Abstract
Context: The codling moth, Cydia pomonella L. (Tortricidae), is a major cosmopolitan pest of the apple. The potential of plant-derived semiochemicals for codling moth control is poorly studied.
Objective: To evaluate the potential of crude extracts of five plants from the Asteraceae family: Artemisia absinthium L., Artemisia arborescens L. “Powis Castle”, Artemisia annua L., and Artemisia ludoviciana Nutt. to prevent apple infestation by C. pomonella larvae and to identify the deterrent(s) in these plants.
Materials and methods: Artemisia dried leaves were extracted in v/v mixture of 80% ethanol, 10% isopropanol, and 10% of methanol, and the extracts were analyzed using high-performance thin layer chromatography. Preference of fruit treated with test solutions (Artemisia extracts or α-thujone) versus fruit treated with solvent was studied using choice assays.
Results: α-Thujone was detected in A. arborescens extract at a concentration of 77.4 ± 2.4 mg/g of dry tissue, localized between Rf 0.75 and 0.79 and was absent from crude extracts of remaining Artemisia species. Material from each extract in the zone between Rf 0.75 and 0.79 was removed from chromatographic plates and tested for feeding deterrence. Only the material from A. arborescens showed feeding deterrent properties. Minimum concentrations that prevented fruit infestation were 10 mg/ml for α-thujone and 1 mg/ml for A. arborescens crude extract.
Discussion and conclusions: Artemisia arborescens contains chemicals that prevent apple infestation by codling moth neonates. Thujone is one of these chemicals, but it is not the only constituent of A. arborescens crude extract that prevents fruit infestation by codling moth neonates.
Introduction
The codling moth, Cydia pomonella L. (Tortricidae) (), is the major cosmopolitan pest of apples. In most locations, C. pomonella has two or three generations per year, and in unmanaged orchards, fruit infestation by this species may reach up to 80% (Arthurs et al., Citation2005; Tadic, Citation1957). This potentially translates to losses exceeding US$2.1 billion in the United States alone (Geisler, Citation2012).
Figure 1. The codling moth and two examples of fruit damage caused by this pest. (A) The moths immobilized on the sticky board of monitoring trap. The wingspan of the moth equals approximately 2 cm. (B) Codling moth neonate larva, about 1.5 mm long, is the invasive stage. (C) Fruit damage caused by infestation early in the season. (D) Fruit damage caused by infestation late in the season, about 30 d before harvest.
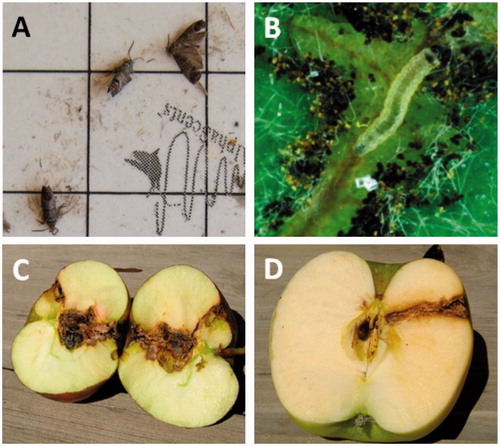
The grower has limited options to control the codling moth because shortly after hatching larvae burrow into the fruit and stay there until development is complete. This is especially problematic in growing areas that have partial third generations where neonates attempt to penetrate the fruit within days of harvest, the time when insecticides are not permitted to be used.
Sprays containing the broad-spectrum organophosphate neurotoxin, azinphos-methyl, are an effective control measure for C. pomonella; however, this insecticide must be applied at high levels (1.7 kg/hectare) due to resistance that has accumulated over the years. Due to serious concerns raised by the Environmental Protection Agency, azinphos-methyl is no longer allowed to be used on apples in the USA, has also been banned in European Union, and soon will be banned in New Zealand. The insect ryanodine receptor activator, Rynaxypyr, chloride channel activator, emamectin benzoate, and disruptors of nicotinic/γ amino butyric acid (GABA)-gated chloride channels, such as Spinetoram, have been implemented against the codling moth in the USA and in Europe, but the development of resistance to these insecticides is only a matter of time. Microbial insecticides such as Bacillus thuringiensis or codling moth granulosis virus could be used against codling moth neonates, but these insecticides must be consumed in large quantities to exert effects, thus their efficacy is often too low to provide fruit of satisfactory quality.
Pheromone- or kairomone-based strategies such as attract-and-kill and mating disruption are expensive and do not resolve problems caused by dense codling moth populations, and migration of gravid moths from adjacent unmanaged areas in mosaic landscapes typical of small-scale apple production (Witzgall et al., Citation2008) or insecticide resistance (Poullot et al., Citation2001). Recent studies using microencapsulated kairomones against codling moth showed promising results (Light & Beck, Citation2010). However, further research on alternative strategies of controlling the codling moth is needed. Preventing fruit infestation by neonate larvae with feeding deterrents of plant origin is a novel strategy, and may be useful especially prior to harvest when all insecticides are restricted.
Some plants belonging to the Asteraceae family and Artemisia genus exhibit arthropod-repellent properties (Abad et al., Citation2012). Terpinen-4-ol isolated from Artemisia vulgaris L. repelled the yellow fever mosquito Aedes aegypti L. (Hwang et al., Citation1985). Essential oils from A. vulgaris and Artemisia annua L. repel the red flower beetle, Tribolium castaneum Herbst (Tripathi et al., Citation2000; Wang et al., Citation2006). Coumarin and thujyl alcohol from Artemisia abrotanum L. repel the tick Ixodes ricinus L. (Tunón et al., Citation2006).
There are also reports that chemicals from plants belonging to the Artemisia genus inhibit feeding in insects, including codling moth larvae. Phenylacetylenes from growing buds of Artemisia capillaris Thunb. are insect anti-feedants (Yano, Citation1983, Citation1987). 1,8-Cineole from A. annua is a feeding deterrent in T. castaneum (Tripathi et al., Citation2001). Ethanolic extracts from A. annua as well as artemisinin, a sesquiterpene lactone isolated from this plant, inhibit feeding of the South American ladybird beetle, Epilachna paenulata Germar, and caterpillars of the southern armyworm Spodoptera eridania Stoll (Maggi et al., Citation2005). Crude extracts (single doses of 10 mg/ml) from Artemisia absinthium L. lowered apple infestation by codling moth neonates in preliminary experiments by Suomi et al. (Citation1986); however, the methodology of their bioassay did not allow accurate evaluation of Artemisia’s repellent properties (due to oversized test arena, 33% of tested larvae never found either treated or control apples in their study). Single doses (10 mg/ml) of crude extracts from A. arborescens and Artemisia ludoviciana Nutt. had deterrent effects in experiments of Durden et al. (Citation2008, Citation2009) who used improved bioassay for feeding deterrence of the extracts. In a more recent study (Durden et al., Citation2011), crude extracts from A. annua, and two of its metabolites, artemisinin or 1,8-cineole, were shown to prevent apple infestation by codling moth neonates.
Concentration-dependent effects of A. arborescens extracts and thujone, a metabolite found in some species belonging to the Artemisia genus, on feeding by codling moth neonates, have not been previously studied. Here, we show that A. arborescens extracts, and a thujone stereoisomer, α-thujone, prevent fruit infestation by codling moth neonates.
Materials and methods
Plant material
The aerial parts of A. arborescens “Powis Castle” were collected in Ozark Arboretum, Mountain Grove, MO, in May 2009 and were air dried at room temperature. A sample was authenticated by S. Lallemand, and vouchered, as MSFES/CIP 006, in the Collection of Insects and Plants at Missouri State Fruit Experiment Station in Mountain Grove, MO.
Insects
Codling moths from USDA-ARS Yakima Agricultural Research Laboratory, Wapato, WA, were held at 25 °C, 70–80% relative humidity (RH), under a 16 h light, 8 h dark (16 L:8D) light-dark regime. Female moths were allowed to oviposit on strips of polyethylene. Neonates, up to 1 h old, were collected and used for the experiments.
Chemicals and crude plant extracts
Dehydration alcohol (a v/v mixture of 80% ethanol, 10% isopropanol, and 10% of methanol) was purchased from EMD (Gibbstown, NJ). All other chemicals, including methanol, toluene, ethyl acetate, glacial acetic acid (99.9% purity), and α-thujone (96% purity), were from Sigma-Aldrich (St Louis, MO). The powdered air-dried leaf matter (150 mg) was placed in a 2-ml Eppendorf tube with 1 ml dehydration alcohol, vortexed briefly, allowed to rest for 10 min at room temperature, and centrifuged at 2000 × g for 10 min. The liquid fraction was removed with a pipette, transferred to a pre-weighed Eppendorf tube, and evaporated in a SpeedVac rotary evaporator. After desiccation, the tubes were re-weighed to determine the final mass of the residue which was subsequently resuspended in dehydration alcohol to the desired concentration.
Experimental design
First, crude alcoholic extracts of aforementioned Artemisia species were compared by qualitative high-performance thin-layer chromatography (HPTLC), in an attempt to discern chemical(s) of differences among the particular species. Differential bands were isolated by scraping off the appropriate stationary phase area on the TLC plate from each of the lanes representing a different Artemisia species. The samples were then extracted with dehydration alcohol, evaporated, re-dissolved at 10 mg/ml, and their potential for preventing apple infestation by codling moth neonates was evaluated as described below. Because only the sample from A. arborescens prevented fruit infestation, the band of difference was present only in the lane representing A. arborescens, and the band of difference co-localized with α-thujone standard, we further concentrated on bioassaying α-thujone alone and A. arborescens crude extract in attempt of producing concentration-dependent response curves. Thujone was bioassayed at concentrations of 1, 3, 10, 30, and 100 mg/ml. Artemisia arborescens crude extract was bioassayed at concentrations of 0.1, 0.3, 1, 3, and 10 mg/ml.
HPTLC
HPTLC plates (10 × 10 cm; Silica gel 60 F254, purchased from EMD, Gibbstown, NJ) were washed in methanol and dried under a fume hood. Crude Artemisia extracts were spotted using a CAMAG Nanomat 4 dispenser at 50 µg per lane. Thujone () standard was spotted at 25 or 50 µg per lane. The plates were allowed to air dry for 5 min and developed in a CAMAG Horizontal Developing Chamber using a mobile phase consisting of toluene, ethyl acetate, and glacial acetic acid (8:2:0.2 v/v). The plates were then air-dried for 5 min, derivatized in vanillin reagent, and heated for 10 min on a CAMAG TLC Plate Heater III at 100 °C. The plate was then scanned in white light using a Canon CanoScan 5200F flatbed scanner for documentation and thujone quantification was performed by densitometry as described in Valverde and This (Citation2007). Briefly, the image was scanned and registered as a JPEG compressed file. TLC Analyzer (Massachusetts Institute of Technology, Cambridge, MA) a public domain computer program (Hess, Citation2007) was used to analyze digital images of HPTLC plates and generate multispectral scans, densitograms, and calibration curves for thujone.
Figure 2. Chemical structure of a secondary metabolite of Artemisia arborescens “Powis Castle”, α-thujone, tested for codling moth deterrence in this study. α -Thujone exhibited weak deterrent effects, which do not explain the level of deterrent activity in A. arborescens “Powis Castle” crude extracts toward codling moth neonates, suggesting the presence of other codling moth deterrent compounds in this plant.
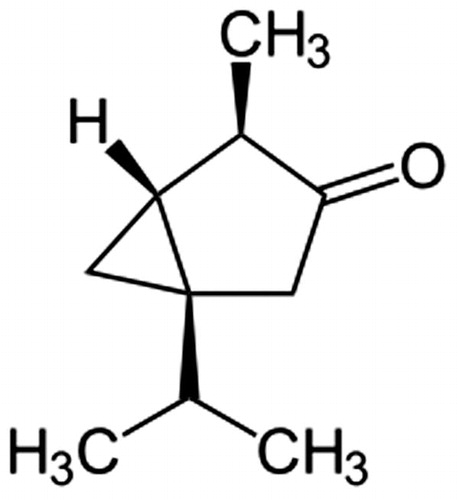
Bioassays
Test solutions were examined for deterrent effects on codling moth neonates at concentrations ranging from 0.1 to 100 mg/ml. For each dose, four plugs were taken from the same Golden Delicious apple, using a length of plastic soda straw, such that the straw covered the pulp, but not the epidermis of the apple. The crevice between the plug and the edge of the straw was sealed with paraffin wax applied with a warm spatula. The straws were then placed in a holder, apple plug facing up, and 5 μl of test solution were applied to each plug. The plugs were allowed to air dry, and four plugs were placed in a 60 × 15 mm polystyrene Petri dish, held in place by small pieces of modeling clay. New clay was used for each assay. A glass rod (1.3 mm diameter, 25–27 mm long) was positioned such that each end of the rod touched both the control and the treated member of the plug pair. One neonate was placed, using a camel-hair artists brush, in the middle of the glass rod and the Petri dish was covered with a lid. The entire assembly was covered with a one half of a white plastic RipBall (TM & Enor Corp., Northvale, NJ) to provide a white, slightly opaque cupola and placed on the testing bench illuminated by fluorescent tubes and Soft White 60 general purpose bulbs (General Electric Canada, Cleveland, OH). Such an arrangement provided dispersed, non-directional light of uniform luminosity (900–920 lux) over each test arena, which is important because codling moth neonates exhibit mild phototropism (Jackson, Citation1982). Assays were evaluated after 20 h to determine which plug was fed upon. A feeding deterrence index was calculated as described in Jones (Citation1952) by dividing the number of neonates feeding on apple plugs treated with Artemisia extracts by the number of neonates feeding on the plugs treated with dehydration alcohol only, subtracting this figure from 1, and multiplying the result by 100. Prior to every experiment, glass rods and Petri dishes were washed sequentially in tap water, double-distilled water, alcohol, and then dried. In contrast with the bioassay of Suomi et al. (Citation1986) where one-third of tested larvae did not find the fruit, our method assures that 100% of tested larvae feed on apple plugs. See Durden et al. (Citation2008) for a detailed description of this bioassay.
Statistics
Exact Fisher’s test (α = 0.05) was used in all bioassays to test the null hypothesis that neonates do not discriminate between plugs or apples treated with plant extract and those treated with alcohol (i.e., 50% of the neonates choose treated plugs or apples and 50% of the neonates choose control plugs or apples).
Results
HPTLC analysis of Artemisia extracts
In order to test the ability of various Artemisia species to deter codling moth neonates from apples, we initially compared crude alcohol extracts of Artemisia leaves by qualitative HPTLC. Derivatized α-thujone produced a pink band that located at Rf 0.75–0.79 in the lane containing the crude extract from A. arborescens. Noteworthy, α-thujone was absent from the A. absinthium, A. annua, and A. ludoviciana crude extracts. The α-thujone concentration in the A. arborescens extract averaged 77.4 ± 2.4 mg/g (n = 16).
Feeding deterrent effects of crude Artemisia extracts isolated from HTPLC plates
Each of the crude Artemisia extracts was examined in a deterrent assay (Durden et al., Citation2008) with codling moth neonates. Only the material from A. arborescens showed feeding deterrent properties at 10 mg/ml (, n = 24, p < 0.01, Fisher’s exact test). The calculated deterrence index was 90.9. The remaining crude extracts, isolated from HPTLC plates at Rf 0.75–0.79, did not have any detectable feeding deterrent properties.
Table 1. Comparative feeding deterrence of extracts isolated from Artemisia absinthium, Artemisia arborescens, Artemisia annua, and Artemisia ludoviciana chromatograms.
Concentration-dependent, feeding deterrent effects of crude Artemisia arborescens extract and α-thujone alone
A pure preparation of commercially available α-thujone prevented fruit infestation by codling moth neonates at concentrations of 10, 30, and 100 mg/ml (, n = 34–44, p < 0.05, Fisher’s exact test) and exhibited moderate deterrence indexes ranging from approximately 70 to 78. The crude extract from A. arborescens was more effective and prevented fruit infestation by codling moth neonates at concentrations as low as 1 mg/ml (, n = 46, p < 0.01, Fisher’s exact test), with higher deterrence indices, in the 80–90 range.
Table 2. Concentration-dependent feeding deterrence of α-thujone against codling moth neonates.
Table 3. Concentration-dependent feeding deterrence of the crude extract from Artemisia arborescens “Powis Castle” against codling moth neonates.
Discussion
The botanical insecticide, repellent, and deterrent field has grown tremendously in the past decade. Plants of the Artemisia family have been one the area of interest in these studies. However, recent reports have concentrated mainly on the effects of Artemisia on stored product beetles such as T. castaneum, Callosobruchus maculatus, Sitophilus oryzae, Sitophilus granaries, or Rhyzopertha dominica (Kordali et al., Citation2006; Liu et al., Citation2006; Negahban et al., Citation2006a,Citationb; Sharifian et al., Citation2012; Wang et al., Citation2006) or in actual and potential malaria vectors such as mosquitoes (Devi et al., Citation2010; El-Sheikh, Citation2009; Panneerselvam et al., Citation2012; Tonk et al., Citation2006; Tunón et al., Citation2006). The same trend can be observed in recent studies on the effects of thujone on insects (Hill et al., Citation2009; Ghaninia et al., Citation2007; Lee et al., Citation2003; Liu et al., Citation2010; Syed & Leal, Citation2008; Sener et al., Citation2009). In all aforementioned species, Artemisia extracts had toxic and/or repellent properties.
Only recent studies have intensively examined the feeding deterrent or repellent effects that Artemisia extracts or essential oils have in economically important caterpillars such as Plodia interpunctella Hübner (Karahroodi et al., Citation2009), Glyphodes pyloalis Walker (Khosravi et al., Citation2010), Plutella xylostella L. (Sharma et al., Citation2006), Pieris rapae L. (Hasheminia et al., Citation2011), or Spodoptera frugiperda J. E. Smith (Knaak et al., Citation2013). In all cases, Artemisia extracts or essential oils exhibited either larvicidal or repellent and anti-feedant properties. Reports on the effects of dietary thujone on caterpillars are scarce; we are aware of only one paper (Lee et al., Citation1999) that shows larvicidal activity and growth inhibitory effect of thujone against the European corn borer, Ostrinia nubilalis Hübner. To the best of our knowledge, our current study demonstrates for the first time that A. arborescens and thujone prevent fruit infestation by internal fruit feeding larvae.
We did not find α-thujone in the crude extracts from A. absinthium, A. annua, and A. ludoviciana. This finding is consistent with recent reports of the chemical composition of plants from the Artemisia genus, which often occur as chemotypes that are morphologically indistinguishable, but chemically different. Artemisia absinthium is a good example here: chemotypes from Siberia, Bashkir, or Morocco may contain significant amounts of α-thujone ranging from 16% to almost 40% of essential oil (Blagojevic et al., Citation2006; Derwich et al., Citation2009), whereas those from Italy contain only trace amounts of α-thujone (Blagojevic et al., Citation2006). Similarly, A. absinthium from Northern Iran contains negligible amounts of α-thujone (Rezaeinodehi & Khangholi, Citation2008).
Recent analysis by GC/MS (Radulović et al., Citation2013) showed the absence of α-thujone in essential oil from Serbian A. annua. Earlier works of Holm et al. (Citation1997) and Goel et al. (Citation2008) yielded similar results: thujone has not been found in any of seven A. annua chemotypes originating from regions as diverse as China, Tasmania, Hungary, France, the former Yugoslavia, Italy, and India.
Blust and Hopkins (Citation1987) found α-thujone in essential oil from A. ludoviciana collected in Kansas. Although the concentration was not reported, visual inspection of gas chromatograms published in their paper suggests that α-thujone was a minor component of the oil in their study. A newer study by Lopes-Lutz et al. (Citation2008) on the chemical composition of Canadian A. ludoviciana demonstrated absence of thujones in essential oil obtained from foliage of this plant.
Tucker et al. (Citation1993) reported about 2.5% of α-thujone in essential oil of A. arborescens “Powis Castle”. Our estimation that crude extract of the same cultivar contains approximately 7.5% of α-thujone is generally consistent with these findings.
In our experiments, only the material from A. arborescens “Powis Castle” contained in the area of chromatograms between Rf 0.75 and 0.79, where α-thujone standards localized, showed feeding deterrent properties (). This finding strongly suggested that α-thujone produced by A. arborescens prevents fruit infestation by the codling moth. Indeed, α-thujone alone, tested with our bioassays, showed feeding deterrent properties (). However, comparison of deterrent activities of various α-thujone concentrations () with those obtained by dilutions of A. arborescens “Powis Castle” crude extracts () indicates that α-thujone is not the only insect deterrent chemical that these extracts contain; the crude plant extract is deterrent at concentration 10 times lower than minimum effective concentration of α-thujone.
The crude extract from A. arborescens “Powis Castle” prevented apple infestation by codling moth neonates in a dose-dependent manner (), and with a efficacy similar to that exhibited by A. annua in our earlier study (Durden et al., Citation2011), with a minimal effective concentration of 1 mg/ml in both cases. Similar to crude extract from A. annua, the crude extract from A. arborescens “Powis Castle” had more pronounced deterrent properties against codling moth neonates than crude extract from A. absinthium that we investigated earlier (Durden et al., Citation2009). Crude A. absinthium extract showed deterrent properties only at concentrations of 10 mg/ml and higher (Durden et al., Citation2009).
Because both A. arborescens “Powis Castle” crude extract and α-thujone exert their codling moth deterrent properties at relatively high concentrations, their use as sprays in codling moth control would probably be impractical. Perhaps small-scale organic or environmentally conscious growers could use extracts of A. arborescens “Powis Castle” as a technique supplementary to mating disruption. Also, it should be noted that the possible use of sprays containing α-thujone may raise some objections. α-Thujone has been found to act as a GABA receptor antagonist both in insects and mice and is regarded as detrimental to human health (Höld et al., Citation2000).
In our experiments, we used apple plugs of standardized surface area (28 mm2) and a standard volume of α-thujone (5 μl at 10 mg/ml) had to be applied to each apple plug to prevent fruit infestation. This allowed for a calculation how much of α-thujone would have to be applied to apples at the time close to harvest. Assuming that the apples are of 8 cm diameter each, and there are 6 apples per kilogram, the amount of α-thujone deposit resulting from direct spray would equal approximately 200 mg/kg of fruit, well above the levels of α-thujone presently permitted in food or beverages in the USA and European Union (Demyttenaere, Citation2012; Pelkonen et al., Citation2013). However, the fact that α-thujone is a GABA receptor antagonist acting similar to many insecticide prototypic substances (Casida & Palmer, Citation1988) and insecticides (Casida & Durkin, Citation2013) suggests screening insecticides that block GABA receptors for their feeding deterrent properties as a new direction of future research on codling moth-feeding deterrents. It may be that some commercially available insecticides prevent apple infestation by the codling moth at concentrations much lower than those required for killing the insect. This way the grower could possibly greatly reduce the field rates of insecticide application (and detrimental impact on the environment) and still maintain satisfactory levels of codling moth neonate control.
Conclusions
Our research showed that A. arborescens “Powis Castle” contains substances that prevent fruit infestation by internal fruit-feeding larvae and identified α-thujone as one of these substances. Our data indicates that there are additional insect feeding deterrents present in A. arborescens “Powis Castle”. Based on this study, we argue that using this plant or α-thujone as large-scale sprays in codling moth control would probably be impractical. However, our findings delineate a new area of research on codling moth neonate-feeding deterrents based on the screening of insecticides that block GABA receptors for their potential of preventing fruit infestation.
Acknowledgements
The authors thank Ms. Pauline Anderson and Mr. Jim Harris (YARL, Wapato, WA) for their assistance with insect breeding and Miss Kelsey Long for help with the bioassays. We are indebted to Dr. Luis Matos (Eastern Washington University, Cheney, WA, USA) for kindly providing the photo of codling moth neonate.
Declaration of interest
This research was funded, in part, by USDA-NLGCA LFASFS, Missouri State University Faculty Research Program, MSU Graduate College Funding and Awards, and The Herb Society of America Inc.
References
- Abad MJ, Bedoya LM, Apaza L, Bermejo P. (2012). The Artemisia L. genus: A review of bioactive essential oils. Molecules 17:2542–66
- Arthurs SP, Lacey LA, Fritts R Jr. (2005). Optimizing use of codling moth granulovirus: Effects of application rate and spraying frequency on control of codling moth larvae in Pacific Northwest Apple Orchards. J Econ Entomol 98:1459–68
- Blagojevic P, Radulovic N, Palic R, Stojanovic G. (2006). Chemical composition of the essential oils of Serbian wild-growing Artemisia absinthium and Artemisia vulgaris. J Agric Food Chem 54:4780–9
- Blust MH, Hopkins TL. (1987). Gustatory responses of a specialist and a generalist grasshopper to terpenoids of Artemisia ludoviciana. Entomol Exp Appl 45:37–46
- Casida JE, Durkin KA. (2013). Neuroactive insecticides: Targets, selectivity, resistance, and secondary effects. Annu Rev Entomol 58:99–117
- Casida JE, Palmer CJ. (1988). 2,6,7-Trioxabicyclo[2.2.2]octanes: Chemistry, toxicology and action at the GABA-gated chloride channel. In: Biggio G, Costa E, eds. Chloride Channels and Their Modulation by Neurotransmitters and Drugs. Advances in Biochemical Psychopharmacology, vol. 45. Raven: New York, 109–123
- Demyttenaere JC. (2012). The new European Union Flavouring Regulation and its impact on essential oils: Production of natural flavouring ingredients and maximum levels of restricted substances. Flavour Frag J 27:3–12
- Derwich E, Benziane Z, Boukir A. (2009). Chemical compositions and insectisidal activity of essential oils of three plants Artemisia sp.: Artemisia herba-alba, Artemisia absinthium and Artemisia pontica (Morocco). Electron J Environ, Agric Food Chem 8:1202–11
- Devi RU, Lakshmi D, Aarthi N. (2010). Toxicity effect of Artemisia parviflora against malarial vector Anopheles stephensi Liston. J Biopesticides 3:195–8
- Durden K, Brown J, Pszczolkowski M. (2008). Extracts of Ginkgo biloba or Artemisia species reduce feeding by neonates of codling moth, Cydia pomonella (Lepidoptera: Tortricidae), on apple in a laboratory bioassay. J Entomol Soc B 105:83–8
- Durden K, Sellars S, Cowell B, et al. (2011). Artemisia annua extracts, artemisinin and 1,8-cineole, prevent fruit infestation by a major, cosmopolitan pest of apples. Pharm Biol 49:563–8
- Durden K, Sellars S, Pszczolkowski M. (2009). Preventing fruit infestation by codling moth neonates with Artemisia extracts. Pestycydy/Pesticides 104:51–6
- El-Sheikh TMY. (2009). Field evaluation of repellency effect of some plant extracts against mosquitoes in Egypt. J Egyptian Soc Parasitol 39:59–72
- Geisler M. (2012). Commodity Apple Profile. Agricultural Marketing Resource Center Web site. Available from: http://www.agmrc. org/commodities_products/fruits/apples/commodity-apple-profile [last accessed 25 Apr 2014]
- Ghaninia M, Ignell R, Hansson BS. (2007). Functional classification and central nervous projections of olfactory receptor neurons housed in antennal trichoid sensilla of female yellow fever mosquitoes, Aedes aegypti. Eur J Neurosci 26:1611–23
- Goel D, Mallavarupu GR, Kumar S, et al. (2008). Volatile metabolite compositions of the essential oil from aerial parts of ornamental and artemisinin rich cultivars of Artemisia annua. J Essent Oil Res 20:147–52
- Hasheminia SM, Sendi JJ, Jahromi KT, Moharramipour S. (2011). The effects of Artemisia annua L. and Achillea millefolium L. crude leaf extracts on the toxicity, development, feeding efficiency and chemical activities of small cabbage Pieris rapae L. (Lepidoptera: Pieridae). Pestic Biochem Phys 99:244–9
- Hess AVI. (2007). Digitally enhanced thin-layer chromatography: An inexpensive, new technique for qualitative and quantitative analysis. J Chem Educ 84:842–7
- Hill SR, Hansson BS, Ignell R. (2009). Characterization of antennal trichoid sensilla from female southern house mosquito, Culex quinquefasciatus Say. Chem Senses 34:231–52
- Höld KM, Sirisoma NS, Ikeda T, et al. (2000). α-Thujone (the active component of absinthe): γ-Aminobutyric acid type A receptor modulation and metabolic detoxification. Proc Natl Acad Sci USA 97:3826–31
- Holm Y, Laakso I, Hiltunen R, Galambosi B. (1997). Variation in the essential oil composition of Artemisia annua L. of different origin cultivated in Finland. Flavour Frag J 12:241–6
- Hwang Y-S, Wu K-H, Kumamoto J, et al. (1985). Isolation and identification of mosquito repellents in Artemisia vulgaris. J Chem Ecol 11:1297–306
- Jackson MD. (1982). Searching behavior and survival of 1st-instar codling moths. Ann Entomol Soc Am 75:284–9
- Jones GDG. (1952). The responses of the honey-bee to repellent chemicals. J Exp Biol 29:372–86
- Karahroodi ZR, Moharramipour S, Rahbarpour A. (2009). Investigated repellency effect of some essential oils of 17 native medicinal plants on adults Plodia interpunctella. Am Eurasian J Sustain Agric 3:181–4
- Khosravi R, Sendi JJ, Ghadamyari M. (2010). Effect of Artemisia annua L. on deterrence and nutritional efficiency of lesser mulberry pyralid (Glyphodes pylolais Walker) (Lepidoptera: Pyralidae). J Plant Protect Res 50:423–8
- Knaak N, Wiest SL, Andreis TF, Fiuza LM. (2013). Toxicity of essential oils to the larvae of Spodoptera frugiperda (Lepidoptera: Noctuidae). J Biopesticides 6:49–53
- Kordali S, Aslan I, Çalmaşur O, Cakir A. (2006). Toxicity of essential oils isolated from three Artemisia species and some of their major components to granary weevil Sitophilus granaries L.) (Coleoptera: Curculionidae). Ind Crop Prod 23:162–70
- Lee S, Peterson CJ, Coats JR. (2003). Fumigation toxicity of monoterpenoids to several stored product insects. J Stored Prod Res 39:77–85
- Lee S, Tsao R, Coats JR. (1999). Influence of dietary applied monoterpenoids and derivatives on survival and growth of the European corn borer (Lepidoptera: Pyralidae). J Econ Entomol 92:56–67
- Light DM, Beck JJ. (2010). Characterization of microencapsulated pear ester (2E,4Z)-ethyl-2,4-decadienoate: A kairomonal spray adjuvant against neonate codling moth larvae. J Agric Food Chem 58:7838–45
- Liu CH, Mishra AK, Tan RX, et al. (2006). Repellent and insecticidal activities of essential oils from Artemisia princeps and Cinnamomum camphora and their effect on seed germination of wheat and broad bean. Bioresour Technol 97:1969–73
- Liu ZL, Liu QR, Chu SS, Jiang GH. (2010). Insecticidal activity and chemical composition of the essential oils of Artemisia lavandulaefolia and Artemisia sieversiana from China. Chem Biodivers 7:2040–5
- Lopes-Lutz D, Alviano DS, Alviano CS, Kolodziejczyk PP. (2008). Screening of chemical composition, antimicrobial and antioxidant activities of Artemisia essential oils. Phytochemistry 69:1732–8
- Maggi ME, Mangeaud A, Carpinella MC, et al. (2005). Laboratory evaluation of Artemisia annua L. extract and artemisinin activity against Epilachna paenulata and Spodoptera eridania. J Chem Ecol 31:1527–36
- Negahban M, Moharramipour S, Sefidkon F. (2006a). Fumigant toxicity of essential oil from Artemisia sieberi Besser against three stored-product insects. J Stored Prod Res 43:123–8
- Negahban M, Moharramipour S, Sefidkon F. (2006b). Chemical composition and insecticidal activity of Artemisia scoparia essential oil against three coleopteran stored-product insects. J Asia Pacific Entomol 9:381–8
- Panneerselvam C, Murugan K, Kovendan K, Kumar PM. (2012). Mosquito larvicidal, pupicidal, adulticidal, and repellent activity of Artemisia nilagirica (Family: Compositae) against Anopheles stephensi and Aedes aegypti. Parasitol Res 111:2241–51
- Pelkonen O, Abass K, Wiesner J. (2013). Thujone and thujone-containing herbal medicinal and botanical products: Toxicological assessment. Regul Toxicol Pharm 65:100–7
- Poullot D, Beslay D, Bouvier JC, Sauphanor B. (2001). Is attract-and-kill technology potent against insecticide-resistant Lepidoptera? Pest Manag Sci 57:729–36
- Radulović NS, Randjelović PJ, Stojanović NM, et al. (2013). Toxic essential oils. Part II: Chemical, toxicological, pharmacological and microbiological profiles of Artemisia annua L. volatiles. Food Chem Toxicol 58:37–49
- Rezaeinodehi A, Khangholi S. (2008). Chemical composition of the essential oil of Artemisia absinthium growing wild in Iran. Pakistan J Biol Sci 11:946–9
- Sener O, Arslan M, Demirel N, Uremis I. (2009). Insecticidal effects of some essential oils against the confused flour beetle (Tribolium confusum du Val) (Col.: Tenebrinoidea) in stored wheat. Asian J Chem 21:3995–4000
- Sharifian I, Hashemi SM, Aghaei M, Alizadeh M. (2012). Insecticidal activity of essential oil of Artemisia herba-alba Asso against three stored product beetles. Biharean Biol 6:90–3
- Sharma A, Kaushal P, Sharma KC, Kumar R. (2006). Bioefficacy of some plant products against diamond back moth Plutella xylostella L. (Lepidoptera: Yponomeutidae). J Entomol Res 30:213–17
- Suomi D, Brown JJ, Akre RD. (1986). Responses to plant extracts of neonatal codling moth larvae Cydia pomonella (L.), (Lepidoptera: Tortricidae: Olethreutinae). J Entomol Soc B C 83:12–18
- Syed Z, Leal WS. (2008). Mosquitoes smell and avoid the insect repellent DEET. Proc Natl Acad Sci USA 105:13598–603
- Tadic MD. (1957). The Biology of the Codling Moth (Carpocapsa pomonella L.) as the Basis for Its Control. Beograd, Yugoslavia: Univerzitet u Beogradu, 9–10
- Tonk S, Bartarya R, Kumari KM, et al. (2006). Effective method for extraction of larvicidal component from leaves of Azadirachta indica and Artemisia annua Linn. J Environ Biol 27:103–5
- Tripathi AK, Prajapati V, Aggarwal KK, et al. (2000). Repellency and toxicity of oil from Artemisia annua to certain stored-product beetles. J Econ Entomol 93:43–7
- Tripathi AK, Prajapati V, Aggarwal KK, Kumar S. (2001). Toxicity, feeding deterrence, and effect of activity of 1,8-cineole from Artemisia annua on progeny production of Tribolium castanaeum (Coleoptera: Tenebrionidae). J Econ Entomol 94:979–83
- Tucker AO, Maciarello MJ, Sturtz G. (1993). The essential oils of Artemisia ‘Powis Castle’and its putative parents, A. absinthium and A. arborescens. J Essent Oil Res 5:239–42
- Tunón H, Thorsell W, Mikiver A, Malander I. (2006). Arthropod repellency, especially tick (Ixodes ricinus), exerted by extract from Artemisia abrotanum and essential oil from flowers of Dianthus caryophyllum. Fitoterapia 77:257–61
- Wang J, Zhu F, Zhou X, et al. (2006). Repellent and fumigant activity of essential oil from Artemisia vulgaris to Tribolium castaneum (Herbst) (Coleoptera: Tenebrionidae). J Stored Prod Res 42:339–47
- Witzgall P, Stelinski L, Gut L, Thomson D. (2008). Codling moth management and chemical ecology. Annu Rev Entomol 53:503–22
- Valverde J, This H. (2007). Quantitative determination of photosynthetic pigments in green beans using thin-layer chromatography and a flatbed scanner as densitometer. J Chem Educ 84:1505–7
- Yano K. (1983). Insect antifeeding phenylacetylenes from growing buds of Artemisia capillaris. J Agric Food Chem 31:667–8
- Yano K. (1987). Minor components from growing buds of Artemisia capillaris that act as insect antifeedants. J Agric Food Chem 35:889–8