of locomotor activity in comparison with 3-NP-treated animals. CPE (200 mg/kg), EAE (30 mg/kg), and BE (50 mg/kg) exhibited maximum significant (p < 0.001) restoration of the locomotor activity in comparison with the 3-NP-treated group. Further, AE (100 mg/kg, p.o.) pretreatment significantly restored the locomotor activity in 3-NP-treated animals only on the 15th day. However, lower dose of AE (50 mg/kg, p.o.) did not produce any significant (p > 0.05) effect in locomotor activity when compared with the 3-NP-treated group ().
Figure 2. Effect of hydromethanol extract of C. pluricaulis and its fractions on the locomotor activity of 3-NP-treated rats. Results are expressed as mean total count ± SD (n = 8); #p < 0.05 versus control; *p < 0.05, **p < 0.01, ***p < 0.001 versus 3-NP-treated rats. Results are compared using two-way analysis of variance followed by Bonferoni’s post hoc test. CPE, EAE, BE, and AE: hydromethanol, ethyl acetate, butanol, and remaining aqueous extract of C. pluricaulis, respectively.
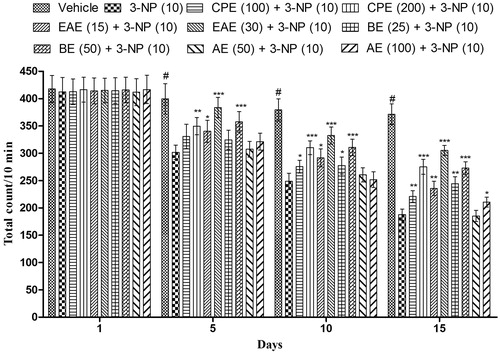
Effect of CPE and its various fractions on rotarod activity in 3-NP-treated rats
Results of clearly indicate that 3-NP treatment significantly impaired grip strength of the animals when tested on the rotarod test in comparison with animals treated with vehicle. However, CPE (200 mg/kg, p.o.) showed significant improvement in the rotarod activity on days 5, 10, and 15 as compared with the 3-NP-treated group. Further, CPE (100 mg/kg, p.o.) pretreatment significantly (p < 0.05) improved the rotarod activity in the 3-NP-treated group only on the 10th and the 15th day ().
Figure 3. Effect of hydromethanol extract of C. pluricaulis and its fractions on the rotarod activity of 3-NP-treated rats. Results are expressed as mean total time of fall ± SD (n = 8); #p < 0.05 versus control; *p < 0.05, **p < 0.01, ***p < 0.001 versus 3-NP-treated rats. Results are compared using two-way analysis of variance followed by Bonferoni’s post hoc test. CPE, EAE, BE, and AE: hydromethanol, ethyl acetate, butanol, and remaining aqueous extract of C. pluricaulis, respectively.
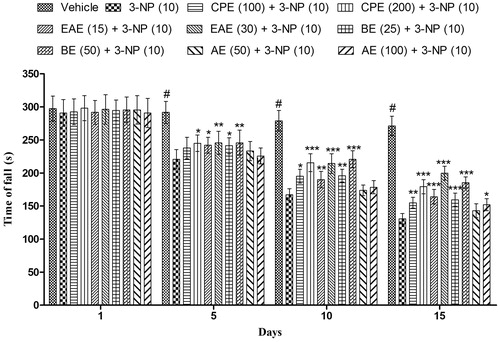
EAE and BE (15 and 25 mg/kg, respectively) exhibited significant p < 0.01 and 0.001 increase in the rotarod activity on days 10 and 15, respectively, in comparison with 3-NP-treated group. EAE and BE (30 and 50 mg/kg, respectively) showed maximum (p < 0.001) improvement in the 3-NP induced decreased rotarod activity of rats ().
Effect of CPE and its various fractions on narrow beam walk in 3-NP-treated rats
Systemic administration of 3-NP significantly increases the time taken by rats to traverse from start platform to their home cage as compared with the vehicle-treated group on the 5th, 10th, and 15th day (). Pretreatment with CPE and its fractions decreases the time taken by rats to cross the narrow beam and the number of slips as compared with the 3-NP-treated group (). Among various fractions tested, EAE (30 mg/kg) exhibited a significant (p < 0.001) and maximum decrease in the time taken by rats to cross the narrow beam as well as decreases the number of slips as compared with the 3-NP-treated groups on 5th, 10th, and 15th day ().
Figure 4. Effect of hydromethanol extract of C. pluricaulis and its fractions on the narrow beam walk activity of 3-NP-treated rats. (A) Time taken to cross the beam; (B) the number of slips. Results are expressed as mean ± SD (n = 8); #p < 0.05 versus control; *p < 0.05, **p < 0.01, ***p < 0.001 versus 3-NP-treated rats. Results of the time taken to cross the beam were compared using two-way analysis of variance followed by Bonferoni’s post hoc test; results of the number of slips were compared using one-way of analysis of variance followed by Tukey’s test. CPE, EAE, BE, and AE: hydromethanol, ethyl acetate, butanol, and remaining aqueous extract of C. pluricaulis, respectively.
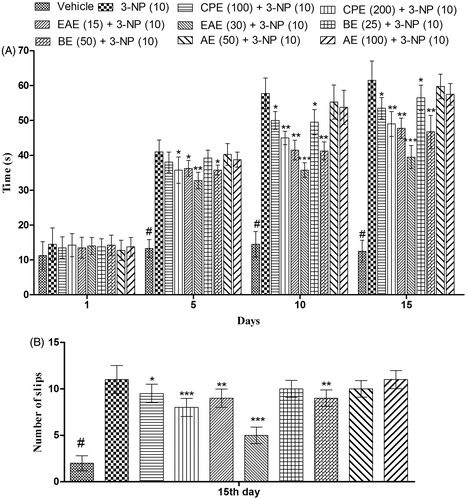
Effect of CPE and its various fractions on memory in MWM in 3-NP-treated rats
In the MWMT, mean escape latency of the vehicle-treated group gradually shortened during the training session from day 1st to 5th in comparison with the rats of the 3-NP group clearly indicating the impairment of learning process in 3-NP-treated groups. Moreover, significant (p < 0.05) increase in the mean transfer latency (day 10th and 15th) of the 3-NP-treated rats in comparison with the vehicle-treated group corroborate the memory impairment effect of 3-NP (). CPE (100 and 200 mg/kg, p.o.) treatment significantly improved memory performance that was indicated by shortened mean transfer latency on day 10th and 15th (). 3-NP treatment also significantly shortened the time spent in the target quadrant on the 15th day as compared with the vehicle-treated group (). However, the animals pretreated with CPE (100 and 200 mg/kg, p.o.) spent more time in the target quadrant as compared with 3-NP-treated animals ().
Figure 5. Effect of hydromethanol extract of C. pluricaulis and its fractions on memory in the Morris water maze test. (A) Time taken by rats to reach platform (transfer latency); (B) time spent in the target quadrant. Results are expressed as mean ± SD (n = 8); #p < 0.05 versus control; *p < 0.05, **p < 0.01, ***p < 0.001 versus 3-NP-treated rats. Results of the transfer latency were compared using two-way analysis of variance followed by Bonferoni’s post hoc test; results of the time spent in the target quadrant were compared using one-way of analysis of variance followed by Tukey’s test. CPE, EAE, BE, and AE: hydromethanol, ethyl acetate, butanol, and remaining aqueous extract of C. pluricaulis, respectively.
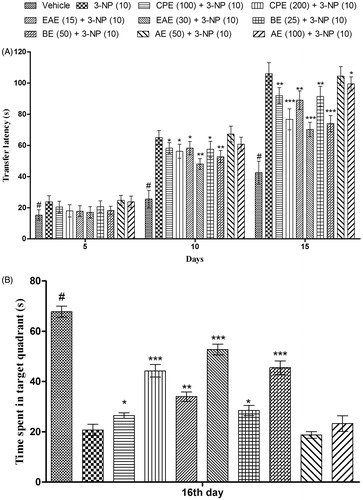
Among various fractions of CPE, EAE (15 and 30 mg/kg, p.o.) and BE (25 and 50 mg/kg, p.o.) treatments significantly improved memory performance on day 10th and 15th (shortened mean transfer latency) and increased the time spent in the target quadrant on day 16th, respectively, in comparison with 3-NP-treated rats (). EAE (30 mg/kg) and BE (50 mg/kg) exhibited maximum (p < 0.001) protection against 3-NP-induced memory impairment.
Effect of CPE and its various fractions on MDA, nitrite, catalase, SOD, and GSH levels in striatum and cortex in the brain of 3-NP-treated rats
Systemic administration of 3-NP significantly increased MDA and nitrite levels in the striatum and cortex of the animals as compared with the animals of the vehicle-treated group (). There was also a decrease in the levels of catalase, SOD, and reduced GSH in comparison with the vehicle-treated group (). Pretreatment with CPE (100 and 200 mg/kg, p.o.) significantly attenuated the increase in MDA and nitrite level, and also reversed the decrease in the levels of catalase, SOD, and GSH in the striatum and cortex in comparison with the 3-NP-treated groups. EAE and BE at their respective dose levels significantly attenuated the alterations of various biochemical parameters. As observed in the results of behavioral parameters, EAE (30 mg/kg) exhibited maximum protection against 3-NP-induced oxidative damage.
Figure 6. Effect of hydromethanol extract of C. pluricaulis and its fractions on various biochemical parameters. (A) MDA levels, (B) nitrite levels, (C) catalase levels, (D) SOD levels, and (E) reduced GSH levels. Results are expressed as mean ± SD (n = 8); #p < 0.05 versus control; *p < 0.05, **p < 0.01, ***p < 0.001 versus 3-NP-treated rats. Results were compared using one-way of analysis of variance followed by Tukey’s test. CPE, EAE, BE, and AE: hydromethanol, ethyl acetate, butanol, and remaining aqueous extract of C. pluricaulis, respectively.
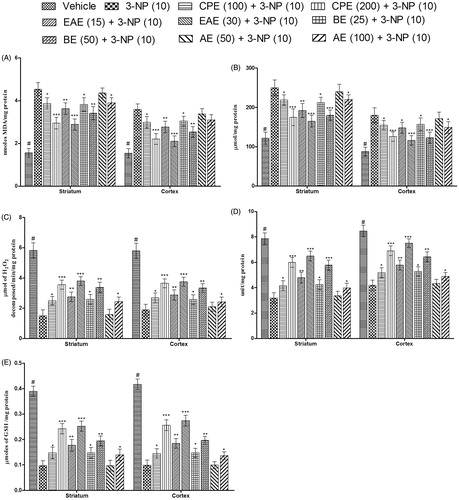
Discussion
The present study highlights the therapeutic potential of CPE and its fractions namely EAE, BE, and AE, against 3-NP-induced behavioral alterations and oxidative damage. Among various fractions tested, EAE (30 mg/kg) was found to be the most effective in reversing 3-NP-induced behavioral alterations (improved body weight, locomotor, rotarod performance, balance beam walk performance, and memory retention) and oxidative damage (attenuated lipid peroxidation, nitrite levels, restored catalase, SOD, and GSH levels).
Systemic 3-NP treatment for 14 d significantly caused body weight loss and motor abnormalities (reduced locomotor, grip strength performance, increases time in beam walk performance, and the number of slips), suggesting behavioral changes. Supporting to the present investigation, late-stage HD patients also exhibit dysphagia and loss of body weight (Saydoff et al., Citation2003). 3-NP-induced loss in the body weight could be partially due to factors outside the CNS, whereas alterations in locomotor and motor behavior could be its specific action on striatum (basal ganglia) that controls body movement (Saydoff et al., Citation2003). Further, the abnormal behavioral symptoms in early HD patients are primarily due to the dysfunction of cholinergic interneurons in striatal circuits or cell loss within the lateral striatum, ventral pallidum, and entopedoncular nucleus (Picconi et al., Citation2006). It seems that 3-NP produces alteration in motor behavioral by influencing striatum. However, the possibility of involvement of other brain areas such as cortex and hippocampus cannot be ignored in 3-NP action (Silva et al., Citation2007). Intriguingly, systemic treatments with CPE and its fractions for 14 d significantly restored the loss in the body weight in 3-NP-treated rats. Previous laboratory reports also reconfirm that substances possessing antioxidant property significantly restored the behavioral changes and oxidative defense level in 3-NP-treated animals (Kumar & Kumar, Citation2009c; Kumar et al., Citation2006a, Citation2007a; Rodríguez-Martínez et al., Citation2004). Pretreatment with CPE and its fractions also significantly attenuated other behavioral alterations (locomotor, rotarod, as well as beam walk performance) following 3-NP administration.
Systemic 3-NP administration also causes cognitive dysfunction that has not been understood clearly so far (Duckworth et al., Citation1999; Shear et al., Citation2000). 3-NP treatment significantly caused cognitive dysfunction as demonstrated by the results of MWMT (delayed transfer latency and decreased time spent in the target quadrant). These findings are in agreement with earlier reports suggesting a variety of neurobehavioral abnormalities and cognitive deficits after 3-NP administration (Kumar & Kumar, Citation2009b; Palfi et al., Citation1996). It has also been reported that 3-NP produces lesion in hippocampal CA1 and CA3 pyramidal neurons, areas of the brain associated with cognitive task (Sugino et al., Citation1999). Treatment with CPE and its various fractions significantly attenuated memory dysfunction against 3-NP treatment in animals. CPE (200 mg/kg) and EAE (30 mg/kg) treatment most significantly decreased time latency and increased the time spent in the target quadrant in the MWMR, suggesting its therapeutic potential against 3-NP-induced cognitive dysfunction. Reports have shown that dietary feeding of C. pluricaulis (CP) increases protein synthesis of the hippocampus, thus enhancing memory and learning in experimental animals (Sinha et al., Citation1989). It has also been found to augment memory function in many behavioral studies (Bihaqi et al., Citation2011; Malik et al., Citation2011; Sharma et al., Citation2010). Methanolic extract of plant also showed acetylcholinesterase inhibitory activity (Vinutha et al., Citation2007). The results of the present study also strengthened the claim of CP as a potential memory enhancer.
Previous studies have shown that 3-NP significantly induced oxidative damage and impaired antioxidant defense enzymes in the brain (Kumar & Kumar, Citation2009d; Perez-De La Cruz et al., Citation2009; Tunez & Santamaria, Citation2009). Antioxidant treatment strategies showed a significant neuroprotective effects against 3-NP neurotoxicity (Kumar et al., Citation2006b, Citation2007b). In the present study, 3-NP significantly induced oxidative damage (increased lipid peroxidation, nitrite concentration, and depleted catalase, SOD and reduced glutathione levels) in the striatum, and cortex regions. These changes were significantly restored by pretreatment with CPE and its fractions. Furthermore, among various fractions of CPE tested, EAE showed maximum protection against 3-NP-induced oxidative damage.
In summary, the present study suggests the protective effect of C. pluricaulis against 3-NP-induced neurotoxicity could be due to its strong antioxidant effect. However, more investigations are required to elucidate the cellular mechanisms of CP against 3-NP-induced Huntington’s disease-like symptoms.
Acknowledgements
The research facilities provided by Mr. Praveen Garg (Chairman), ISF College of Pharmacy, Moga, Punjab (India), are gratefully acknowledged.
Declaration of interest
The authors report that there are no declaration of interest.
References
- Beal MF, Brouillet E, Jenkins BG, et al. (1993). Neurochemical and histologic characterization of striatal excitotoxic lesions produced by the mitochondrial toxin 3-nitropropionic acid. J Neurosci 13:4181–92
- Beal MF. (1998). Mitochondrial dysfunction in neurodegenerative diseases. Biochim Biophys Acta 1366:211–23
- Bihaqi SW, Sharma M, Singh AP, Tiwari M. (2009). Neuroprotective role of Convolvulus pluricaulis on aluminium induced neurotoxicity in rat brain. J Ethnopharmacol 124:409–15
- Bihaqi SW, Singh AP, Tiwari M. (2011). In vivo investigation of the neuroprotective property of Convolvulus pluricaulis in scopolamine-induced cognitive impairments in Wistar rats. Indian J Pharmacol 43:520–5
- Bihaqi SW, Singh AP, Tiwari M. (2012). Supplementation of Convolvulus pluricaulis attenuates scopolamine-induced increased tau and amyloid precursor protein (AbetaPP) expression in rat brain. Indian J Pharmacol 44:593–8
- Bisht NPS, Singh R, Bansal RK, Singh P. (1978). Isolation of n-triacontane, aliphatic alcohols and sitosterols from Convolvulus microphyllus Sieb. Organische Chemie 33B:249
- Borlongan CV, Koutouzis TK, Sanberg PR. (1997). 3-Nitropropionic acid animal model and Huntington's disease. Neurosci Biobehav Rev 21:289–93
- Butterfield DA, Howard BJ, LaFontaine MA. (2001). Brain oxidative stress in animal models of accelerated aging and the age-related neurodegenerative disorders, Alzheimer's disease and Huntington's disease. Curr Med Chem 8:815–28
- Chowdhary MR, Kapoor RC. (1986). Chemical constituents of Convolvulus microphyllus. Curr Sci 55:917–18
- Chunekar KC, Pandey GS. (2002). Bhavaprakasa Nighantu – Indian Materia Medica of Sri Bhavamisra. Varanasi: Chaukhambha Bharati Academy
- Coles CJ, Edmondson DE, Singer TP. (1979). Inactivation of succinate dehydrogenase by 3-nitropropionate. J Biol Chem 254:5161–7
- Deshpande SM, Srivastava DN. (1969). Chemical studies of Convolvulus puricaulis. I. J Indian Chem Soc 46:759–60
- Dhingra D, Valecha R. (2007). Evaluation of the antidepressant-like activity of Convolvulus pluricaulis Choisy in the mouse forced swim and tail suspension tests. Med Sci Monit 13:BR155–61
- Duckworth EA, Koutouzis TK, Borlongan CV, et al. (1999). Rats receiving systemic 3-nitropropionic acid demonstrate impairment of memory in Morris water maze. Psychobiology 27:561–6
- Ellman GL. (1959). Tissue sulfhydryl groups. Arch Biochem Biophys 82:70–7
- Fatokun AA, Smith RA, Stone TW. (2008). Resistance to kynurenic acid of the NMDA receptor-dependent toxicity of 3-nitropropionic acid and cyanide in cerebellar granule neurons. Brain Res 1215:200–7
- Gornall AG, Bardawill CJ, David MM. (1949). Determination of serum proteins by means of the biuret reaction. J Biol Chem 177:751–66
- Green LC, Wagner DA, Glogowski J, et al. (1982). Analysis of nitrate, nitrite, and [15N] nitrate in biological fluids. Anal Biochem 126:131–8
- Joshi JP, Kamat JP, Mohan H, et al. (2004). Antioxidant effects of Convolvulus pluricalus in rat brain mitochondria against oxidative damage induced by gamma radiation and photosensitization. BARC Newslett 249:183–7
- Kalonia H, Kumar P, Kumar A. (2010). Targeting oxidative stress attenuates malonic acid induced Huntington like behavioral and mitochondrial alterations in rats. Eur J Pharmacol 634:46–52
- Kalonia H, Kumar P, Kumar A. (2011). Licofelone attenuates quinolinic acid induced Huntington like symptoms: Possible behavioral, biochemical and cellular alterations. Prog Neuropsychopharmacol Biol Psychiatry 35:607–15
- Kapadia NS, Acharya NS, Acharya SA, Shah MB. (2006). Use of HPTLC to establish a distinct chemical profile for Shankhpushpi and for quantification of scopoletin in Convolvulus pluricaulis Choisy and in commercial formulations of Shankhpushpi. JPC 19:195–9
- Kono Y. (1978). Generation of superoxide radical during autoxidation of hydroxylamine and an assay for superoxide dismutase. Arch Biochem Biophys 186:189–95
- Kumar P, Kalonia H, Kumar A. (2009). Sesamol attenuate 3-nitropropionic acid-induced Huntington-like behavioral, biochemical, and cellular alterations in rats. J Asian Nat Prod Res 11:439–50
- Kumar P, Kalonia H, Kumar A. (2011). Role of LOX/COX pathways in 3-nitropropionic acid-induced Huntington's disease-like symptoms in rats: Protective effect of licofelone. Br J Pharmacol 164:644–54
- Kumar P, Kumar A. (2009a). Effect of lycopene and epigallocatechin-3-gallate against 3-nitropropionic acid induced cognitive dysfunction and glutathione depletion in rat: A novel nitric oxide mechanism. Food Chem Toxicol 47:2522–30
- Kumar P, Kumar A. (2009b). Neuroprotective effect of cyclosporine and FK506 against 3-nitropropionic acid induced cognitive dysfunction and glutathione redox in rat: Possible role of nitric oxide. Neurosci Res 63:302–14
- Kumar P, Kumar A. (2009c). Possible role of sertraline against 3-nitropropionic acid induced behavioral, oxidative stress and mitochondrial dysfunctions in rat brain. Prog Neuropsychopharmacol Biol Psychiatry 33:100–8
- Kumar P, Kumar A. (2009d). Protective effect of rivastigmine against 3-nitropropionic acid-induced Huntington's disease like symptoms: Possible behavioural, biochemical and cellular alterations. Eur J Pharmacol 615:91–101
- Kumar P, Kumar A. (2010). Protective effect of hesperidin and naringin against 3-nitropropionic acid induced Huntington’s like symptoms in rats: Possible role of nitric oxide. Behav Brain Res 206:38–46
- Kumar P, Padi SS, Naidu PS, Kumar A. (2006a). Effect of resveratrol on 3-nitropropionic acid-induced biochemical and behavioural changes: Possible neuroprotective mechanisms. Behav Pharmacol 17:485–92
- Kumar P, Padi SS, Naidu PS, Kumar A. (2007a). Cyclooxygenase inhibition attenuates 3-nitropropionic acid-induced neurotoxicity in rats: Possible antioxidant mechanisms. Fundam Clin Pharmacol 21:297–306
- Kumar P, Padi SS, Naidu PS, Kumar A. (2007b). Possible neuroprotective mechanisms of curcumin in attenuating 3-nitropropionic acid-induced neurotoxicity. Methods Find Exp Clin Pharmacol 29:19–25
- Kumar P, Padi SSV, Naidu PS, Kumar A. (2006b). Protective effect of antioxidants on 3-nitropropionic acid induced oxidative stress and cognitive impairment. Ann Neurosci 13:41–7
- Kumar P, Padi SSV, Naidu PS, Kumar A. (2007c). 3-Nitropropionic acid induced neurotoxicity: An animal model for Huntington's disease. J Cell Tissue Res 7:853–60
- Luck H. (1971). Catalase. In: Bergmeyer HU, ed. Methods of Enzymatic Analysis. New York: Academic Press, 885–93
- Ludolph AC, He F, Spencer PS, et al. (1991). 3-Nitropropionic acid-exogenous animal neurotoxin and possible human striatal toxin. Can J Neurol Sci 18:492–8
- Malik J, Karan M, Vasisht K. (2011). Nootropic, anxiolytic and CNS-depressant studies on different plant sources of shankhpushpi. Pharm Biol 49:1234–42
- Malik J, Kumar M, Deshmukh R, Kumar P. (2013). Ameliorating effect of lyophilized extract of Butea frondosa leaves on scopolamine-induced amnesia in rats. Pharm Biol 51:233–9
- Nahata A, Patil UK, Dixit VK. (2008). Effect of Convolvulus pluricaulis Chois. on learning behaviour and memory enhancement activity in rodents. Nat Prod Res 22:1472–82
- Palfi S, Ferrante RJ, Brouillet E, et al. (1996). Chronic 3-nitropropionic acid treatment in baboons replicates the cognitive and motor deficits of Huntington's disease. J Neurosci 16:3019–25
- Pang Z, Geddes JW. (1997). Mechanisms of cell death induced by the mitochondrial toxin 3-nitropropionic acid: Acute excitotoxic necrosis and delayed apoptosis. J Neurosci 17:3064–73
- Perez-De La Cruz V, Elinos-Calderon D, Robledo-Arratia Y, et al. (2009). Targeting oxidative/nitrergic stress ameliorates motor impairment, and attenuates synaptic mitochondrial dysfunction and lipid peroxidation in two models of Huntington's disease. Behav Brain Res 199:210–17
- Picconi B, Passino E, Sgobio C, et al. (2006). Plastic and behavioral abnormalities in experimental Huntington's disease: A crucial role for cholinergic interneurons. Neurobiol Dis 22:143–52
- Rodríguez-Martínez E, Rugerio-Vargas C, Rodríguez AI, et al. (2004). Antioxidant effects of taurine, vitamin C, and vitamin E on oxidative damage in hippocampus caused by the administration of 3-nitropropionic acid in rats. Int J Neurosci 114:1133–45
- Saydoff JA, Liu LS, Garcia RA, et al. (2003). Oral uridine pro-drug PN401 decreases neurodegeneration, behavioral impairment, weight loss and mortality in the 3-nitropropionic acid mitochondrial toxin model of Huntington's disease. Brain Res 994:44–54
- Sharma K, Bhatnagar M, Kulkarni SK. (2010). Effect of Convolvulus pluricaulis Choisy and Asparagus racemosus Willd on learning and memory in young and old mice: A comparative evaluation. Indian J Exp Biol 48:479–85
- Shear DA, Haik KL, Dunbar GL. (2000). Creatine reduces 3-nitropropionic-acid-induced cognitive and motor abnormalities in rats. Neuroreport 11:1833–7
- Silva RH, Abilio VC, Kameda SR, et al. (2007). Effects of 3-nitropropionic acid administration on memory and hippocampal lipid peroxidation in sleep-deprived mice. Prog Neuropsychopharmacol Biol Psychiatry 31:65–70
- Sinha SN, Dixit VP, Madnawat AVS, Sharma OP. (1989). The possible potentiation of cognitive processing on administration of Convolvulus microphyllus in rats. Indian Med 1:1–6
- Sugino T, Nozaki K, Takagi Y, et al. (1999). Expression and distribution of redox regulatory protein, thioredoxin after metabolic impairment by 3-nitropropionic acid in rat brain. Neurosci Lett 275:145–8
- Tunez I, Santamaria A. (2009). Model of Huntington's disease induced with 3-nitropropionic acid. Rev Neurol 48:430–4
- Vinutha B, Prashanth D, Salma K, et al. (2007). Screening of selected Indian medicinal plants for acetylcholinesterase inhibitory activity. J Ethnopharmacol 109:359–63
- Wills ED. (1966). Mechanisms of lipid peroxide formation in animal tissues. Biochem J 99:667–76