Abstract
Context: Mentha piperita L. (Lamiaceae) has been used in folk medicine since antiquity. Its essential oil (mint EO) and major bioactive components have antimicrobial properties but their mechanism of action is still not clear.
Objective: The present work aims to elucidate M. piperita’s anti-Candida activity and mode of action.
Materials and methods: Chemical constituents of mint EO were identified by GC-MS by injecting 0.1 ml sample in a splitless mode. MIC was determined by the broth dilution method. Synergy with fluconazole (FLC) was evaluated by checkerboard assay and FICI. Mid log phase cells harvested from YPD media were used for proton extrusion measurement and the rate of glucose-induced H+ efflux gives PM-ATPase activity. Cell membrane integrity was estimated by total ergosterol content and scanning microscopy at respective MIC and sub-MIC values. In vitro hemolytic activity was performed to rule out possible cytotoxicity of the test compounds.
Results: The MIC value of mint EO, carvone, menthol, and menthone was 225, 248, 500, and 4200 µg/ml, respectively. At their respective MICs, these compounds showed 47, 42, 35, and 29% decrease in PM-ATPase activity besides showing synergy with FLC. In case of FLC-resistant strains, the decrease in H+ efflux was by 52, 48, 32, and 30%, a trend similar to the susceptible cases. Exposed Candida cells showed a 100% decrease in the ergosterol content, cell membrane breakage, and alterations in morphology.
Discussion and conclusion: Our studies suggest that mint EO and its lead compounds exert antifungal activity by reducing ergosterol levels, inhibiting PM-ATPase leading to intracellular acidification, and ultimately cell death. Our results suggest that mint EO and its constituents are potential antifungal agents and need to be further investigated.
Introduction
Infections caused by opportunistic fungal pathogens have become increasingly common in immunocompromised patients. Candida albicans is the predominant organism associated with candidiasis; but other Candida species, including Candida glabrata, Candida parapsilosis, and Candida krusei, are now emerging as serious hospital-acquired infections (Maschmeyer, Citation2006; Pfaller & Diekema, Citation2004). Available antifungal drugs can treat superficial fungal infections, but only a few treatments are available for invasive diseases. Polyenes cause serious host toxicity (Cohen, Citation1998) whereas azoles are fungistatic and their prolonged use leads to drug resistance (Sanglard et al., Citation2003). Due to a dramatic rise in fungal infections and the current trend toward increasing awareness in traditional medicine, plant-derived antifungal compounds are attracting much interest as natural alternatives owing to their versatile applications (Ahmad et al., Citation2010b; Iwu et al., Citation1999).
Essential oils and their major components have been explored for antimicrobial properties (Matasyoh et al., Citation2009). Mentha piperita L. (Lamiaceae) essential oil (mint EO) has been used in traditional medicine and its biological activity may be due to its major components, carvone, menthol, and menthone (). Recent studies have shown that it inhibits the formation of Candida and other microbial biofilms (Carretto et al., Citation2010; Derwich et al., Citation2011; Saharkhiz et al., Citation2012; Sandasi et al., Citation2011), decreasing the production of virulence-associated exoproteins by Staphylococcus aureus at sub-inhibitory concentrations in a dose-dependent manner (Li et al., Citation2011).
The fungal plasma membrane H+ ATPase is a predominant membrane protein that belongs to P-type ATPase family of ion translocating ATPases (Perlin et al., Citation2006; Set-Young et al., Citation1997). These include Na+ K+-ATPase and H+ K+-ATPase, which are molecular targets for several clinically important therapeutics (Yatime et al., Citation2009). H+ ATPase plays a crucial role in fungal cell physiology as it maintains electrochemical proton gradient across cell membranes necessary for nutrient uptake. It regulates intracellular pH, cell growth, and has been implicated in the pathogenicity of fungi through its effects on dimorphism, nutrient uptake, and medium acidification (Manzoor et al., Citation2002; Set-Young et al., Citation1997). The enzyme activity and H+ efflux are regulated by some nutrients, most notably by glucose (Serrano, Citation1983). Plasma membrane H+ ATPase is unique and crucial to fungal cells and hence is a promising antifungal target. It will help in the development of new mechanism-based drugs.
The present work investigates the anti-Candida activity of mint EO and its lead molecules carvone, menthol, and menthone against five Candida strains; C. albicans (ATCC 10261, ATCC 44829, and ATCC 90028), C. tropicalis (ATCC 750), and C. glabrata (ATCC 90030). The fungicidal efficacy of these compounds was evaluated by studying the effect of growth and virulence of Candida. The antifungal mode of action of mint EO and its lead molecules was further elucidated by determining their effect on PM-ATPase-mediated H+ efflux, ergosterol biosynthesis, and the ultrastructure of Candida cells using scanning electron microscopy (SEM).
Materials and methods
Strains and media
Three Candida species were used in the present study: C. albicans (ATCC 10261, ATCC 44829, and ATCC 90028), C. tropicalis (ATCC 750), and C. glabrata (ATCC 90030). All the strains were grown on the YPD medium containing 1% yeast extract, 2% peptone, and 2% dextrose (w/v) (Hi Media, Mumbai, India). YPD agar plates containing 2.5% agar in addition were used to maintain the culture. Extra pure grade of mint EO (ISO 9001:2008) extracted from Mentha piperita was purchased from Mohan Perfumery Co. (Delhi, India), and stored at 4 °C. Carvone, menthol, and menthone were purchased from SAFC (St. Louis, MO), Aldrich (Munich, Germany) and MP Biomedicals (Santa Ana, CA), respectively, whereas all inorganic chemicals were of analytical grade and procured from E. Merck (Mumbai, India). Fluconazole (FLC) was purchased from HiMedia (Mumbai, India).
Minimum inhibitory and fungicidal concentrations
The MIC values was defined as the lowest concentration of the oil/test molecules that caused inhibition of visible growth of Candida cells and was determined in vitro in the liquid medium by the broth dilution method as described by Clinical and Laboratory Standards Institute (CLSI, Citation2008). To determine the MFC values after reading the corresponding MIC values, 20 ml samples from all optically clear tubes (complete growth inhibition) plus the last tube showing growth were sub-cultured on YEPD agar plates. The plates were incubated at 35 °C for a minimum of 3 d, until growth was clearly visible in the control samples, and MFC values were determined as the lowest concentration of the mint EO and its three major components for which there was no visible growth.
Checkerboard assay (FIC index, FICI)
Checkerboard synergy testing was performed as reported earlier (Ahmad et al., Citation2010a). Fractional inhibitory concentrations (FIC) were calculated as follows: FIC of drug A = MIC of drug A in combination/MIC of drug A alone; FIC of drug B = MIC of drug B in combination/MIC of drug B alone. FICI was defined as the sum of FIC of drug A and FIC of drug B and was interpreted as follows: synergy, FICI ≤ 0.5; antagonism, FICI > 4.0; and indifferent, 0.5 < FICI < 4.0.
GC-MS analysis
Mint EO was analyzed by GC-MS as described previously (Khan et al., Citation2010a). The chemical components of oil were identified by comparing the retention times of the chromatographic peaks with those of authentic compounds using the WILEY8.LIB and NIST05s.LIB.
Proton extrusion measurement
The proton pumping activity of Candida cells was determined as described previously (Ahmad et al., Citation2010c; Manzoor et al., Citation2002). Briefly, mid-log phase cells harvested from the YPD medium were washed twice with distilled water and routinely 100 mg cells were suspended in 5 ml solution containing 0.1 M KCl, 0.1 mM CaCl2 in distilled water. Suspension was kept in a double-jacketed glass container with constant stirring. The container was connected to a water circulator at 25 °C. Initial pH was adjusted to 7.0 using 0.01 M HCl/NaOH. Test compounds were added to achieve the desired concentrations (MIC90) in 5 ml solution. For glucose stimulation experiments, 100 μl of glucose was added to achieve a final concentration of 5 mM in total volume. H+ extrusion rate was calculated from the volume of 0.01 N NaOH consumed. The experiment was also performed with 5 mM vanadate (a potent inhibitor of the H+ ATPase) and 5 µg/ml FLC used as the standard antifungal drug.
Sterol quantification method
The total intracellular sterols were extracted as reported earlier with slight modifications (Ahmad et al, Citation2011; Arthington-Skaggs et al., Citation1999). Briefly, a single Candida colony from an overnight sabouraud dextrose agar plate culture was used to inoculate 50 ml of sabouraud dextrose broth (HiMedia, Mumbai, India) containing 0.056, 0.112, and 0.225 mg/ml of mint EO; 0.062, 0.124, and 0.248 mg/ml of carvone; 0.125, 0.25, and 0.5 mg/ml of menthol and 2.1, 4.2, and 8.4 mg/ml of menthone along with a positive control (FLC). The cultures were incubated for 16 h with shaking at 35 °C. The stationary-phase cells were harvested by centrifugation at 2700 rpm (Sigma 3K30, Sigma, St. Louis, MO) for 5 min and washed once with sterile distilled water. The net wet weight of the cell pellet was determined. Three milliliters of 25% alcoholic potassium hydroxide solution (25 g of KOH and 35 ml of sterile distilled water, brought to 100 ml with 100% ethanol) was added to each pellet and vortex mixed for 1 min. Cell suspensions were transferred to 16 × 100 mm sterile borosilicate glass screw-cap tubes and were incubated in an 85 °C water bath for 1 h. Following incubation, tubes were allowed to cool to room temperature. Sterols were then extracted by the addition of a mixture of 1 ml of sterile distilled water and 3 ml of n-heptane followed by vigorous vortex mixing for 3 min. The heptane layer was transferred to a clean borosilicate glass screw-cap tube and stored at −20 °C for as long as 24 h. Prior to analysis, a 20 µl aliquot of sterol extract was diluted five-fold in 100% ethanol and scanned between 240 nm and 300 nm using Systronics UV–Vis 117 spectrophotometer (Systronics 117, Mumbai, India). The presence of ergosterol and the late sterol intermediate 24 (28) DHE in the extracted sample resulted in a characteristic four-peaked curve (). A dose-dependent decrease in the height of the absorbance peaks was evident and corresponded to decreased ergosterol concentration. The absence of detectable ergosterol in extracts was indicated by a flat line. The ergosterol content was calculated as percentage of wet weight of the cell by the following equations:
where F is the factor for dilution in ethanol and 290 and 518 are the E values (in percentages per centimeter) determined for crystalline ergosterol and 24 (28) DHE, respectively. This experiment was performed on FLC-sensitive and FLC-resistant strains. The values are shown in terms of the mean ± STD of all three respective categories.
Figure 2. UV spectrophotometric sterol profiles of representative fluconazole-susceptible (A) and fluconazole-resistant (B) Candida strains. Strains were grown for 16 h in Sabouraud dextrose broth containing MIC/4, MIC/2, and MIC values of mint EO (curves c, d, and e); carvone (curves f, g, and h); menthol (curves i, j, and k), and menthone (curves l, m, and n), respectively. Curve ‘a’ is control (untreated cells) while curve ‘b’ shows positive control (FLC). Sterols were extracted from the cells and spectral profiles between 240 and 300 nm were determined.
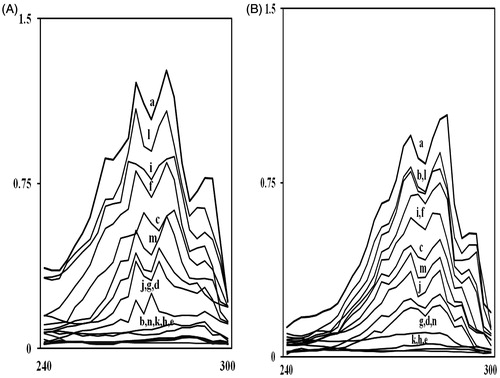
Scanning electron microscopy
Candida cell suspensions from overnight cultures were prepared in YPD medium. Test compound, at the MIC value, was added to the cell culture (∼1 × 106) and incubated for 14 h at 30 °C and prepared for electron microscopy. All Candida cells were fixed with 2% glutaraldehyde in 0.1% phosphate buffer for 1 h at 20 °C (Kaneshima et al., Citation1977; Mares, Citation1989). Cells were washed with 0.1 M phosphate buffer (pH 7.2) and post fixed with 1% OsO4 in 0.1 M phosphate buffer for 1 h at 4 °C. Samples were dehydrated in acetone and dropped on round glass cover slip with HMDS and dried at room temperature followed by sputter coating with gold and observed under the electron microscope (Zeiss EV040, Carl Zeiss Meditec Inc., Dublin, CA).
Hemolytic activity
Hemolytic activity of mint EO and its lead compounds on human red blood cells was performed in vitro as described earlier (Ahmad et al., Citation2011). Human erythrocytes from healthy individuals were collected in tubes containing EDTA as an anti-coagulant. The erythrocytes were harvested by centrifugation for 10 min at 2000 rpm at 20 °C, and washed three times in PBS. The pellet was suspended in PBS to yield 10% (v/v) erythrocyte/PBS suspension. The 10% suspension was then diluted 1:10 in PBS. From each suspension, 100 µl was added in triplicate to 100 µl of a different dilution series of test compounds (and FLC) in the same buffer in eppendorf tubes. Total hemolysis was achieved with 1% Triton X-100. The tubes were incubated for 1 h at 37 °C and then centrifuged for 10 min at 2000 rpm at 20 °C. From the supernatant fluid, 150 µl was transferred to a flat-bottomed microtiter plate (BIO-RAD, iMark, Hercules, CA), and the absorbance was measured spectrophotometrically at 450 nm. Hemolysis percentage was calculated by following equation:
Results
Antifungal activity of mint EO
The test compounds were found to be active against all the strains tested. The MIC value was defined as the concentration required to reduce 90% cell growth. MIC and MFC values of mint EO against all the Candida strains used in the present study were 225 µg/ml. The MIC of carvone was 248 µg/ml for all the strains except for C. glabrata ATCC 90030 (497 µg/ml) while the MFC value was 2 MIC. Menthol gave an MIC value of 500 µg/ml and an MFC value of 1000 µg/ml (2 MIC). Menthone was the weaker antifungal giving an MIC of 4.2–8.4 mg/ml and an MFC double that of its MIC. Mint EO and carvone were the most effective with low MIC and MFC values. No systematic differences were observed between FLC sensitive and resistant strains.
GC-MS analysis
Mint EO was characterized by a detailed GC-MS analysis and the results are shown in . Thirty-three compounds were identified representing 100% oil. The oil was characterized by high amounts of menthol (34.82%), carvone (19.54%), and menthone (9.10%) as the main components.
Table 1. GC-MS analysis of mint essential oil.
Proton extrusion measurements
The plasma membrane H+ ATPase regulates intracellular pH (Stewart et al., Citation1988), maintains ion balance, and generates the electrochemical proton gradient crucial for nutrient uptake and morphogenesis in fungal cells. The inhibition of its activity correlates with the cessation of cell growth. Hence PM-ATPase-mediated H+ efflux in C. albicans was determined in the presence of mint EO and its lead molecules. gives relative rates of H+ efflux by Candida cells in the presence of MIC of mint EO, carvone, menthol, and menthone. The effect of FLC (5 µg/ml) on H+ efflux was also studied. The inhibitory effect of mint EO on the rate of H+ efflux was determined in nmoles/min/mg of Candida cells. The control (cells only) showed H+ efflux rate of 5.1–5.5 nmoles/min/mg yeast cells. Mint EO, carvone, menthol, and menthone showed about 47, 42, 35, and 29% inhibition, respectively. In the case of FLC-resistant strains, the decrease in efflux was by 52, 48, 36, and 30%, respectively, when treated with the same compounds, a trend similar in the susceptible cases. In the presence of 5 mM glucose, H+ efflux was enhanced by 4.2-fold in susceptible cells and two-fold in resistant cells. This is in conformity with previous observations (Manzoor et al., Citation2002; Serrano, Citation1983). Inhibition of glucose induced H+ efflux by mint EO in standard and resistant Candida strains was 44 and 38%, respectively, with respect to control at pH 7.0. The values were 35 and 35.5% in the presence of carvone; 34 and 32% in the presence of menthol, and 24 and 23% in the presence of menthone, respectively. The above inhibition studies were done at respective MIC values of mint EO and its main constituents. Experiments were also performed at ½ MIC values but data are not shown as the results were similar to that of the control. Also, H+ extrusion in every case was inhibited by 100% following the addition of 5 mM orthovandate (positive control), a specific inhibitor of H+-ATPase, suggesting it to be the major agency responsible for H+ extrusion whereas FLC did not have a significant effect on the acidification of the extracellular medium.
Table 2. Effect of mint essential oil and its lead compounds on the rate of H+-efflux by Candida cells at pH 7.0: Cells were suspended in 0.1 mM CaCl2 and 0.1 M KCl at 25 °C.
Sterol quantification method
Ergosterol is specific to fungi and is the major sterol component of the fungal cell membranes, being responsible for maintaining cell function and integrity. The primary mechanism of action by which azole antifungal drugs inhibit fungal cell growth is the disruption of normal sterol biosynthetic pathways, leading to a reduction in ergosterol biosynthesis. shows UV spectrophotometric sterol profiles of standard and FLC-resistant Candida strains when grown in SD broth for 16 h in the presence of MIC and sub-MIC values of mint EO, carvone, menthol, and menthone. Their effect on ergosterol biosynthesis in both FLC-sensitive and resistant Candida strains is summarized in . A dose-dependent decrease in ergosterol synthesis was observed when the yeast cells were grown in the presence of test compounds. The total ergosterol content in Candida cells grown with mint EO and its bioactive lead molecules at their respective MIC values showed a 100% decrease in all the strains used in the present study. Cells grown in FLC (8 μg/ml) also showed 100% decrease in susceptible cells which inhibit ergosterol synthesis by interacting with lanosterol 14α-demethylase. As expected in the case of FLC-resistant strains, the inhibition observed was only 16% when cells were exposed to 64 µg/ml FLC (MIC of resistant strains). It was encouraging to see that mint EO and its lead molecules inhibited the ergosterol content in resistant strains to the same extent. At sub-MIC values of the test compounds, the decrease in the ergosterol content varied. At half MIC values, mint EO showed the maximum decrease by 59% in sensitive and 42% in resistant strains, carvone was next with 46% in sensitive, and 32% in resistant strains. Menthol and menthone showed a decline in the ergosterol content by only 15–25%. At even lower concentration (MIC/4), mint EO showed a decline in ergosterol content by 46% in sensitive and 38% in resistant while carvone showed a decline by 30% in sensitive and 22% in resistant Candida strains. The decrease was not significant in case of menthol and menthone at MIC/4, the values being less than 17%.
Table 3. Reduction in ergosterol content by mint essential oil, carvone, menthol and menthone in Candida cells.
Combination studies of FLC with mint EO
Azoles have excellent efficacy–toxicity profiles and the most widely used azole in both the treatment and the prevention of candidiasis is FLC (Vanden Bossche, Citation1985). However, treatment failures are increasing, especially in AIDS patients, as prolonged use of this drug has led to resistance in Candida spp. probably due to its fungistatic rather than fungicidal nature of antifungal action (Sanglard et al., Citation2003; Uppuluri et al., Citation2008). New therapeutic strategies are required to reduce the toxicity of these drugs.
The perspective on antifungal drug resistance and other problems suggest several possible ways of overcoming, or preventing, drug resistance. The efficacy of FLC and other antifungal agents can be improved by using combination therapy (Ghannoum et al., Citation1995; Mukherjee et al., Citation2005; Pinto et al., Citation2009; Tariq et al., Citation1995). Combinational therapy can be used to attack more than one target simultaneously with a low probability so that resistance to both drugs will arise, or to attack a drug target and its resistance mechanism. Synergistic antifungal activity of carvone and essential oils rich in carvone, with azoles, is well described against Candida (Dudhatra et al., Citation2012).
To assess the interaction of drug combinations (mint EO plus FLC, carvone plus FLC, menthol plus FLC, and menthone plus FLC), the MIC data were further analyzed using the fractional inhibitory concentration index (FICI) (Berenbaum, Citation1989). The FICI was calculated by the formula,
where MICA and MICB are the MICs of drugs A and B, respectively. The interaction was defined as synergistic if the FICI was ≤0.5, indifferent if the FICI was >0.5 and ≤4, and antagostic if the FICI was >4.0 (Cantón et al., Citation2005).
MIC value of FLC against the standard susceptible and resistant strains ranged from 7.5 to 8.0 and 64 to 80 µg/ml, respectively. FICI of mint EO in combination with FLC against all FLC sensitive and FLC-resistant Candida strains studied, calculated from the checkerboard microtiter assay ranged from 0.28 to 0.41 and 0.26 to 0.35, respectively, indicating significant synergism (). Carvone in combination with FLC gave an FICI of 0.28–0.42 in susceptible and 0.38–0.48 in resistant Candida strains. FICI of menthol with FLC was 0.36–0.41 and 0.26–0.49, respectively, in susceptible and resistant cells. Menthone did not show significant synergism with FLC. Almost all FICI values were showing indifferent interaction.
Table 4. Synergistic antifungal activity (MIC and FICI) of mint EO and its major compounds against fluconazole-sensitive standard strains and fluconazole-resistant strains.
Scanning electron microscopy
Alterations in the morphology of Candida cells in the presence of test compounds were studied using scanning electron microscopy (SEM). shows SEM images of C. albicans ATCC 90028 cells when exposed to test compounds for 14 h at their respective MIC values. shows the SEM micrographs of untreated cells having well-defined shape and normal smooth surfaces. Cells treated with mint EO and its lead compound carvone at their respective MIC values for 14 h showed extensive cell damage and leakage of the intracellular content in large quantities (). Images show changes in the cell shape due to the formation of deep wrinkles in both the cases.
Hemolytic activity
The effects of mint EO and its lead molecules were studied on human red blood cells and are shown in . At 2000 μg/ml, mint EO, carvone, menthol, and menthone showed 6, 19, 27, and 42% hemolysis, respectively, while FLC, a conventional antifungal therapeutic agent, showed 85% hemolysis at the same concentration. Hence hemolytic activity was in the following order: FLC > menthone > menthol > carvone > mint EO. All the compounds tested here showed less hemolysis in comparison with a conventional drug even at very high concentrations.
Discussion
Although there has been a surge of improved antifungal drugs for the treatment of fungal infections, not many drugs are effective against invasive fungal infections, and hence remain a significant clinical problem. Infections caused by eukaryotic organisms like yeasts are generally more problematic than bacterial infections. There are only a few antifungal agents that can identify targets unique to the fungus and not shared with human host. The fact that most available antifungal drugs are fungistatic leads to the emergence of intrinsically resistant fungal species. Moreover, high treatment costs and associated host cytotoxicity justify the search for new strategies to combat fungal infections. Natural products provide an unparalleled source of chemical scaffolds with diverse biological activities. Plants and plant products significantly contribute to antimicrobial drug discovery (Khan et al., Citation2010a,Citationb). Our results suggest that plant active principles can be developed into potential antifungal agents.
As reported earlier (Burt, Citation2004; Palmeira-de-Oliveira et al., Citation2009), majority of the essential oils act by disrupting microbial cytoplasmic membranes. In the present study, we also show membrane damage as a mode of action of mint EO and its major constituents. The antimicrobial activity of essential oils and their components have been studied extensively (Cristani et al., Citation2007; Lucchini et al., Citation1990; Xu et al., Citation2008), but not much is known about their mechanism of action. This work is an attempt to assess the antifungal role of mint EO, carvone, menthol, and menthone by studying their effect on structural and functional aspects of membrane integrity in terms of ergosterol content and PM-H+ ATPase activity.
PM-H+ ATPase plays a crucial role in fungal cell physiology and maintains electrochemical proton gradient across the cell membrane necessary for nutrient uptake. It regulates pHi, changes which are widely regarded as of crucial importance to cell physiology and morphogenesis (Kaur et al., Citation1988; Manzoor et al., Citation2002). We explored the effect of mint EO and its major constituents on glucose-induced H+ efflux which is an estimate of PM-H+ ATPase activity. Inhibition of enzyme activity correlates well with the cessation of cell growth and this observation is in accordance with previous studies as well. Azoles did not affect medium acidification by yeast cells even at concentrations higher than its MIC value (Ben Josef et al., Citation2000; Manavathu et al., Citation1999) while mint EO, carvone, menthol, and menthone could do so at MIC values. It may be conjectured that the test compounds are possibly interactive with the enzyme directly, serving as the primary reason for their antifungal activity. Our studies also suggest the presence of cellular target that is accessible to the compounds externally. Thus, it would be useful to further investigate the interaction of the test compounds with purified PM-ATPase enzyme. Existing classes of antifungals have low efficacy, high toxicity, and frequently lead to drug resistance. There is thus a critical need to develop more effective therapies to deal with such infections and natural essential oils like mint EO and its active components offer a safer alternative. Our results suggest that PM H+-ATPase can be explored as a potential surface active antifungal target for these and other potential drugs.
Although natural antifungal compounds act by disrupting cytoplasmic membranes of fungi (Ahmad et al., Citation2011), specific mechanisms involved in their mode of action remain poorly characterized. It was interesting to observe that cell leakage induced by mint EO and its main constituents was far greater than that induced by FLC which is an established membrane-targeting antifungal drug. Ergosterol is a unique membrane sterol of fungi. The selective cytotoxic behavior and affinity of test compounds for this particular target hints towards their interference with ergosterol biosynthesis pathway. Analysis of ergosterols obtained from FLC-sensitive and resistant Candida strains showed no major difference in the sterol content or pattern in comparison with the untreated control. Growth of Candida in the presence of sub-inhibitory concentrations of the test compounds altered the sterol patterns of the FLC-sensitive and resistant strains in a similar manner. These plant active principles completely blocked ergosterol biosynthesis at MIC values. Studying the effect of these compounds at various sub-inhibitory concentrations on the sterols of the FLC-resistant Candida strains showed that they act in a dose-dependent fashion in decreasing the ergosterol content ( and ). In the present study, very significant inhibition of ergosterol biosynthesis was observed even at the sub-MIC concentration of mint EO and its lead constituents. The effect on membrane integrity, thus, appears to originate from ergosterol biosynthesis inhibition in a manner similar to that of FLC. The antifungal effects were attributed to their ability to inhibit ergosterol biosynthesis and to make the cytoplasmic membrane porous in both sensitive and resistant strains. SEM analysis performed in the present study on a representative Candida strain ATCC 90028 cells treated with compounds for 14 h clearly demonstrates damaging effect of mint EO and its active compound carvone on membrane and cell wall structure.
Conclusion
This work suggests that naturally occurring plant active principles – mint EO, carvone, menthol, and menthone, could be developed into promising antifungal drugs and also advocates the determination of their optimal concentrations for clinical application, as an alternative to FLC therapy for the treatment and prevention of candidiasis. Mint EO and its major constituents are fungicidal bioactive compounds that have a profound effect on PM-ATPase of Candida species making this membrane protein a very good potential antifungal target. These compounds penetrate the cell membrane and target ergosterol biosynthesis pathway, thus, impairing its biosynthesis. Simultaneously they react with the membrane itself with their reactive hydroxyl moiety, and the extensive lesion on membrane is a combined effect of the two events. Although the hypothesis requires identification/screening of the target enzyme(s) of the pathway, involvement of hydroxyl group in the formation of hydrogen bonds and the acidity of these phenolic compounds may provide possible explanations. The excellence of these compounds demand more insight studies into all possible mechanisms of these compounds.
Declaration of interest
The authors report that they have no conflicts of interest. This work was supported by Indian Council of Medical Research, India (59/24/2008/BMS/TRM [2008-04780]).
References
- Ahmad A, Khan A, Akhtar F, et al. (2011). Fungicidal activity of thymol and carvacrol by disrupting ergosterol biosynthesis and membrane integrity against Candida. Eur J Clin Microbiol Infect Dis 30:41–50
- Ahmad A, Khan A, Khan L, Manzoor N. (2010a). In vitro synergy of eugenol and methyleugenol with fluconazole against clinical Candida isolates. J Med Microbiol 59:1178–84
- Ahmad A, Khan A, Manzoor N, Khan LA. (2010b). Evolution of ergosterol biosynthesis inhibitors as fungicidal against Candida. Microb Pathog 48:35–41
- Ahmad A, Khan A, Yousuf S, et al. (2010c). Proton translocating ATPase mediated fungicidal activity of eugenol and thymol. Fitoterapia 81:1157–62
- Arthington-Skaggs BA, Jradi H, Desai T, Morrison CJ. (1999). Quantitation of ergosterol content: Novel method for determination of fluconazole susceptibly of Candida albicans. J Clin Microbiol 37:3332–7
- Ben-Josef AM, Manavathu EK, Platt D, Sobel JD. (2000). Proton translocating ATPase mediated fungicidal activity of a novel complex carbohydrate: CAN-296. Int J Antimicrob Agents 13:287–95
- Berenbaum MC. (1989). What is synergy? Pharmacol Rev 41:93–141.
- Burt S. (2004). Essential oils: Their antibacterial properties and potential applications in foods – A review. Int J Food Microbiol 94:223–53
- Cantón E, Pemán J, Gobernado M, et al. (2005). Synergistic activities of fluconazole and voriconazole with terbinafine against four Candida species determined by checkerboard, time-kill, and Etest methods. Antimicrob Agents Chemother 49:1593–6
- Carretto CFP, Almeida RBA, Furlan MR, et al. (2010). Antimicrobial activity of Mentha piperita L. against Candida spp. Braz Dent Sci 13:4–9
- CLSI. (2008). Reference Method for Broth Dilution Antifungal Susceptibility Testing of Yeasts. Approved Standard M27-A3, 3rd ed. Wayne, PA: Clinical and Laboratory Standards Institute
- Cohen ML. (1998). Resurgent and emergent disease in a changing world. Br Med Bull 54:523–32
- Cristani M, D'Arrigo M, Mandalari G, et al. (2007). Interaction of four monoterpenes contained in essential oils with model membranes: Implications for their antibacterial activity. J Agric Food Chem 55:6300–8
- Derwich E, Benziane Z, Chabir R. (2011). Aromatic and medicinal plants of Morocco: Chemical composition of essential oils of Rosmarinus officinalis and Juniperus phoenicea. IJABPT 2:145–53
- Dudhatra GB, Mody SK, Awale MM, et al. (2012). A comprehensive review on pharmacotherapeutics of herbal bioenhancers. Sci World J 2012:1–33
- Ghannoum MA, Fu Y, Ibrahim AS, et al. (1995). In vitro determination of optimal antifungal combinations against Cryptococcus neoformans and Candida albicans. Antimicrob Agents Chemother 39:2459–65
- Iwu MW, Dunean AR, Okunji CO. (1999). New antimicrobials of plant origin. In: Janick J, ed. Perspectives on New Crop and New Uses. Alexandria: ASHS Press, 457–62
- Kaneshima S, Koga S, Tanaka K. (1977). A simple method for cellular identification of free cell by light microscopy and scanning electron microscopy. J Electron Microsc 26:355–8
- Kaur S, Mishra P, Prasad R. (1988). Dimorphism associated changes in intracellular pH of Candida albicans. Biochim Biophys Acta 972:277–82
- Khan A, Ahmad A, Manzoor N, Khan LA. (2010a). Antifungal activities of Ocimum sanctum essential oil and its lead molecules. Nat Prod Commun 5:345–9
- Khan A, Ahmad A, Xess I, et al. (2010b). Anticandidal effect of Ocimum sanctum essential oil and its synergy with fluconazole and ketoconazole. Phytomedicine 17:921–5
- Li J, Dong J, Qiu JZ, et al. (2011). Peppermint oil decreases the production of virulence-associated exoproteins by Staphylococcus aureus. Molecules 16:1642–54
- Lucchini JJ, Corre J, Cremieux A. (1990). Antibacterial activity of phenolic compounds and aromatic alcohols. Res Microbiol 141:499–510
- Manavathu EK, Dimmock JR, Vashishtha SC, Chandrasekar PH. (1999). Proton-pumping-ATPase-targeted antifungal activity of a novel conjugated styryl ketone. Antimicrob Agents Chemother 43:2950–9
- Manzoor N, Amin M, Khan LA. (2002). Effect of phosphocreatine on H+ extrusion, pHi and dimorphism in Candida albicans. Indian J Exp Biol 40:785–90
- Mares D. (1989). Electron microscopy of Microsporum cookie after in vitro treatment with protoanemonin: A combined SEM and TEM study. Mycopatholgia 108:37–47
- Maschmeyer G. (2006). The changing epidemiology of invasive fungal infections: New threats. Int J Antimicrob Agents 27:3–6
- Matasyoh JC, Maiyo ZC, Ngure RM, Chepkorir R. (2009). Chemical composition and antimicrobial activity of essential oil of Coriandrum sativum. Food Chem 113:526–9
- Mukherjee PK, Sheehan DJ, Hitchcock CA, Ghannoum MA. (2005). Combination treatment of invasive fungal infections. Clin Microbiol Rev 18:163–94
- Palmeira-de-Oliveira A, Salgueiro L, Palmeira-de-Oliveira R, et al. (2009). Anti-Candida activity of essential oils. Mini Rev Med Chem 9:1292–305
- Perlin DS, Seto-Young D, Monk BC. (2006). The plasma membrane H+ ATPase of fungi. A candidate drug target? Ann NY Acad Sci 834:609–17
- Pfaller MA, Diekema DJ. (2004). Rare and emerging opportunistic fungal pathogens: Concern for resistance beyond Candida albicans and Aspergillus fumigates. J Clin Microbiol 42:4419–31
- Pinto E, Vale-Silva L, Cavaleiro C, Salgueiro L. (2009). Antifungal activity of the clove essential oil from Syzygium aromaticum on Candida, Aspergillus and Dermatophyte species. J Med Microbiol 58:1454–62
- Saharkhiz MJ, Motamedi M, Zomorodian K, et al. (2012). Chemical composition, antifungal and antibiofilm activities of the essential oil of Mentha piperita L. ISRN Pharm 2012:1–6
- Sandasi M, Leonard CM, Van-Vuuren SF, Viljoen AM. (2011). Peppermint (Mentha piperita) inhibits microbial biofilms in vitro. South Afr J Bot 77:80–5
- Sanglard D, Ischer F, Parkinson T, et al. (2003). Candida albicans mutations in the ergosterol biosynthetic pathway and resistance to several antifungal agents. Antimicrob Agents Chemother 47:2404–12
- Serrano R. (1983). In vivo glucose activation of the yeast plasma membrane ATPase. FEBS Lett 156:11–14
- Set-Young D, Monk BC, Mason AB, Perlin DS. (1997). Exploring an antifungal target in the plasma membrane H+ ATPase of fungi. Biochim Biophys Acta 1326:249–56
- Stewart E, Gow NA, Bowen DV. (1988). Cytoplasmic alkalinization during germ tube formation in Candida albicans. J Gen Microbiol 134:1079–87
- Tariq VN, Scott EM, McCain NE. (1995). Use of decimal assay for additivity to demonstrate synergy in pair combinations of econazole, nikkomycin Z, and ibuprofen against C. albicans in vitro. Antimicrob Agents Chemother 39:2615–19
- Uppuluri P, Nett J, Heitman J, Andes D. (2008). Synergistic effect of calcineurin inhibitors and fluconazole against C. albicans biofilms. Antimicrob Agents Chemother 52:1127–32
- Vanden Bossche H. (1985). Biochemical targets for antifungal azole derivatives: Hypothesis on the mode of action. Curr Top Med Mycol 1:313–51
- Xu J, Zhou F, Ji BP, et al. (2008). The antibacterial mechanism of carvacrol and thymol against Escherichia coli. Lett Appl Microbiol 47:174–9
- Yatime L, Pedersen MJ, Musgaard M, et al. (2009). P-type ATPase as drug targets: Tools for medicine and science. Biochim Biophys Acta 1787:207–20