Abstract
Context: Macrophage, involved at all stages of immune response, is an important component of the host defense system. Polysaccharides exist almost ubiquitously in medical plants and most of them possess immunomodulation and macrophage activation properties.
Objective: This study elucidates the effects on macrophage activation and molecular mechanism induced by the polysaccharides (SOPs) from the roots of Sanguisorba officinalis Linne (Rosaceae).
Materials and methods: Polysaccharides (SOPs) from the roots of S. officinalis were obtained by water extraction and ethanol precipitation. Physicochemical characterization of SOPs was analyzed by phenol–sulfuric acid, m-hydroxydiphenyl, Bradford method, and gas chromatography. Phagocytic capacity of RAW 264.7 macrophages incubated with SOPs (25 and 100 μg/ml) was determined by the aseptic neutral red method. Macrophages were incubated with SOPs (25 and 100 μg/ml), and the TNF-α and NO the secretion were measured using ELISA kit and Griess reagent, respectively. In addition, TNF-α and iNOS transcripts were evaluated by semi-quantitative RT-PCR, and NF-κB signaling activation was detected by Western blot assay.
Results: SOPs enhanced the phagocytosis capacity of macrophages to aseptic neutral red solution and increased TNF-α and NO secretion. The amounts of TNF-α and iNOS transcript were increased significantly at the mRNA level when macrophages were exposed to SOPs. Meanwhile, the stimulation of macrophages by SOPs induced phosphorylation of p65 at serine 536 and a marked decrease of IκB expression.
Discussion and conclusion: These results suggested that SOPs exhibited significant macrophage activation properties through NF-κB signaling pathway and could be considered as a new immunopotentiator.
Introduction
The enhancement or potentiation of host defense mechanisms has been recognized as a possible means of inhibiting tumor cell without any harm to the host (Schepetkin & Quinn, Citation2006). Macrophages are known to be the pivotal immunocytes of host defense against invading pathogen or tumor growth (Varin & Gordon, Citation2009). Activated macrophages release a wide range of mediators including reactive oxygen species (ROS), nitric oxide (NO), hydrolytic enzymes, bioactive lipids, and proinflammatory cytokines such as tumor necrosis factor (TNF)-α and interleukin (IL)-1, which have been identified as the major effector molecules involved in the destruction of tumor cells by macrophages (Gordon & Martinez, Citation2010; Pan, Citation2012).
Polysaccharides, especially β-d-glucan derivates, glycopeptide/protein complexes, and proteoglycans, have been considered to posses the immunomodulating and antitumor activities, which are related to their effects to act on immune effector cells such as lymphocytes, macrophages, dendritic cells (DCs), and natural killer (NK) cells, involved in the innate and adaptive immunities.
Sanguisorba officinalis Linne (Rosaceae) is a traditional Chinese herbal plant, officially listed in the Chinese Pharmacopoeia, with multiple pharmacological functions, such as anti-infection, anti-inflammatory, antitumor, and neuroprotective effect (Cai et al., Citation2012; Lee et al., Citation2010; Nguyen et al., Citation2008; Yu et al., Citation2011). Although numerous activities of this plant have been reported and published so far, not many reports explicitly explain the mechanisms that underlie the immunomodulation and macrophage activation properties of S. officinalis. Therefore, the purpose of present investigation was to elucidate the effects and possible mechanism of macrophage activation induced by the water-soluble polysaccharide from S. officinalis.
Materials and methods
Plant material
The plant material of S. officinalis was purchased from local pharmaceutical market in September 2011, and identified by Dr. Yu Hou, School of Life Science, Beihua University. The specimen was deposited in School of Life Science, Beihua University, with the Voucher Specimen Number of P-2013-00784.
Chemicals and reagents
Chemiluminescent detection kit of ECL plus western blotting reagents was purchased from Amersham Pharmacia Biotech (Buckinghamshire, UK). Lipopolysaccharides (LPS) and Griess reagent were obtained from Sigma (St. Louis, MO). Affi-Prep Polymyxin Matrix was purchased from BIO-RAD (Hercules, CA). RPMI 1640 medium and fetal bovine serum (FBS) were the products of Gibco (Grand Island, NY). Anti-NF-κB p65 antibody was obtained from Abcam (Cambridge, UK). Phospho-NF-κB p65 (Ser536) and IκB-α antibodies were purchased from Cell Signaling Technology (Danvers, MA). Antibody against β-actin (AC-40) was obtained from Sigma-Aldrich (St. Louis, MO). All other chemical reagents were of analytical grade.
Cell culture
RAW264.7 murine macrophages were cultured in Dulbecco’s modified Eagle’s medium (DMEM) supplemented with 10% fetal bovine serum, 2 mM l-glutamine, 100 U/ml penicillin, and 100 μg/ml streptomycin. Cultures were maintained at 37 °C in a 5% CO2 humidified atmosphere.
Isolation and purification of polysaccharide
After cleaned and dried, the roots of S. officinalis were ground, and the powders were extracted with distilled water at 80 °C. The whole extract was filtered, concentrated, and centrifuged, and then the supernatant was treated with three volumes of ethanol at 4 °C overnight. The crude polysaccharide precipitate was collected by centrifugation, and dried under reduced pressure after washed with dehydrated alcohol and diethyl ether. The sample was dissolved in distilled water, then frozen at −20 °C, thawed at room temperature and centrifuged to remove insoluble materials. The supernatant was deproteinated by a combination of proteinase and Sevag method (Staub, Citation1965). And then, the supernatant was collected, dialyzed, and lyophilized to obtain water-soluble S. officinalis polysaccharides (SOPs). Possible contaminants of endotoxin contained in SOPs were removed using Affi-Prep Polymyxin Matrix (BIO-RAD, Hercules, CA).
Analytical methods for physicochemical characterization
Total carbohydrate content of SOPs was determined by the phenol–sulfuric acid colorimetric method using glucose as the standard (Dubois et al., Citation1956). Total uronic acid content was measured by m-hydroxydiphenyl method using glucuronic acid as the standard (Filisetti-Cozzi & Carpita, Citation1991). Protein content was quantified according to Bradford’s method with bovine serum albumin as the standard (Bradford, Citation1976). The molecular weight range of SOPs was evaluated by HPLC (Shimadzu, Kyoto, Japan) equipped with a TSKGEL G3000 PWXL column and RID-10A Refractive Index Detector. Gas chromatography (GC) was used for identification and quantification of monosaccharide compositions of polysaccharides. Polysaccharides were hydrolyzed and acetylated according to Lehrfeld (1985). The acetylated products were analyzed by GC, which was performed on an Agilent 6890 instrument (Agilent Technologies, Santa Clara, CA) equipped with the DM-2330 capillary column (30 m × 0.32 mm × 0.2 μm) and flame-ionization detector (FID). Ultraviolet-visible spectra were recorded with Varian Cary-100 spectrophotometer (Varian Inc., Victoria, Australia). Absorbance readings were performed at 260 or 280 nm.
Measurement of phagocytic capacity of macrophages
RAW264.7 macrophages were incubated with SOPs (25 and 100 μg/ml) for 24 h, and then 100 μl of aseptic neutral red solution (0.075%) was added into each well and incubated for another 1 h. After washed with PBS, the cells were lysed by adding 150 μl cell lysis buffer (anhydrous ethanol:acetic acid = 1:1, v/v). The absorbance was measured at 550 nm using Bio-Tek EXL800 microplate reader (Bio-Tek, Winooski, VT). The absorbance represented the phagocytic ability of the macrophages.
Assay of TNF-α and NO secretion of RAW 264.7 macrophages
RAW 264.7 macrophages were cultured in 96-well plates. After incubation with SOPs in different concentrations (25 and 100 μg/ml) for 24 h, TNF-α secretion was measured using enzyme-linked immunosorbent assay (ELISA) kit. LPS (1 μg/ml) was used as the positive control. To measure nitrite, 100 μl of the cell-free culture medium was removed and placed into new 96-well plates. Griess reagent (100 μl) was added into each well and incubated for 10 min at room temperature. The absorbance was measured at 540 nm using Bio-Tek EXL800 microplate reader (Bio-Tek, Winooski, VT). The nitrite amount in each sample was calculated using a standard curve generated with sodium nitrite (from 0 to 100 μM in the cell culture medium).
TNF-α and iNOS transcript of RAW 264.7 macrophages
RAW 264.7 macrophages were cultured in the presence of 25 and 100 μg/ml SOPs in 6-well tissue culture plate (1 × 106 cells/well) for 24 h. Total RNA (1 μg) isolated from the stimulated cells were reverse transcribed into cDNA using Promega RT-PCR kit (Promega, Madison, WI). Primers used in the present study were as follows: TNF-α (forward 5′-GAGAAGTTCCCAAATGGC-3′ and reverse 5′-CACTTGGTGGTTTGCTACG-3′), and iNOS (forward 5′-ATGGACCAGTATAAGGCAAGC-3′ and reverse 5′-GCTCTGGATGAGCCTATATTG-3′). The cDNAs from the reverse transcription reactions were amplified under the following conditions: denaturation at 94 °C for 30 s, annealing at 55 °C for 30 s, and extension at 72 °C for 60 s with a final extension at 72 °C for 5 min. Amplified cDNA products were resolved on 1.5% agarose gel by electrophoresis and then stained with ethidium bromide.
NF-κB signaling activation
NF-κB signaling activation was detected by Western blot assay. RAW 264.7 macrophages were cultured in the presence of SOPs (25 and 100 μg/ml) in 6-well tissue culture plate (1 × 106 cells/well) for 24 h. Cell pellets were lysed with RIPA lysis buffer and then centrifuged at 12 500 g for 20 min at 4 °C to remove cell debris. The supernatants were subjected to SDS-PAGE, and then proteins were transferred to NC membranes. The membranes were incubated with 5% nonfat milk in TBST, and then with the indicated primary antibodies (IκB, p65, and phosphorylation of p65) and the HRP-conjugated secondary antibodies. Chemiluminescent detection was performed by using ECL Plus Western blotting reagents. Quantification was performed using ImageJ software (National Institutes of Health, Bethesda, MD).
Statistical analysis
All statistical analyses were performed with SPSS version 13.0 (SPSS Inc., Chicago, IL) for Windows. Data were expressed as mean ± SEM and examined for their statistical significance of difference with one-way ANOVA. p-Values of less than 0.05 were considered to be statistically significant.
Results
Physicochemical characterization of SOPs
The total content of carbohydrate, protein, and uronic acid, and the monosaccharide composition of SOPs are summarized in . The yield of SOPs was 3.7% of dried material. The carbohydrate content of SOPs was 97.7%, and uronic acid was not detected in SOPs. In addition, SOPs had a negative response to the Bradford assay and no absorption at 260 or 280 nm in the UV spectrum was detected indicating the absence of protein and nucleic acid. The molecular weight range of SOPs was from 470 to 7.2 kDa, and GC analysis showed that SOPs were composed of four kinds of monosaccharide, namely arabinose, mannose, galactose, and glucose, with a molar ratio of 1:3.3:4.1:7.6.
Table 1. Physicochemical properties of SOPs.
SOPs enhance phagocytosis capacity of RAW 264.7 macrophage
Phagocytic activity of macrophages to aseptic neutral red solution was examined in vitro. As shown in , the absorbance at 550 nm was markedly increased when the cells were treated with SOPs, which indicated that SOPs significantly promoted the phagocytic capacity of RAW 264.7 macrophage. Compared with the negative control group, phagocytosis capacity was significantly increased by SOPs treatment at the doses of 100 μg/ml (p < 0.001). Moreover, we also found that SOPs-stimulated macrophages had obviously morphological changes from round cells to irregular cells (data not shown), which showed a typical morphology of macrophage activation.
SOPs promote TNF-α and NO secretion of RAW 264.7 macrophages
RAW 264.7 macrophages were stimulated by SOPs, the culture supernatants were collected at 24 h, and the amounts of TNF-α and NO were measured. As shown in , when the macrophages were treated with SOPs at 100 μg/ml, the level of TNF-α was significantly increased compared with negative control (p < 0.01). SOPs-activated macrophages produced especially large amounts of TNF-α (454.1 pg/ml) at the concentration of 100 μg/ml, which was over 5.7-fold compared with the negative control (medium alone), and that approximated the effects of LPS (p > 0.05). As shown in , a minimum amount of NO was produced when macrophages were incubated with medium alone, whereas the treatment with SOPs at the concentration of 100 μg/ml led to a significant increase in NO production, enhancing the response >10-fold compared with the negative control. Further, the gene expression of TNF-α and iNOS was detected at mRNA level by semi-quantitative RT-PCR. As shown in , the transcripts of TNF-α and iNOS were hardly detectable in unstimulated macrophages. When the macrophages were exposed to SOPs for 24 h, the amounts of TNF-α and iNOS transcript were increased significantly.
Figure 2. Effect of SOPs on TNF-α secretion by RAW 264.7 macrophages. Values are means ± SEM; **p < 0.01 versus the control group; #p > 0.05 versus the LPS group.
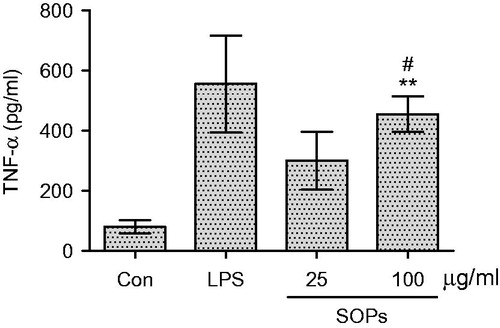
Figure 3. Effect of SOPs on NO secretion by RAW 264.7 macrophages. Values are means ± SEM; **p < 0.01 versus the control group; #p > 0.05 versus the LPS group.
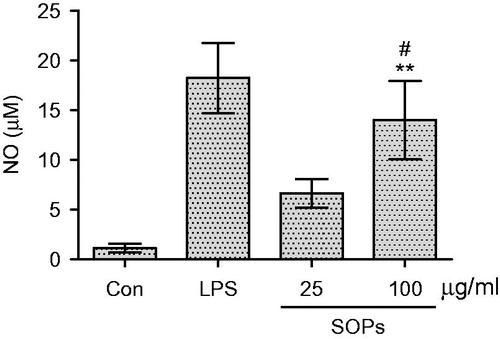
Figure 4. Effect of SOPs on the mRNA transcript level of TNF-α and iNOS in RAW 264.7 macrophages by RT-PCR. Transcripts of GAPHD were served as internal controls. A representative gel graph from three experiments was shown. The semi-quantitative analysis of mRNAs level of TNF-α (B) and iNOS (C) was performed using ImageJ software (National Institutes of Health, Bethesda, MD). Values are means ± SEM; *p < 0.05 and **p < 0.01 versus the control group; #p > 0.05 versus the LPS group.
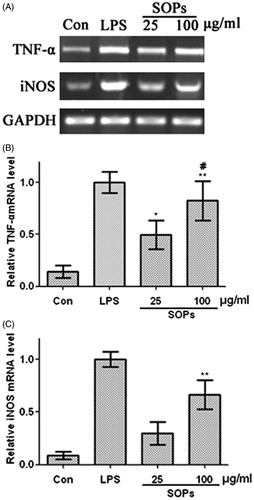
SOPs activate NF-κB in stimulated macrophage
The phosphorylation of p65 protein (S536) and IκB degradation of macrophages stimulated by SOPs were investigated using the Western blot analysis. As shown in , a small amount of p65 protein was phosphorylated in macrophages incubated with medium alone, whereas the p65 phosphorylation occurred after SOPs treatment, and the p65 phosphorylation level was markedly promoted for about 3.5-fold at the concentration of 100 μg/ml, quantitatively analyzed by NIH ImageJ software (National Institutes of Health, Bethesda, MD), compared with the negative control (medium alone). Besides, there was a significant decrease of IκB expression by SOPs-treatment compared with the negative control. Taken together, those results indicated that SOPs could activate NF-κB signaling, which may be the molecular mechanism of macrophage activation by the polysaccharides from S. officinalis.
Figure 5. SOPs induced NF-κB activation in RAW 264.7 macrophages. RAW 264.7 macrophages were exposed to SOPs for 24 h. The whole cell lysates were probed with indicated antibodies. The quantitative analysis was performed using ImageJ software. The images are a representative of three independent experiments.
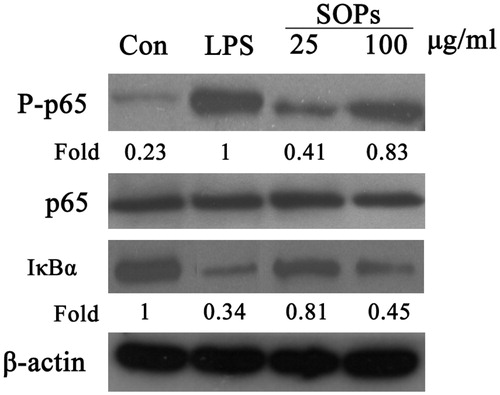
Discussion
Polysaccharide or polysaccharide-peptide/protein complexes are carbohydrate polymers that can be found abundantly in the cell wall of medical plant, and most of them have shown immunomodulating properties, which are effective in treating and preventing immune dysfunction or tumor without low side effects (Schepetkin & Quinn, Citation2006; Zong et al., Citation2012). Although it has been reported that the polysaccharides from S. officinalis possess numerous activities, none report explicitly explain the mechanism of SOPs involved in the immunomodulation and macrophage activation.
Macrophages are involved at all stages of the immune response, which have been considered as an important component of the host defense system (Locati et al., Citation2013). Activation of macrophages is a key event for initiating and propagating defensive reactions against pathogens. Phagocytosis is the first step in the macrophage response to invading microorganisms, and activated macrophages further function in antigen processing and presentation, and antibody-dependent cell-mediated cytotoxicity (Liu & Yang, Citation2013; Mantovani et al., Citation2013). In addition, they also induce the production of numerous cytokine or mediators, such as TNF-α, interferons, interleukins, NO, and other reactive oxygen species. The roles of TNF-α include activation and chemotaxis of leukocytes and induction of the expression of adhesion molecules. NO can diffuse freely through cells and is synthesized from l-arginine by NOS, which contributes to killing microorganisms and tumor cells through activated macrophages and mediates a variety of biological functions as an intracellular messenger molecule. In addition, TNF-α and NO can destroy and inhibit the growth of malignant cells or activate other immunocyte, which are indispensable for keeping homeostasis (Siveen & Kuttan, Citation2009; Wynn et al., Citation2013). Here, we observed that SOPs could significantly enhance the macrophage phagocytosis and increase the release of TNF-α and NO produced by macrophages. Consistent with those results, the genes expression of TNF-α and iNOS was also significantly increased at mRNA level after treating with SOPs, which may explain the immunostimulatory properties of SOPs.
The recognition between polysaccharide ligand and receptors including TLR4 and Dectin-1 on the surface of macrophage induces the activation of various transcription factors such as NF-κB family. NF-κB family members are served as inducible transcription factors and play essential roles in host immune and inflammatory responses, which are required for the cytokine gene expression, such as TNF-α and iNOS. Once activated, the transcription factors of NF-κB family translocate into nucleus, where they bind to specific sequences in the promoter region of immune response gene, then up-regulate gene expression (Hayden & Ghosh, Citation2004; Vallabhapurapu & Karin, Citation2009). Our results showed SOPs induced an increase in TNF-α and NO production and their gene expression, thus, we wanted to confirm whether SOPs can activate NF-κB signaling pathway inducing the expression of TNF-α and iNOS. NF-κB consists of heterodimeric protein complex, such as p65 and p50 subunits, and the inactive p65 is deposited in the cytoplasm with the inhibitory IκB proteins. When the macrophages were activated, the upstream IKK kinases phosphorylate IκB, leading to its ubiquitination and degradation, thereby allowing p65 to translocate to the nucleus. IKKs also phosphorylate p65 on a critical serine (S536) that modulates transcriptional activity (Hoesel & Schmid, Citation2013). We found that SOPs treatment induced the marked up-regulation of p65 phosphorylation at serine 536 site. Moreover, the IκB expression was significantly decreased with SOPs treatment. Therefore, we speculated that SOPs induced macrophage activation and increased TNF-α and NO production might be related to the activation of NF-κB signaling pathway, and further evidence is required to confirm.
In summary, we isolated the polysaccharides SOPs from S. officinalis, and our present study demonstrated that SOPs exhibited significant macrophage activation properties through NF-κB signaling pathway. These results suggest that SOPs can be considered as a new immunopotentiator to activate the immune system.
Declaration of interest
This work was financially supported by the Innovative Team of Plant Chemical Engineering of Jilin Province (No. 20130521022JH), National Natural Science Foundation of China (31401203), the Key Projects (Nos. 20140311056YY and 20140204039YY) and the Youth Foundation (No. 201201143) of Science and Technology Department of Jilin Province, the Scientific Foundation of Education Department of Jilin Province (2013-169).
References
- Bradford MM. (1976). A rapid and sensitive method for the quantitation of microgram quantities of protein utilizing the principle of protein binding. Anal Biochem 72:248–54
- Cai ZB, Li W, Wang HT, et al. (2012). Anti-tumor and immunomodulating activities of a polysaccharide from the root of Sanguisorba officinalis L. Int J Biol Macromol 51:484–8
- Dubois M, Gilles KA, Hamilton JK, et al. (1956). Colorimetric method for determination of sugars and related substances. Anal Chem 28:350–6
- Filisetti-Cozzi TM, Carpita NC. (1991). Measurement of uronic acids without interference from neutral sugars. Anal Biochem 197:157–62
- Gordon S, Martinez FO. (2010). Alternative activation of macrophages: Mechanism and functions. Immunity 32:593–604
- Hayden MS, Ghosh S. (2004). Signaling to NF-κB. Genes Dev 18:2195–224
- Hoesel B, Schmid JA. (2013). The complexity of NF-κB signaling in inflammation and cancer. Mol Cancer 12:86–101
- Lee NH, Lee MY, Lee JA, et al. (2010). Anti-asthmatic effect of Sanguisorba officinalis L. and potential role of heme oxygenase-1 in an ovalbumin-induced murine asthma model. Int J Mol Med 26:201–8
- Lehrfeld J. (1985). Simultaneous gas-Liquid chromatographic determination of aldonic acids and aldoses. Anal Chem 57:346–8
- Liu G, Yang H. (2013). Modulation of macrophage activation and programming in immunity. J Cell Physiol 228:502–12
- Locati M, Mantovani A, Sica A. (2013). Macrophage activation and polarization as an adaptive component of innate immunity. Adv Immunol 120:163–84
- Mantovani A, Biswas SK, Galdiero MR, et al. (2013). Macrophage plasticity and polarization in tissue repair and remodelling. J Pathol 229:176–85
- Nguyen TT, Cho SO, Ban JY, et al. (2008). Neuroprotective effect of Sanguisorbae radix against oxidative stress-induced brain damage: In vitro and in vivo. Biol Pharm Bull 31:2028–35
- Pan XQ. (2012). The mechanism of the anticancer function of M1 macrophages and their use in the clinic. Chin J Cancer 31:557–63
- Schepetkin IA, Quinn MT. (2006). Botanical polysaccharides: Macrophage immunomodulation and therapeutic potential. Int Immunopharmacol 6:317–33
- Siveen KS, Kuttan G. (2009). Role of macrophages in tumour progression. Immunol Lett 123:97–102
- Staub AM. (1965). Removal of protein-Sevag method. Methods Carbohydr Chem 5:5–6
- Vallabhapurapu S, Karin M. (2009). Regulation and function of NF-κB transcription factors in the immune system. Annu Rev Immunol 27:693–733
- Varin A, Gordon S. (2009). Alternative activation of macrophages: Immune function and cellular biology. Immunobiology 214:630–41
- Wynn TA, Chawla A, Pollard JW. (2013). Macrophage biology in development, homeostasis and disease. Nature 496:445–55
- Yu T, Lee YJ, Yang HM, et al. (2001). Inhibitory effect of Sanguisorba officinalis ethanol extract on NO and PGE2 production is mediated by suppression of NF-κB and AP-1 activation signaling cascade. J Ethnopharmacol 134:11–17
- Zong AZ, Cao HZ, Wang FS. (2012). Anticancer polysaccharides from natural resources: A review of recent research. Carbohydr Polym 90:1395–410