Abstract
Context: Despite several studies on the effects of Solidago chilensis Meyen (Asteraceae), the phytochemical and hypolipidemic properties remain underappreciated.
Objective: This study evaluates the hypolipidemic and antioxidant effects of hydroalcoholic extract (HE) and quercetrin from S. chilensis aerial parts in cholesterol-fed rats.
Materials and methods: The HE was analyzed by high-performance liquid chromatography, followed by quercetrin isolation. Hypercholesterolemic rats (1% cholesterol and 0.5% cholic acid for 15 d) were treated with HE (150, 300, and 600 mg/kg p.o.; n = 6), simvastatin (4 mg/kg p.o.; n = 6), or quercetrin (10 mg/kg p.o.; n = 6) once a day for 30 d. During this period, a high-cholesterol diet was maintained until the 30th day of treatment.
Results: Rats treated with HE (150, 300, and 600 mg/kg) and quercetrin showed decreased serum levels of total cholesterol (−19.9, −27.5, −31.0, and −39.4%), lipoprotein-cholesterol (−36.0, −37.5, −43.3, and −59.4%), and triacylglycerides (−15.6, −23.5, −29.8, and −27.2%) when compared with the control group similar to simvastatin. Moreover, treatment with HE and quercetrin decreased hepatic 3-hydroxy-3-methylglutaryl coenzyme A (HMG-CoA) reductase activity (35.1% on average) and increased fecal cholesterol levels (38.2% on average).
Discussion and conclusions: Our results suggest that hypolipidemic effects of HE are associated with it modulating the activity of HMG-CoA reductase and its interference in the reabsorption and/or excretion of intestinal lipids. Solidago chilensis and its main constituent, quercetrin, may thus be effective as cholesterol-lowering agents and in preventing atherosclerosis.
Introduction
High plasma levels of total cholesterol (TC), low density lipoprotein-cholesterol (LDL-C), and triacylglycerides (TG), along with oxidative stress and reductions in high-density lipoprotein (HDL-C) levels, are associated with an increased risk of cardiovascular diseases (Dai et al., Citation2013; Lee et al., Citation2010). Many studies have shown that elevated plasma cholesterol concentrations can cause coronary atherosclerosis (Park & Velasquez, Citation2012) and that this effect is associated with heart disease, stroke, and death in both developed and developing countries (Raida et al., Citation2008). In addition, increases in serum lipid levels have been considered a major risk factor for liver disturbances such as non-alcoholic fatty liver disease, involving oxidative stress, inflammation, liver damage, and fat deposition (Brunt, Citation2004). Although TG are the main lipids that are accumulated in hyperlipidemia-induced hepatic diseases, increased TC levels have also been associated with cell and tissue damage in the liver (Tabas, Citation2002).
The currently available therapy for hypercholesterolemia involves the use of statins, which act by inhibiting 3-hydroxy-3-methylglutaryl coenzyme A (HMG-CoA) reductase, thereby reducing cholesterol synthesis (Istivan, Citation2003). These drugs are used as monotherapy or in combination, but they are associated with numerous serious adverse effects (Bao et al., Citation2012).
Efforts have recently been dedicated to the identification of natural compounds capable of reducing and/or regulating serum cholesterol and triacylglycerol levels. In this sense, medicinal plants have played a major role in the development of new therapeutic agents (Nabi et al., Citation2013).
Solidago chilensis Meyen (Asteraceae) is a species native to the southern region of South America. It is an upright subshrub perennial that is slightly aromatic and 80–120 cm tall. It is widely distributed in the South and Southeast Brazil, where it is popularly known as “arnica-do-brasil”, and it is used as a substitute of true arnica (Arnica montana L., Asteraceae) for the relief of inflammation (Lorenzi & Matos, Citation2002). Its main chemical constituents are essential oils, acetophenones, diterpenoids (clerodano and labdano), saponins, and both free and glycosylated flavonoids, with quercetrin being the major constituent (Soares-Valverde et al., Citation2009; Torres et al., Citation1987).
Previous ethnopharmacological and clinical studies have described this species as having gastric protective (Schemeda-Hirchmann et al., Citation2002), wound-healing (Facury-Neto et al., Citation2004), antifungal (Webster et al., Citation2008), and anti-inflammatory properties (Tamura et al., Citation2009). Recently, there have been considerable advances in the treatment of lumbago (Da Silva et al., Citation2010). However, the cholesterol-lowering effects of S. chilensis in hypercholesterolemic animal models have not yet been studied.
Therefore, the objective of this study was to investigate the protective effects of S. chilensis on hyperlipidemia and hepatic alterations induced by a cholesterol-enriched diet in rats. For this purpose, levels of serum TC, TG, LDL-C, very low-density lipoprotein (VLDL), and HDL-C were determined, along with hepatic HMG-CoA reductase activity and fecal cholesterol levels. Oxidative stress parameters, liver weights, and serum alanine aminotransferase (ALT) activity were determined to detect possible alterations induced by the hypercholesterolemic diet. The in vitro free-radical scavenging properties of S. chilensis were also evaluated with the aim of identifying a possible antioxidant effect in vivo.
Materials and methods
Plant materials
Aerial parts of Solidago chilensis were collected in Chapecó, SC, Brazil (S 27° 06′ 38.83″/W 52° 34′ 26.52″). The plant samples were authenticated by Osmar dos Santos Ribas, the curator of the herbarium of the Botanical Museum of Curitiba, where a voucher specimen (MBM number 356792) was deposited.
Hydroalcoholic extract preparation
A sample of the dried aerial parts of S. chilensis (500 g) of the same particle size was collected by passage through a mesh (425 μm; 35 Tyler/Mesch). The sample was exhaustively extracted in a percolator tube with 70% EtOH (v/v). Hydroalcoholic extract (HE) of S. chilensis was concentrated to dryness under reduced pressure at 40 °C and then freeze dried and stored at −20 °C.
High-performance liquid chromatography analysis
Chromatographic analysis was performed using a Varian® Pro-Star high-performance liquid chromatography (HPLC) system (Agilent Technologies, Santa Clara, CA) consisting of an automatic injector, ternary gradient detectors, pumps, and a UV/Vis Kromasil® C18 reversed-phase ODS column (Agilent Technologies, Santa Clara, CA) (5 µm; 25 × 4.5 mm2). The mobile phase consisted of two solvents: H2O:acetic acid (40:1 v/v; solvent A) and CH3CN (solvent B). Both solvents were filtered through 0.45 μm millipore polytetrafluoroethylene membranes. Separations were performed with a linear gradient as follows: 86% solvent A and 14% solvent B for 15 min, 35% solvent B for 30 min, and 100% solvent B for 2 min. The UV absorbance at 360 nm was measured, and the results were then compared with the retention times of an authentic external standard followed by a UV spectrum analysis. The flow rate of the mobile phase was 1 mL min−1 and the injection volume was 20 μL. The chromatographic runs were performed at 22 °C. The UV absorbance at 360 nm was measured (Apáti et al., Citation2006). Six different concentrations of quercetrin (12.5, 25, 50, 100, 200, and 450 µg/mL; Sigma-Aldrich®, St. Louis, MO) were analyzed in triplicates and a calibration curve was generated. HE was dissolved in MeOH (1 mg/mL) and filtered through a micropore filter (0.45 µm) before the chromatographic profile was generated. The results are expressed as the concentration of quercetrin per gram of HE and in the dried plant material.
Isolation of quercetrin
HE (20 g) was partitioned into equal volumes of solvents of increasing polarity. After freeze drying, the ethyl acetate fraction yielded a product weighing 3.1 g (representing 15.6% of the hydroethanolic extract mass). This product (1 g) was subjected to column chromatography with silica gel used as the stationary phase and CHCl3:EtOH (v/v) solution used as the eluent. One subfraction, F6 (0.6 g), was applied to a Sephadex LH-20 column (Fluka®, Jefferson Lab, Newport News, VA) with MeOH to yield the subfraction F6.1 (0.2 g). This subfraction F6.1 was then subjected to further fractionation by flash chromatography and eluted with ethyl acetate solutions containing increasing concentrations of MeOH (up to 100%) to yield the subfraction F6.1.1 (0.020 g), which was subjected to 1H- and 13C-nuclear magnetic resonance (NMR) spectroscopy (400 and 100 MHz, respectively; Bruker®, Bruker BioSpin Corporation, Billerica, MA) and electrospray ionization–mass spectrometry (ESI-MS) analysis.
In vitro 2,2-diphenyl-1-picrylhydrazyl (DPPH) radical-scavenging assay
Free radical-scavenging activity of the vegetal sample was measured using the method described by Brand-Williams et al. (Citation1995) with some modifications. HE (1 mL) and quercetrin (1 mL) (5–200 μg/mL) were added to 2 mL of a solution of 2,2-diphenyl-1-picrylhydrazyl (DPPH) radicals in ethanol (0.004%). The mixture was shaken vigorously and allowed to stand for 30 min at room temperature (RT). The absorbance (Abssample) of the resulting solution was measured at 517 nm and the antioxidant activity (AA) percentage was calculated using the following formula: AA% = 100 − {[(Abssample − Absblank) × 100]/Abscontrol}. A solution of ethanol (2.0 mL) and plant extract/quercetrin (1.0 mL) was used as the blank (Absblank). A solution of DPPH (2.0 mL) and ethanol (1.0 mL) was used as the control (Abscontrol). Ascorbic and gallic acids were used as standards. Free radical-scavenging activity was expressed in terms of the amount of antioxidants necessary to decrease the initial DPPH absorbance by 50% (IC50). The IC50 value was determined by interpolation from the non-linear regression of the plot of percentage of inhibition against the concentration of extract/quercetrin, which is defined as the amount of extract/quercetrin needed to scavenge 50% of DPPH radicals.
Animals
The International Guidelines for Care and Use of Laboratory Animals were followed for all experiments, and the experimental protocol was approved by the Ethics Committee on Animal Use (CEUA number #574) of the biological center of Federal University of Paraná, Brazil. The manuscript was prepared in compliance with the in vivo study guideline “ARRIVE”. Male Wistar rats (n = 42) weighing 250–275 g were used in the study. The animals were housed in wire-bottomed 17 × 33.5 × 40.5 cm cages in a controlled environment at 22 ± 2 °C with a 12-h light-dark cycle and minimal noise. The rats had ad libitum access to water and commercially prepared chow pellets (Nuvilab® CR-1, Millipore, Billerica, MA) for rodents.
Hyperlipidemic diet
The high cholesterol diet was prepared by mixing a normal basal diet [composition (w/w): 48.3% carbohydrate, 23.5% crude protein, 5.9% crude fat, 5.9% crude ash, and 3.9% crude fiber (Sam #31, Samtaco Bio Korea, Osan, Gyeonggi, Korea))] (Kin et al., Citation2008) with cholic acid and cholesterol (989.9:10:0.1). The mixture was pelleted prior to use.
Experimental design and sample collection
After 15 d of acclimatization, six animals were randomly designated as the normal group (N); these animals continued receiving food and water, ad libitum, until the end of the experiment. Another group of animals (n = 36) received a hypercholesterolemic diet (Nuvilab® CR-1 chow pellets for rodents) comprising 1% of cholesterol and 0.1% of cholic acid (Sigma-Aldrich®, Sigma, St. Louis, MO). After 2 weeks under these conditions, the animals were randomly divided into six groups of six animals each (n = 6) and then orally treated for 4 weeks as follows: group C (negative control) received distilled water (0.5 mL/100 g); groups HE 150, HE 300, and HE 600 received HE of S. chilensis at doses of 150, 300, and 600 mg/kg, respectively; group QRT (quercetrin) received 10 mg/kg quercetrin; and group SIMV (simvastatin powder) received 4 mg/kg simvastatin (Sigma-Aldrich®, Sigma, St. Louis, MO) (positive control). All drugs were diluted with distilled water in established doses (Adeneye et al., Citation2010; Balzan et al., Citation2013) submitted to ultrasound bath (40 kHz) at 20 °C by 30 min to facilitate solubility (Patel et al., Citation2008; Vilkhu et al., Citation2008; Wang & Weller, Citation2006) and administered by gavage in a volume of 0.5 mL/100 g body weight (Diehl et al., Citation2001; Trovato et al., Citation1996). During this period, the high cholesterol diet was continued until treatment day 30. Doses of HE, quercetrin, and simvastatin were based on previous studies (Pankaj et al., Citation2010).
Dietary intake was measured daily and body weights of animals were recorded before and every 3 d following the initiation of treatment. At the end of the experimental period and a subsequent 12 h of fasting, the animals were anesthetized by a mixture of ketamine and xylazine (75 and 10 mg/kg, respectively). Blood aliquots were collected for biochemical analyses via cardiac puncture, and the animals were then euthanized by an overdose of anesthetic. The livers were collected, weighed, and immediately homogenized for the evaluation of lipid peroxidation, antioxidant enzyme activity, and hepatic HMG-CoA reductase activity. The liver/body weight ratio (liver/body weight × 100) and cholesterol levels of feces were also calculated.
Biochemical analysis of blood samples
Upon collection, blood samples were immediately centrifuged at 3000 × g for 15 min. Serum TC, TG, HDL-C, and ALT levels were determined by enzymatic colorimetric methods (UV/Vis) using commercial Labtest® kits (ELISA Technologies, Gainesville, FL) according to the instructions of the manufacturer. A semi-automated analyzer (BioSystems®, Applied Biosystems, Waltham, model BTS 310) was used for all analysis (Li et al., Citation2012). Serum LDL-C and VLDL levels were calculated using the Friedewald equation (Friedewald, Citation1972): LDL-C = TC − [HDL-C − (TG/5)], and the atherogenic index (of TC/HDL-C) was calculated by the method described by Xia et al. (Citation2011).
Hepatic HMG-CoA reductase activity
HMG-CoA reductase activity was indirectly determined as the ratio of HMG-CoA and mevalonate (Rao & Ramakrishnan, Citation1975). The liver tissue was collected, immediately washed with saline, blotted on filter paper, weighed, segmented, and homogenized in Tris-HCl buffer (0.025 M and pH 7.5) to yield a 10% (w/v) liver homogenate. The homogenate was then centrifuged at 12 000 × g for 15 min, and the supernatant was collected and stored at −70 °C until further analysis. HMG-CoA activity was determined by its reaction with hydroxylamine hydrochloride at an alkaline pH, and the subsequent colorimetric measurement of the resulting hydroxamic acid generation by the formation of complexes with ferric salts was performed at 540 nm. Similarly, mevalonate activity was estimated by a reaction with the same reagent but at a pH of 2.1. At this pH, the lactone form of mevalonate readily reacts with hydroxylamine hydrochloride to form hydroxamate.
Fecal cholesterol analysis
Feces were collected during the last 3 d of the experiment, lyophilized, and milled into powder. Total lipids were extracted with chloroform/methanol (2:1; v/v) solution according to the method described by Folch et al. (Citation1957). Fecal powder (1 g) was mixed with 10 mL of chloroform and 5 mL of methanol solutions, shaken, stored at −20 °C for 24 h, and then centrifuged at 3000 × g for 15 min at RT. The suspension was filtered through Whatman filter paper No. 2, methanol was aspirated, and chloroform was evaporated. H2O (2 mL) was added, and a suspension was created using a bath sonicator. This suspension was used to estimate the fecal cholesterol content, which was estimated by the enzymatic method using the cholesterol oxidase assay at 500 nm.
Lipid peroxidation
To evaluate lipid peroxidation and antioxidant enzyme activity, the liver was homogenized in 3 volumes of 150 mM NaCl (pH 7.4) and centrifuged at 3000 × g and at 4 °C for 10 min yielding a low-speed supernatant, which was used for further analysis. Lipid peroxidation was evaluated by measuring the thiobarbituric acid reactive substances (TBARS) level (Ohkawa et al., Citation1979) after the addition of 7.2 mmol/L of butylated hydroxytoluene to the homogenates to prevent further oxidation. Liver homogenates were deproteinized with 10% trichloroacetic acid (TCA) and preincubated with 0.67% thiobarbituric acid (TBA) at 100 °C for 1 h. The colored product of the reaction was then extracted with n-butanol and measured at 535 nm using a standard curve of 1,1,3,3-tetraethoxypropane.
Determination of antioxidant enzymes activity
Superoxide dismutase activity was determined based on its ability to inhibit the autoxidation of adrenaline to adrenochrome at an alkaline pH (Misra & Fridovich, Citation1972). Catalase activity was determined using hydrogen peroxide (H2O2) as the substrate (Aebi, Citation1984). The pseudo-first-order reaction constant (k) of the decrease in the H2O2 absorption at 25 °C was determined and specific activity was expressed as k/g protein. Glutathione peroxidase activity was determined using glutathione reductase and NADPH. The method is based on the oxidation of NADPH, which is indicated by a decrease in the absorbance at 340 nm (Paglia & Valentine, Citation1967). Thioredoxin reductase (TrxR) activity was determined using 5,5′-dithiobis(2-nitrobenzoic acid) (DTNB) and NADPH (Holmgren & Björnstedt, Citation1995). The method is based on the reduction of DTNB, which is indicated by an increase in the absorbance at 412 nm. Gold (III) chloride trihydrate (500 nM) was used to inhibit TrxR activity (Omata et al., Citation2006) and determine non-TrxR DTNB reduction, which was subtracted from total DTNB reduction in order to obtain TrxR activity. The amount of DTNB reduced was calculated using an absorption coefficient of 13.6 × 103/mol/cm.
Statistics
All results shown are presented as mean values ± standard deviation. The data were evaluated by one-way ANOVA followed by Tukey’s test and correlation analyses using SPSS 20.0 (Chicago, IL). A p value of <0.05 was considered statistically significant.
Results
Chemical constituents of S. chilensis
The structure of the isolated substance was identified as glycosylated flavonoid quercetrin (quercetin-3-O-α-l-rhamnoside, 1), a yellow amorphous powder, by uni- and bi-dimensional NMR spectroscopic data analysis (UV, ESI-MS, and 1H and 13C NMR) and by comparing with data from literature (Correia et al., Citation2008; Tiberti et al., Citation2007).
The amount of quercetrin in HE was quantified by HPLC using an analytical curve (r = 0.9999; y = 411127x − 190316) with a retention time of 27.60 min. The HPLC analysis revealed quercetrin concentration to be 0.2916 g of quercetrin/g of total extract, which was equivalent to 110.8 g. Thus, the aerial parts of S. chilensis were found to contain 6.5% quercetrin.
Determination of DPPH radical-scavenging activity
DPPH assay showed that HE exhibits antioxidant properties in vitro. The highest scavenging effect was observed for QRT with an IC50 value of 23.78 ± 2.64 μg/mL followed by HE 71.24 ± 5.29 μg/mL, although both showed lower scavenging abilities than ascorbic and gallic acids, which were used as standards (14.39 ± 2.16 and 3.24 ± 0.29 μg/mL, respectively).
Animal body and liver weights
All experimental animals gained weight from the beginning to the end of the experiment. However, no differences in weight gain were observed between the groups during the experimental period (). The average liver weight was significantly higher in rats that received the high-cholesterol diet only (group C) as compared with the other groups. The different doses of HE, QRT, and SIMV attenuated the increase in liver weight induced by the hypercholesterolemic diet of these respective groups (HE, QRT, and SIMV) compared with group C (F(6, 34) = 13.21; p < 0.005), even though the liver weights remained higher than those of group N. Treatment with QRT and SIMV, but not HE, had similar effects on the liver/body weight ratio (F(6, 32) = 31.69; p < 0.005) when compared with groups C and N (), indicating that QRT and SIMV attenuated the effects induced by the high-cholesterol diet. This parameter is predictive to detect potentially harmful effects on liver status (Bailey et al., Citation2004; Lin et al., Citation2008).
Table 1. The liver weight/body weight ratios of rats subjected to a hyperlipidemic diet (mean ± SD) (n = 6).
Effects of HE extracts of S. chilensis and quercetrin on serum lipid profiles
At the end the 4-week treatment, group C exhibited higher levels of TC, LDL-C, and TG in serum than group N (p < 0.0001). All hyperlipemic rats treated with HE (150, 300, and 600), quercetrin (group QRT), and simvastatin (group SIMV) exhibited an accentuated decrease in the levels of TC (−19.9%, −27.5%, −31.0%, −39.4%, and −34.2%, respectively), LDL-C (−36.0%, −37.5%, −43.3%, −59.4%, and −44.2%, respectively), and TG (−15.6%, −23.5%, −29.8%, −27.2%, and −32.4%, respectively) compared with group C (p < 0.005; ). The atherogenic index (CT/HDL-C) was increased in group C and decreased in the other groups (F(6, 39) = 28; p < 0.0001), whereas for VLDL levels, only the HE 150 group did not prevented the increase induced by the high cholesterol diet (F(6, 39) = 8.6; p < 0.005). There was no difference in serum ALT enzyme activity between the groups ().
Figure 1. The effects of treatments on TC, LDL-C, and TG values (mean ± SD) (n = 6). Rats were either given a normal diet (N) or the following treatments with a high-fat diet: water (C); 150, 300, and 600 mg/kg Solidago chilensis hydroalcoholic extracts (150, 300, and 600); quercetrin (QRT); and simvastatin (4 mg/kg) (SIMV). ANOVA one-way *p < 0.0001 compared with the group N. #p < 0.005 compared with the group C.
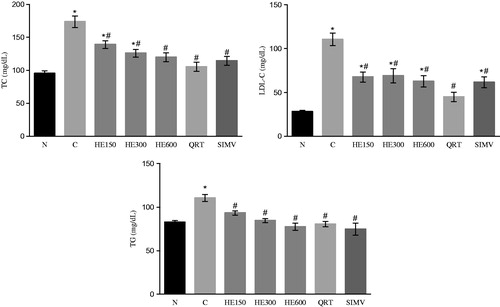
Table 2. The effects of the treatments on atherogenic index values, VLDL, and ALT enzymatic activity (n = 6) (mean ± SD).
Effects of Solidago chilensis extracts and quercetrin on HMG-CoA reductase activity
To propose a possible mechanism of the hypolipidemic effects of HE and QRT, we measured reductase activity of hepatic HMG-CoA, which is the regulatory enzyme in cholesterol biosynthesis. The analysis of enzymatic activity revealed a significant decrease in HMG-CoA activity in the groups treated with HE (150, 300, and 600 mg/kg; reductions of 4.20, 4.70, and 4.80, respectively) or QRT (reduction of 4.80) compared with rats fed with the high-fat diet and water (group C; F(6, 39) = 148; p < 0.005; reduction of 3.00; ). These effects were similar to those of group SIMV, which received simvastatin, a hypolipidemic drug known to be an HMG-CoA inhibitor.
Figure 2. Index of inhibition of activity HMG-CoA reductase of the animals (mean values ± SD) (n = 6). Rats were either given a normal diet (N) or the following treatments with a high-fat diet: water (C); 150, 300, and 600 mg/kg Solidago chilensis hydroalcoholic extracts (150, 300, and 600); quercetrin (QRT); and simvastatin (4 mg/kg) (SIMV). *p < 0.005 compared with the group N. #p < 0.005 compared with the group C.
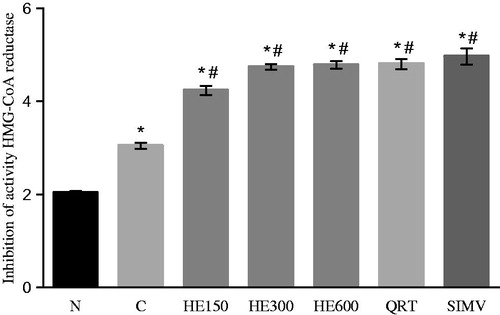
Fecal excretion of cholesterol
To determine whether HE and quercetrin could interfere with the excretion of cholesterol, fecal cholesterol levels were measured. Observed fecal cholesterol levels differed between the experimental groups (F(6, 39) = 176.4; p < 0.0001) and the N group. In cholesterol-fed rats, all groups exhibited significantly higher cholesterol excretion values than group C (p < 0.0001). The highest excretion value was observed in the HE 600 group and was significantly higher than that of the HE 150, HE 300, and QRT groups (p < 0.0001; ).
Figure 3. The effects of the treatments on cholesterol excretion in feces (data shown represent mean values ± SD) (n = 6). Rats were either given a normal diet (N) or the following treatments with a high-fat diet: water (C); 150, 300, and 600 mg/kg Solidago chilensis hydroalcoholic extracts (150, 300, and 600); quercetrin (QRT); and simvastatin (4 mg/kg) (SIMV). *p < 0.0001 compared with the group N. #p < 0.0001 compared with the group C. &p < 0.0001 compared with the groups HE 150, HE 300 and QRT.
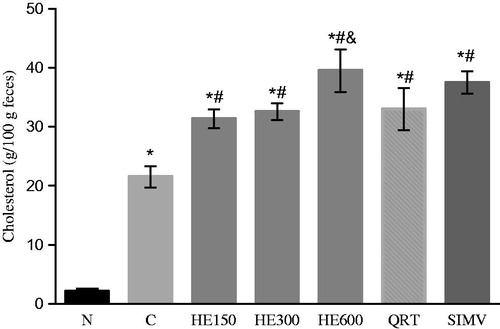
Effects of S. chilensis extracts and quercetrin on lipid peroxidation and antioxidant enzyme activity in the liver
Hepatic lipid peroxidation and antioxidant enzymes were evaluated to determine whether HE and quercetrin could protect the liver against possible changes associated with oxidative stress induced by the high-cholesterol diet. Unexpectedly, TBARS levels decreased in the rat that received the cholesterol-enriched diet when compared with group N, and neither HE nor QRT showed any effect on this parameter (p = 0.0016; ). In addition to the lowering effect of the high-cholesterol diet on TBARS levels, this treatment showed no effect on antioxidant enzyme activity, suggesting that the cholesterol-enriched diet did not trigger oxidative stress in the liver (data not shown).
Figure 4. The effects of the treatments on liver lipid peroxidation (data shown represent mean values ± SD) (n = 6). Rats were either given a normal diet (N) or the following treatments with a high-fat diet: water (C); 150, 300, and 600 mg/kg Solidago chilensis hydroalcoholic extracts (150, 300, and 600); quercetrin (QRT); and simvastatin (4 mg/kg) (SIMV). *p = 0.0016 compared with the N group.
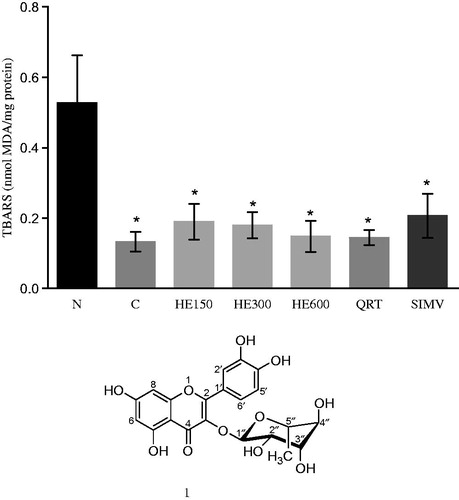
Discussion
This is the first report on the hypolipidemic effect of S. chilensis in cholesterol-fed rats. The present results showed that a cholesterol-enriched diet caused an increase in serum lipid levels (TC, TG, LDL-C, and VLDL), which was mitigated by all doses of HE of S. chilensis. The TC and TG lowering effects were dose related (r = −0.494, p < 0.05 and r = −0.677, p < 0.01, respectively, by Pearson’s correlation analysis). Because the lipid-lowering effects exerted by HE were similar to those of isolated quercetrin, this flavonoid possibly played a key role in the decrease of serum lipid levels observed for all doses of this extract. Both HE and quercetrin lowered serum-TG levels, similar to the simvastatin group. A previous study using other plants suggested that these effects are attributed to the restoration of TG catabolism by stimulating lipolytic pathways involving plasma lipoprotein lipase (LPL) (Xie et al., Citation2007). Moreover, quercetin can reduce the activities and mRNA levels of several enzymes involved in the synthesis of fatty acids (Odbayar et al., Citation2006). Similar effects may have occurred with S. chilensis HE and quercetrin used in this study.
Hypercholesterolemia and elevated levels of LDL-C are the major risk factors involved in the pathogenesis of cardiovascular diseases, including atherosclerosis (Dai et al., Citation2013; Lee et al., Citation2010). The atherogenic index, defined as the TC/HDL-C ratio, has been considered as an indicator of the risk of atherosclerosis development (Silva, Citation2002; Xia et al., Citation2011). In the present study, the increase in atherogenic index in cholesterol-fed rats was significantly reduced by all treatments, suggesting that S. chilensis HE and quercetrin are potential candidates for the therapy of hypercholesterolemia-induced atherosclerosis.
Increased serum lipid levels were accompanied by liver weight gain in cholesterol-fed rats, and this effect was attenuated by all treatments, of which quercetrin and simvastatin exerted the greatest effects. A cholesterol-enriched diet induces the accumulation of cholesterol in the liver, possibly accounting for the increased liver weight (Chen et al., Citation2012; Felmlee et al., Citation2009). The attenuation of liver-weight gain by all doses of HE and quercetrin may be associated with a decrease in the hepatic production (as suggested by dose-dependent effects of HE on the HMG-CoA/mevalonate ratio; r = 0.726, p < 0.01 by Pearson’s correlation analysis) and/or an increase in fecal excretion of cholesterol. Effects of HE were dose dependent (r = 0.786, p < 0.01 by Pearson’s correlation analysis).
We cannot discard the possibility that S. chilensis HE and quercetrin also interfere with the cholesterol metabolic cycle at other points, such as intestinal uptake, endogenous metabolism, and transport by lipoproteins (Bei et al., Citation2012), which were not assessed in this study.
A high-cholesterol diet with cholic acid led to liver steatosis, inflammation, and fibrosis in a previous study (Jeong et al., Citation2005). Our results showed that despite the increased liver weight resulting from the cholesterol-enriched diet, it was unlikely to induce hepatic damage as evidenced by the lack of change in serum levels of ALT, a useful indicator of hepatic function (Kew, Citation2000). In fact, liver TBARS levels were lower and antioxidant enzyme activity remained unchanged in cholesterol-fed rats compared with group N, even though oxidative stress is reportedly involved in hepatic damage that is associated with a high-cholesterol diet in rats (Attia et al., Citation2010; Balkan et al., Citation2004). Nevertheless, these results are in agreement with those of Yuan and Kitts (Citation2003), which showed that a cholesterol-enriched (5.0 g/kg) diet reduced susceptibility to lipid peroxidation in red blood cells and the liver of rats. This effect of lowering TBARS levels has been attributed to the protective antioxidant and stabilizing effects of enhanced membrane cholesterol against oxidative stress, possibly by the interception of peroxyl radicals produced during lipid peroxidation (Girao et al., Citation1999; Yuan & Kitts, Citation2002). Moreover, bile acids including cholate have shown in vitro antioxidant properties by inhibiting peroxidations of polyunsaturated lipids, linoleic acid, and the highly fluorescent protein phycoerythrin. This activity resulted from the scavenging activity of peroxyl radicals by the direct oxidation of bile acids (DeLange & Glazer, Citation1990).
Because oxidative stress in the liver is unlikely to have occurred in this study, verifying in vivo antioxidant activity of S. chilensis was impossible. However, free-radical scavenging properties of S. chilensis, as well as those of isolated quercetrin, were observed in the DPPH assay. Further studies are required to confirm the in vivo antioxidant effects of S. chilensis and its benefits in hypercholesterolemic animal models.
In summary, our results showed that S. chilensis HE exerted marked hypolipidemic effects on cholesterol-fed rats, possibly by the reduction of HMG-CoA reductase activity and an increased fecal excretion of cholesterol. Furthermore, there was strong evidence that quercetrin, the major constituent of S. chilensis extracts, is largely responsible for the observed biological activities. However, the underlying mechanisms of these effects need to be elucidated by further studies.
Conclusions
Solidago chilensis, “arnica-do-brasil”, and its main constituent, quercetrin, may be effective cholesterol-lowering agents. They can be effective in the prevention of hypercholesterolemia and possible protection against atherosclerosis.
Declaration of interest
The authors declare that they have no conflicts of interest. CAMS thanks CNPq for a research fellowship (Proc. no. 311787/2012-6).
References
- Adeneye AA, Adeyemi OO, Agbaje EO. (2010). Anti-obesity and antihyperlipidaemic effect of Hunteria umbellata seed extract in experimental hyperlipidaemia. J Ethnopharmacol 130:307–14
- Aebi H. (1984). Catalase in vitro. Meth Enzymol 105:121–6
- Apáti P, Houghton PJ, Kite G, et al. (2006). In-vitro effect of flavonoids from Solidago canadensis extract on glutathione-S-transferase. J Pharm Pharmacol 58:251–6
- Attia A, Ragheb A, Sylwestrowicz T, Shoker A. (2010). Attenuation of high cholesterol-induced oxidative stress in rabbit liver thymoquinone. Eur J Gastroenterol Hepatol 22:826–34
- Bailey SA, Zidell RH, Perry RW. (2004). Relationships between organ weight and body/brain weight in the rat: What is the best analytical endpoint? Toxicol Pathol 32:448–66.
- Balkan J, Dogru-Abbasoglu S, Aykaç-Toker G, Uysal M. (2004). The effect of a high cholesterol diet on lipids and oxidative stress in plasma, liver and aorta of rabbits and rats. Nutr Res 24:229–34
- Balzan S, Hernandes A, Reichert CL, et al. (2013). Lipid-lowering effects of standardized extracts of Ilex paraguariensis in high-fat-diet rats. Fitoterapia 86:115–22
- Bao L, Bai S, Borijihan G. (2012). Hypolipidemic effects of a new piperine derivative GB-N from Piper longum in high-fat diet-fed rats. Pharm Biol 50:962–7
- Bei WJ, Guo J, Wu HY, Cao Y. (2012). Lipid-regulating effect of traditional Chinese medicine: Mechanisms of actions. Evid Based Complement Alternat Med 2012:970635
- Brand-Williams W, Cuvelier ME, Berset C. (1995). Use of a free-radical method to evaluate antioxidante activity. Food Sci Technol-Lebens Wissens Technol 28:25–30
- Brunt EM. (2004). Nonalcoholic steatohepatitis. Semin Liver Dis 24:3–20
- Chen Y, Chen Y, Zhao L, et al. (2012). Inflammatory stress exacerbates hepatic cholesterol accumulation via disrupting cellular cholesterol export. J Gastroenterol Hepatol 27:974–84
- Correia SJ, David JM, Silva EP, et al. (2008). Flavonoides, norisoprenoides e outros terpenos das folhas de Tapirira guianensis. Quim Nova 31:2056–9
- Da Silva AG, De Souza CPG, Koehler J, et al. (2010). Evaluation of an extract of Brazilian arnica (Solidago chilensis Meyen, Asteraceae) in treating lumbago. Phytother Res 24:283–7
- Dai FJ, Hsu WH, Huang JJ, Wu SC. (2013). Effect of pigeon pea (Cajamus cajan L.) on high-fat diet-induced hypercholesterolemia in hamsters. Food Chem Toxicol 53:384–93
- DeLange RJ, Glazer AN. (1990). Bile acids: Antioxidants or enhancers of peroxidation depending on lipid concentration. Arch Biochem Biophys 276:19–25
- Diehl KH, Hull R, Morton D, et al. (2001). A good practice guide to the administration of substances and removal of blood, including routes and volumes. J Appl Toxicol 21:15–23
- Facury Neto MA, Fagundes DJ, Beletti ME, et al. (2004). Systemic use of Solidago microglossa DC in the cicatrization of open cutaneous wounds in rats. Braz J Morphol Sci 21:207–10
- Felmlee MA, Woo G, Simko E, et al. (2009). Effects of the flaxseed lignans secoisolariciresinol diglucoside and its aglycone on serum and hepatic lipids in hyperlipidaemic rats. Br J Nutr 102:361–9
- Folch J, Lees M, Sloane Stanley GH. (1957). A simple method for the isolation and purification of total lipids from animal tissues. J Biol Chem 226:497–509
- Friedewald WT. (1972). Estimation of concentration of low-density lipoprotein cholesterol in plasma, without use of the preparative ultracentrifuge. Clin Chem 18:499–502
- Girao H, Mota C, Pereira P. (1999). Cholesterol may act as an antioxidant in lens membranes. Curr Eye Res 18:448–54
- Holmgren A, Björnstedt M. (1995). Thioredoxin and thioredoxin reductase. Meth Enzymol 252:199–208
- Istivan E. (2003). Statin inhibition of HMG-CoA reductase: A 3-dimensional view. Atheroscler Suppl 4:3–8
- Jeong WI, Jeong DH, Do SH, et al. (2005). Mild hepatic fibrosis in cholesterol and sodium cholate diet-fed rats. J Vet Med Sci 67:235–42
- Kew MC. (2000). Serum aminotransferase concentration as evidence of hepatocellular damage. Lancet 355:591–2
- Kin HY, Jeong DM, Jung HJ, et al. (2008). Hypolipidemic effects of Sophora flavescens and its constituents in polaxamer 407-induced hyperlipidemic and cholesterol-fed rats. Biol Pharm Bull 31:73–8
- Lee JS, Bok SH, Jeon SM, et al. (2010). Antihyperlipidemic effects of Buckwheat leaf and flower in rats fed a high-fat diet. Food Chem 119:235–40
- Li W, Zhang M, Gu J, et al. (2012). Hypoglycemic effect of protopanaxadiol-type ginsenosides and compound K on Type 2 diabetes mice induced by high-fat diet combining with Streptozotocin via suppression of hepatic gluconeogenesis. Fitoterapia 83:192–8
- Lin LY, Peng CC, Liang YJ, et al. (2008). Alpinia zerumbet potentially elevates high-density lipoprotein cholesterol level in hamsters. J Agric Food Chem 56:4435–43
- Lorenzi H, Matos FJA. (2002). Plantas Medicinais do Brasil: Nativas e Exóticas. São Paulo: Instituto Plantarum de Estudos da Flora
- Misra HP, Fridovich I. (1972). The role of superoxide anion in the autooxidation of epinephrine and a simple assay for superoxide dismutase. J Biol Chem 247:3170–5
- Nabi SA, Kasetti RB, Sirasanagandla S, et al. (2013). Antidiabetic and antihyperlipidemic activity of Piper longum root aqueous in STZ induced diabetic rats. BMC Complement Altern Med 13:1–9
- Odbayar TO, Badamhand D, Kimura T, et al. (2006). Comparative studies of some phenolic compounds (quercetin, rutin, and ferulic acid) affecting hepatic fatty acid synthesis in mice. J Agric Food Chem 54:8261–5
- Ohkawa H, Ohishi N, Yagi K. (1979). Assay for lipid peroxides in animal tissues by thiobarbituric acid reaction. Anal Biochem 95:351–8
- Omata Y, Folan M, Shaw M, et al. (2006). Sublethal concentrations of diverse gold compounds inhibit mammalian cytosolic thioredoxin reductase (TrxR1). Toxicol In Vitro 20:882–90
- Paglia DE, Valentine WN. (1967). Studies on the quantitative and qualitative characterization of erythrocyte glutathione peroxidase. J Lab Clin Med 70:158–69
- Pankaj GJ, Patil SD, Haswani NG, et al. (2010). Hypolipidemic activity of Moringa oleifera Lam., Moringaceae, on high fat diet induced hyperlipidemia in albino rats. Rev Bras Farmacogn 20:969–73
- Patel RP, Baria AH, Patel NA. (2008). An overview of size reduction technologies in the field of pharmaceutical manufacturing. Asian J Pharm 2:216–20
- Park JB, Velasquez MT. (2012). Potential effects of lignan-enriched flaxseed powder on bodyweight, visceral fat, lipid profile and blood pressure in rats. Fitoterapia 83:941–6
- Raida K, Nizar A, Barakat S. (2008). The effect de Crataegus aronica aqueous extract in rabbits fed with high cholesterol diet. Eur J Sci Res 22:352–60
- Rao AV, Ramakrishnan S. (1975). Indirect assessment of hydroxymethylglutaril-CoA reductase (NADPH) activity in liver tissue. Clin Chem 21:1523–5
- Schemeda-Hirchmann G, Rodrigues J, Astudillo L. (2002). Gastroprotective activity of the diterpene solidagenone and its derivates on experimentally induced gastric lesions in mice. J Ethnopharmacol 81:111–15
- Silva JM. (2002). Statins, high-density lipoprotein and the low density lipoprotein/high density lipoprotein ratio. Am J Cardiol 86:593–4
- Soares-Valverde SS, Azevedo-Silva RC, Tomassini TCB. (2009). Utilização de CLAE, como paradigma na obtenção e controle do diterpeno solidagenona a partir de inflorescências de Solidago chilensis Meyen (arnica brasileira). Rev Bras Farm 9:196–9
- Tabas I. (2002). Consequences of cellular cholesterol accumulation: Basic concepts and physiological implications. J Clin Invest 110:905–11
- Tamura EK, Jimenes JK, Waismam K, et al. (2009). Inhibitory effects of Solidago chilensis Meyen hydroalcoholic extract on acute inflammation. J Ethnopharmacol 122:478–85
- Tiberti LA, Yariwake JA, Ndjoko K, Hosttetmann K. (2007). On-Line LC/UV/MS analysis in three aplle varieties most widely cultivated in Brazil. J Braz Chem Soc 18:100–5
- Torres LMB, Akisue MK, Roque NF. (1987). Quercetrina em Solidago microglossa DC, a arnica do Brasil. Rev Farm Bioquím Univ S Paulo 23:33–40
- Trovato A, Monforte MT, Barbera R, et al. (1996). Effects of fruit juices of Citrus sinensis L. and Citrus limon L. on experimental hypercholesterolemia in the rat. Phytomedicine 2:221–7
- Vilkhu K, Mawson R, Simons L, Bates D. (2008). Applications and opportunities for ultrasound assisted extraction in the food industry – A review. Innov Food Sci Emerg Technol 9:161–9
- Yuan YV, Kitts DD. (2002). Dietary fat source and cholesterol interactions alter plasma lipids and tissue susceptibility to oxidation in spontaneously hypertensive (SHR) and normotensive Wistar Kyoto (WKY) rats. Mol Cell Biochem 232:33–47
- Yuan YV, Kitts DD. (2003). Dietary (n-3) fat and cholesterol alter tissue antioxidant enzymes and susceptibility to oxidation in SHR and WKY Rats. J Nutr 133:679–88
- Wang L, Weller CL. (2006). Recent advances in extraction of nutraceuticals from plants. Trends Food Sci Tech 17:300–12
- Webster D, Taschereau P, Belland RJ, et al. (2008). Antifungical activity of medicinal plant extracts: Preliminary screening studies. J Ethnopharmacol 115:140–6
- Xia W, Sun C, Zhao Y, Wu L. (2011). Hypolipidemic e antioxidant activities of Sanchi (Radix notoginseng) in rats with a high fat diet. Phytomedicine 18:516–20
- Xie W, Wang W, Su H, et al. (2007). Hypolipidemic mechanisms of Ananas comosus L. leaves in mice: Different from fibrates but similar of statins. J Pharmacol Sci 103:267–74