Abstract
Context: Alkaloids of Piper longum L. (Piperaceae) (PLA) include piperine and piperlonguminine. Piper longum and piperine have multiple biological properties including antioxidant activity.
Objective: The present study investigated the neuroprotective effects of PLA in a MPTP-induced mouse model of Parkinson’s disease.
Materials and methods: PLA was prepared by extracting the dry seed of P. longum using 85% ethanol. Adult male C57BL/6 mice were divided into eight groups of 12 rats each. Experimental and control groups received an equivalent volume of saline, 0.5% CMC-Na, and 0.1% Tween 80, treated groups received oral PLA (30, 60, and 120 mg/kg), other groups treated with piperine (60 mg/kg) or Madopar (50 mg/kg). The PLA prevention group (PLA-Pr) administrated PLA (120 mg/kg) for 1 week before MPTP challenged. Except for the PLA-Pr group, others were treated for seven consecutive weeks. Parkinson’s disease was induced by injecting MPTP intraperitoneally (25 mg/kg) twice weekly for five consecutive weeks. Dopaminerigic (DA) neurons and their metabolism were detected by UFLC-MS/MS. Tyrosine hydroxylase (TH)-immunohistochemistry assay and Western blotting were performed. The antioxidant enzymatic levels were determined by kit-based assays.
Results: The LD50 value of PLA was determined at 1509 mg/kg of body weight. PLA (60 mg/kg) can significantly increase total movement time and distance (p < 0.05), increase levels of DA (p < 0.05) and DOPAC (p < 0.05), increase glutathione (GSH) level and superoxide dismutase (SOD) activity (p < 0.05), and decrease the lipid peroxidation of malondiadehycle (MDA) (p < 0.05) in PLA-treated groups as compared with the control group.
Discussion and conclusion: Our results indicate that PLA possesses neuroprotective effects and has ameliorative properties in dopaminergic neurons.
Introduction
Parkinson’s disease (PD) is a chronically progressive neurodegenerative disease. It is characterized by the loss of pigmented dopaminergic neurons in the substantia nigral pars compacta (SNpc) of the midbrain and the presence of Lewy bodies (Dickson et al., Citation2009; Ma et al., Citation2008). A variety of hallmark motor symptoms linked to impaired cognitive functions in patients with PD include tremor, postural instability, and Parkinsonian gait and rigidity. These phenotypes can be observed both in the PD and in the animal models of PD. Oxidative stress has been implicated in the etiology of PD, although the cause of PD is still unknown (Wang et al., Citation2014). Neurons in the substantia nigra, as well as other pigmented and non-pigmented nuclei of the brain that degenerate in Parkinson's disease, may be sensitive to oxidative stress (Jenner, Citation2007). Additionally, it has been reported that the neuroprotective effects of dopaminergic drugs is associated with their antioxidant activity (Ciobica et al., Citation2012).
The toxicity of 1-methyl-4-phenyl-1,2,3,6-tetrahydropyridine (MPTP) is associated with oxidative stress such as nitric oxide. MPTP has been shown to cause PD in animals, similar to those observed in humans. MPTP injection in monkeys, cats, and mice can lead to loss of dopaminergic neurons in the SNpc and decreases DA levels in the striatum. Therefore, the MPTP mouse model is the most widely used to study PD (Kim et al., Citation2010; Pattarini, Citation2008). Neuropathological studies of PD indicate that the loss of nerve terminals in the striatum precedes the loss of DAergic neurons in the SNpc.
Despite years of using l-dopa and Madopar, current treatment options for PD are largely ineffective to reverse or prevent pathological outcomes. Because long-term levo-dopa treatment often causes dyskinesias, motor fluctuations, and psychiatric disturbances (Garrett et al., Citation1998; Schneider et al., Citation2003), traditional Chinese Medicine has been used to determine whether its use is more effective and less toxic. Several reports have shown that a number of herbal remedies possess neurotrophic and neuroprotective properties in PD-related models (Gaur et al., Citation2013; Hu et al., Citation2011; Kim et al., Citation2009; Mu et al., Citation2011; Xu et al., Citation2013).
Piper longum L. (Piperaceae) has been used as traditional medicine in Asia and the Pacific Islands. It is widely used as an analgesic and a treatment for stomach disease in China. Recently, multiple biological properties have been reported in preclinical studies, including anti-inflammatory, antisepsis, antioxidant activity, and free radical scavenger. Piperine is the major effective constituent in P. longum. It has also been suggested to have anti-anxiety effects, anti-inflammatory activity, and anti-HBV activity (Bi et al., Citation2011; Jiang et al., Citation2013). Previous studies have indicated that piperine may attenuate viability loss of MPTP-induced PC12 cells by suppressing the mitochondrial membrane permeability transition, leading to the activation of caspase-3 (Lee et al., Citation2006).
PLA is extracted from P. longum seed. It mainly contains 54.10% piperine, 3.74% piperlonguminine, and 0.62% Δα, β-dihydropiperlonguminine (Liu et al., Citation2013). At present, few studies have examined the neuroprotective functions of PLA in PD models. In our study, we experimentally demonstrate the neuroprotective effects of PLA and investigate its possible mechanisms against MPTP-induced neurotoxicity using open-field test, a tyrosine hydroxylase (TH)-immunohistochemistry and antioxidant assay. In addition, the highly sensitive UPLC-ESI-MS/MS method was applied to simultaneously measure seven kinds of dopamine and its metabolites in a sample.
Materials and methods
Chemicals and reagents
3,4-Dihydroxyphenylacetic acid (DOPAC), homovanillic acid (HVA), γ-aminobutyric acid (GABA), 5-hydroxytryptamine (5-HT), 5-hydroxyindoleacetic acid (5-HIAA), acetylcholine (Ach), MPTP-HCl, rabbit anti-tyrosine hydroxylase (TH) antibody, TritonX-100, Tris, glycine, SDS, DTT, APS, TEMED, Tween 20, Acr, Bis, Benzamidine, and Thimerosal were purchased from Sigma-Aldrich (St. Louis, MO). Madopar (Shanghai Roche Led., Shanghai, China) was obtained at the institutional pharmacy. BCA kit was purchased from Kodak (Rochester, NY). Piperine and caffeic acid reference (>99% purity lot numbers 0775-200203 and 110885-200102) were purchased from the National Institutes for Food and Drug Control (Beijing, China). Methanol and acetonitrile were purchased from Fisher (Waltham, MA). Superoxide dismutase (SOD), glutathione (GSH), and malondiadehycle (MDA) kits were purchased from Nanjing Jiancheng Bioengineering Institute (Jiangsu, China).
Plant material and preparation of extracts
Piper longum was purchased from Xinjiang, China, in 2010 and identified by Prof. Qing-Hua Liu. Voucher specimens (PLL-10-0518) have been deposited in School of Traditional Chinese Medicine, Capital Medical University.
The dry seed powder of P. longum (3 kg) was first extracted with 85% ethanol (8 L × 3) using heat reflux extraction (15 L, 120 °C). After filtration, the filtrate was concentrated. The concentrated solution was then mixed with clean D101 macroporous resin (3 kg) and dried using a rotary evaporator (EYELA, Tokyo, Japan). The dried mixture was subjected to the D101 macroporous resin (9 cm × 130 cm, 0.3–1.25 mm) and eluted with an ethanol–water gradient (0, 20, 40, 60, and 85%). The 60% fraction was collected and evaporated to dryness, which contains PLA. It contains 54.10% piperine, 3.74% piperlonguminine, and 0.62% Δα, β-dihydropiperlonguminine determined by HPLC (). The content of total alkaloids was 70.08% determined by UV.
Animals and drug treatments
Adult male C57BL/6 mice (n = 96, 22–25 g, 6–8 weeks old, WeiTongLihua, Beijing, China) were used in this study. The mice were housed five per cage at 23 ± 1 °C and 50–60% humidity, under 12 h light-dark cycle with artificial light conditions and freedom to access food and water. All experimental procedures were performed in accordance with the National Institutes of Health Guide for Care and Use of Laboratory Animals, and the experiments were also approved by Animal Ethics Committee of Capital medical university.
After adaptation for 10 d, 96 mice were randomly divided into eight groups (n = 12 animals per group). Mice of the control group and the experimental group received an equivalent volume of saline, 0.5% CMC-Na, and 0.1% Tween 80. All other groups received MPTP intraperitoneally (25 mg/kg, i.p.) twice weekly for five consecutive weeks. Mice of the PLA prevention group (PLA-Pr) were administrated PLA 120 mg/kg for 1 week before MPTP challenged, and the treatment lasted for 9 weeks with no interruption. After the second week of MPTP injection, mice of the other groups were intragastricly administered PLA, piperine, and Madopar as indicated in the following: the high dosage group were treated with PLA (120 mg/kg, once daily), the middle dosage group were treated with PLA (60 mg/kg, once daily), low dosage group were treated with PLA (30 mg/kg, once daily), the piperine group was treated with piperine (60 mg/kg, once daily), and the Madopar group was treated with Madopar (50 mg/kg, once daily). Except for the PLA-Pr group, all the groups were treated for seven consecutive weeks.
Open-field test
Mice in each group were tested by the Tru Scan behavioral surveillance system (by the U.S. Coulbourn Instrument Company, Whitehall, PA), which contains acoustic insulation and lucifugous field (60 cm × 60 cm × 65 cm). Infrared device was installed at the bottom of the box, which was used to accurately track the movement and the behavior. After the end of each test, 70% ethanol was used to thoroughly clean the open-field apparatus. The mice movement was recorded for the following parameters: total movement distance (cm) and total movement time (s). Each test time was 40 min.
UPLC-ESI-MS/MS analysis
Preparation of samples
The left striatal was weighed and homogenized in a 15-fold volume of formic acid (0.1 M), and the homogenates were centrifuged at 12 000 × g for 30 min at 4 °C. The supernatants were collected and 20 μL of the supernatant was diluted with 80 μL of water. Caffeic acid (250 ng/mL) was then added and used as an internal standard. Stock solutions of standard mixtures of GABA, Ach, DA, 5-HT, DOPAC, 5-HIAA, and HVA were prepared by dissolving a 1.00 mg/mL solution of each compound with DMSO.
Apparatuses
Chromatographic analyses were performed in an Acquity UPLC™ system (Waters, Milford, MA) using a Waters Acquity UPLC® BEH C18 column (2.1 mm × 100 mm), with 1.7 μm particle size. Mass spectrometry was performed using Waters Quattro Premier XE™ (Milford, MA) composing ESI and APCI ionization source with positive and negative ions scanning simultaneously. The multiple reaction monitoring (MRM) scanning mode was used.
Chromatographic conditions
The analytes were separated with a mobile phase consisting of 0.1% formic acid in water (eluent A) and acetonitrile (eluent B), at a flow rate of 0.20 mL/min. The gradient elution was performed as follows: 0.0–3.0 min, 95–30% A, 5–70% B; 3.0–3.2 min, 30–10% A, 70–90% B; 3.2–3. 5 min, 10–95% A, 90–5% B; and 3.5–5.5 min, 95% A, 5% B. The column temperature was 50 °C and 5 µL sample was injected into the chromatographic system.
Method validation
The supernatants of the biological samples were directly injected into the chromatographic system. Stock solutions were diluted to 5000 ng/mL in water, and further diluted with water to standard solutions. Calibrations curves were performed by injecting the standard concentrated solutions between 0.1 and 2000 ng/mL of GABA, Ach, DA, 5-HT, DOPAC, 5-HIAA, and HVA. Data acquisition was performed by Masslynx V4.1 software (Waters, Milford, MA). The LLOQ defined as the lowest concentration at which both precision and accuracy were evaluated by analyzing different concentrations of the standard mixtures.
Immunohistochemistry
After assessing motor function, three mice from each group were injected intraperitoneally 2% sodium pentobarbital anesthesia. Thoracotomy and exposure of the heart were then performed. Paraformaldehyde (4%) was used for perfusion via the left ventricle, and craniotomy was used to quickly remove the brain. Immunohistochemistry was performed using monoclonal mouse antibody to tyrosine hydroxylase (TH) (1:2000; Chemicon, Temecula, CA). Sections were sequentially incubated with primary anti-TH antibody diluted in PBS for 24 h, followed by 1 h incubation with biotinylated secondary antibody. The avidin biotinylated horseradish peroxidase complex method was used to detect the antigen signal (ABC, Vector Laboratories, Burlingame, CA); 3,3-diaminobenzidinetetrachloride (DAB: Sigma Fast DAB, Sigma, St. Louis, MO) in the presence of hydrogen peroxide was used to stain the product light brown. The results were analyzed by counting the numbers of positive cells in each cover slip at 200× magnifications on an image analysis software.
Western blot analysis
The right striatum was weighed and homogenized with 5-fold volume lysis solution. Samples were then placed in an ice bath, followed by centrifugation at 15 000 × g for 15 min at 4 °C. The supernatant was collected. Protein concentration was determined using the BCA kit. To ensure the total protein concentration of each sample was consistent, an equal quantity of proteins were separated by 12.5% SDS-PAGE, and transferred to a PVDF membrane with 250 mA constant current power for 1 h, and the confining liquid was applied for closing. The membrane was soaked in TBS overnight at 4 °C, incubated with primary antibody followed by TH I (1: 10 000 diluted) and horseradish enzyme labeled with biotin for 1 h. The epitope was visualized by an ECL Western blot diction kit. Densitometry analysis was performed using Image software (Olympus, Center Valley, PA).
Measurement of GSH, SOD, and MDA
Brains without the striatum were homogenized in nine volumes of ice-cold saline, and then centrifuged at 4 °C, 4000 r min−1. The supernatant was homogenized with the appropriate buffer solution for the determination of the specific biochemical index. The activities of SOD, GSH, and MDA (a product of lipid peroxidation) levels were determined with specific commercial kits (Nanjing Jiancheng Bioengineering Institute, Nanjing, China). The Coomassie brilliant blue Kit was used for protein measurement.
Statistical analysis
Results were reported as means ± SEM. The data were evaluated by one-way analysis of variance (ANOVA, SPSS16.0, SPSS Inc., Chicago, IL). LSD analysis was used to compare the differences between groups. The significance level for the tests was p < 0.05.
Results
PLA treatments increased movement and distance
The open field test is a commonly used method which can accurately reflect the behavioral activity after MPTP treatment. Application of MPTP at the 21st day led to a significantly lower field of motion distance compared with the control group (p < 0.05) (). The results indicated that intraperitoneal injection of MPTP mice may induce bradykinesia and behavioral abnormality. Furthermore, the total distance in the treatment group was significantly increased by day 21 (p < 0.05). Statistical analysis showed that each treatment group could effectively alleviate bradykinesia and dysfunction caused by MPTP (). By day 14, the total time it took mice to walk the total distance was significantly lower in the MPTP-treated group compared with the control (p < 0.05) (). The total movement time of mice in each treatment group increased significantly, which indicated that the treatment group can effectively alleviate MPTP-induced bradykinesia and dysfunction (). PLA treatment was able to sustainably reverse the effects observed in all PLA treatment groups.
Figure 2. Behavioral performance tested in open-field test. (A1) Total distance of different groups of mice in the open field from 1 d to 28 d (*p < 0.05 versus control; #p < 0.05 versus model). (A2) Total distance of different groups of mice in the open field from 35 d to 56 d (*p < 0.05 versus control; #p < 0.05 versus model). (B1) Total time of different groups of mice in the open field from 1 d to 28 d (s) (*p < 0.05 versus control; #p < 0.05 versus model). (B2) Total time of different groups of mice in the open field from 35 d to 56 d (s) (*p < 0.05 versus control; #p < 0.05 versus model). Data are expressed as mean ± SD (NModel group = 14, NOther groups = 12).
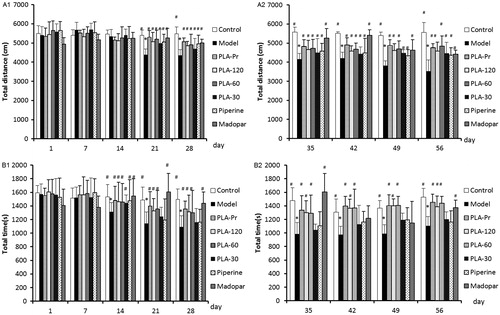
PLA treatments increased DA levels in substantia nigra
The metabolites identified by UPLC-MS/MS analysis to determine neurotransmitter content upon MPTP treatment are shown in . Compared with the control group, the levels of DA and its metabolites were significantly lower in the treatment group (p < 0.01), while HVA levels were not significantly different between the groups. Levels of 5-HT were also significantly reduced in the control group, but its metabolite, 5-HIAA, was significantly increased. The MPTP-treated group also had significantly higher levels of GABA when compared with the control group, while the Ach content remains unchanged.
Table 1. Transmitter contents (ng/g wet brain tissue; mean ± SD) in the striatum analyzed by UFLC-ESI-MS/MS.
PLA- and piperine-treated groups were able to raise the DA levels to different degrees. Furthermore, PLA-Pr, PLA-120, PLA-60, and piperine-treated groups increased DOPAC levels, while administration of Madopar had no significant effects. No significance differences in the HVA content were observed in each of the treatment group. Levels of 5-HT were increased in preventative and Madopar-treated groups, while PLA-120- and piperine-treated groups decreased the 5-HIAA content. PLA-Pr-, PLA-30-, and piperine-treated groups also reduced GABA levels.
Mice treated with MPTP led to significant decreases in TH positive neurons in the substantia nigra. PLA and piperine treatments were able to effectively attenuate the affects of MPTP on dopaminergic neurons injury, while Madopa treatment did not have any effect (). The loss of dopaminergic neurons was assessed by Western blot analysis of tyrosine hydroxylase (TH) expression in the striatum. The striatal TH protein content was decreased after MPTP treatment (). PLA was able to attenuate the MPTP-induced reduction in TH levels, suggesting that PLA might inhibit the injury to dopaminergic neurons.
Figure 3. (A) The photographs of the protective effects of PLA on the MPTP-induced loss of tyrosine hydroxylase (TH)-immunoreactive neurons in the substantia nigral pars compacta (SNpc). (a1 and a2) The control group (n = 12); (b1 and b2) the model group (n = 14); (c1 and c2) the PLA prevention group (n = 12); (d1–f2) PLA (120, 60, and 30 mg/mL) treated plus MPTP-injected groups (n = 12); (g1 and g2) piperine (60 mg/mL) treated plus MPTP-injected group (n = 12); (h1 and h2) the Madopar group (n = 12). (B) The number of TH-immunoreactive neurons in the SNpc of the different groups (*p < 0.05 and **p < 0.01 versus control; #p < 0.05 and ##p < 0.01 versus model).
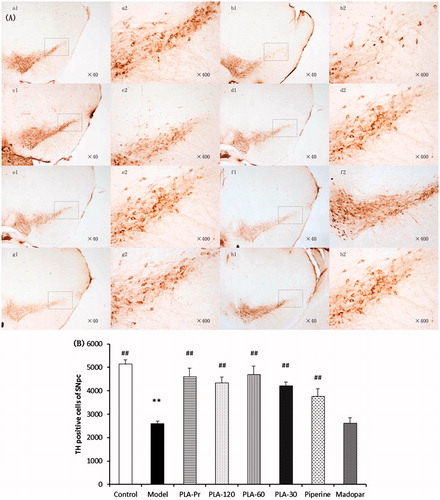
PLA treatment decreased MDA levels
To understand the mechanisms underlying the protective effects of PLA, the activities of SOD and GSH and the MDA contents in the striatum of mice of all treatment were measured. The effects of each treatment on the levels of oxidative stress are shown in . Compared with the normal group, GSH levels in the striatum of MPTP-treated mice were significantly reduced. Furthermore, mice treated with PLA and piperine significantly increased their GSH levels compared with the control group (p < 0.01). However, GSH levels were not affected in Madopa-treated mice (). After MPTP challenge, SOD activity was decreased in the control group, suggesting that antioxidant activity is reduced in PD mouse brain. In PLA-Pr, PLA-60, PLA-30, and piperine-treated groups, SOD activity was enhanced compared with the control group (p < 0.05) (). In addition, compared with the normal group, the content of MDA of the treated group was significantly elevated, suggesting that lipid peroxidation of MDA was increased after treating with MPTP. PLA and piperine treatments significantly decreased the levels of MDA (p < 0.05). The MDA levels of Madopa-treated group were higher than that of the control group. Overall, these results indicate that PLA and piperine can significantly decrease the MDA levels ().
Discussion
Over the past decade, few breakthroughs in the therapeutic intervention of PD have occurred, although advances in the understanding of oxidative stress leading to PD have significantly progressed (Wang et al., Citation2014). However, clinical drugs with few side effects are of high priority and urgently needed. In the present study, the protective effects of PLA against MPTP-induced dopaminergic neuronal cell death in a mouse model of PD were evaluated in vivo. The study provided some evidences that PLA protected dopaminergic cells from MPTP neurotoxicity by ameliorating behavioral abnormalities, increasing levels of DA and its associated metabolites, and enhancing antioxidative defense by increasing GSH level and SOD activity, and decreasing MDA levels. The 1-methyl-4-phenyl-1,2,3,6-tetrahydropyridine (MPTP)-induced PD model which can cause human and animal PD symptoms is the most commonly used mouse model for studying PD (Wang et al., Citation2014). MPTP can selectively damage nigral compacta dopaminergic neurons (Sowell et al., Citation2009). As the male C57BL/6 strains of mice were most sensitive to MPTP, they can better reproduced the pathogenesis of PD (Bernard et al., Citation2008). In this study, 8–12 weeks old male C57BL/6 mice had impaired mobility after 5 d of MPTP treatment. The open field test is the most commonly used method to measure alterations of behavioral activity after MPTP, and this led to a marked decrease in locomotion. MPTP-treated mice also depleted DA in the striatum. The gastric irrigation of PLA (30, 60, and 120 mg/kg) could improve movement impairment, which was associated with more numbers of rearing, longer distances, and shortened duration of immobility time ( and B2). Moreover, TH is the rate-limiting enzyme in the synthesis of catecholamine neurotransmitters such as dopamine. TH activity is progressively decreased following the loss of dopamine neurons in the substantia nigra in PD patients (Zhu et al., Citation2012). Our results showed that PLA reduced the loss of TH-positive neurons in substantia nigra of MPTP mice in vivo. These results suggested that PLA could protect dopaminergic neurons against MPTP-induced toxicity.
One of the primary pathologies of PD is the loss of dopaminergic neurons with a decline in DA and DA-associated metabolites. In this study, UPLC-MS/MS was used to perform neurochemical analysis. A limitation of this method is that the molecular weight of neurotransmitters is small and the polarity is high. Therefore, it is difficult to retain these metabolites in the chromatographic column. Using HPLC-MS/MS provides specific structural information with high sensitivity, fast separation speed, and is simple and quick. Therefore, this method has been increasingly used for different cerebral neurotransmitter quantitative analysis (Carrozzo et al., Citation2010; González et al., Citation2011). A recent study also used HPLC-MS/MS to simultaneously determine a number of rat brain neurotransmitters (Zhu et al., Citation2011). This method has not been used to determine the metabolites found in an animal model of neuronal injury. Therefore, we established a sensitive and fast method with optimal selectivity to detect up to three neurotransmitters simultaneously in the MPTP-induced chronic mouse model.
To further investigate the underlying mechanism of PLA, the total antioxidative capacity was measured. Oxidative stress can be caused by mismatches between the production of free radicals and the ability of the cell to defend against them. Dopaminergic neurons in PD are especially vulnerable to oxidative stress (Barnham et al., Citation2004; Jenner, Citation2007; Prakash et al., Citation2010; Wang et al., Citation2014). MDA is an end product of lipid peroxidation (LPO), which is a measure of free radical generation. LPO may be enhanced by depletion of the GSH content in the brain, which is an endogenous antioxidant often considered as the first line of defense against oxidative stress (Naghizadeh et al., Citation2013). SOD and GSH play an important role in the protection of cells against oxidative stress, and it can thus help prevent neuronal cells from apoptosis (Dringen Citation2000). Hydrogen peroxide (H2O2) is a potent and toxic molecule found in the brain. Normally, any superoxide anion radical (O2−) generated in the brain is first converted into H2O2 that is catalyzed by SODs. GSH eliminates H2O2 and organic peroxides by GSH-Px and converts H2O2 into water as the final product (Khan et al., Citation2012; Kumar et al., Citation2012). In our study, the intraperitoneal injections of MPTP significantly decreased the activities of antioxidant enzyme such as SOD and GSH in the SNc. PLA treatment significantly increased SOD and GSH activity in the SNc compared with untreated mice. Antioxidants might contribute to the beneficial effects of PLA in MPTP-intoxicated mice by restoring SOD and GSH-Px activities and reducing the MDA content. Other mechanisms may be involved for the observed neuroprotective effects of PLA in animal models of PD. Based on our current observations and what has been reported in the literature, P. longum and its major active component, piperine, exhibits neuroprotective effects.
Moreover, our results provided insight into the effective doses of PLA based on its LD50 value. Comparing the activities of PLA with its major active component piperine, we found that PLA had better neuroprotective effects than piperine. The LD50 value of piperine by intragastric administration is 330 mgkg−1 (Piyachaturawat et al., Citation1983), whereas PLA is 1509 mgkg−1, which indicated that PLA is safer with lower toxicity. Pharmacokinetic experiments also demonstrated that the oral bioavailability of PLA is higher than piperine (Liu et al., Citation2011). Therefore, we speculate that PLA may have pleiotropic effects.
Conclusion
In summary, we show for the first time that the alkaloid of P. longum (PLA) has neuroprotective effects in an MPTP-induced chronic mouse model of PD. We further determined the effects of PLA on open-field performance, biochemistry index, DA and metabolic products content, numbers of TH positive neuron, and content of TH. The underlying mechanism of PLA might be associated with antioxidation. Therefore, we propose that PLA might be a potential alternative and complementary medicine used in the treatment of PD. Future studies are needed to further elucidate the detailed mechanisms by which PLA exert its neuroprotective effects.
Declaration of interest
The authors report that they have no conflicts of interest. The authors alone are responsible for the content and writing of this article. This work was supported by National Natural Science Foundation of China (Nos. 81073016 and 81473333), Funding Project for Academic Human Resources Development in Institutions of Higher Learning Under the Jurisdiction of Beijing Municipality (KM 201010025013, PHR201008402, and PHR 200907113) and Key Laboratory of brain diseases in Beijing (2014SJZS04).
References
- Barnham KJ, Masters CL, Bush AL. (2004). Neurodegenerative diseases and oxidative stress. Nat Rev Drug Discov 3:205–14
- Bernard S, Romain Z, Patrick A. (2008). Viral vectors, animal models and new therapies for Parkinson’s disease. Parkinsonism Relat Disord 14:169–71
- Bi Y, Wu X, Chen XQ. (2011). Progress in chemical constituents and pharmacology of Piper longum L. Chin Pharm J 46:1697–700
- Carrozzo MM, Cannazza G, Pinetti D, et al. (2010). Quantitative analysis of acetylcholine in rat brain microdialysates by liquid chromatography coupled with electrospray ionization tandem mass spectrometry. J Neurosci Methods 194:87–93
- Ciobica A, Olteanu Z, Padurariu M, Hritcu L. (2012). The effects of pergolide on memory and oxidative stress in a rat model of Parkinson’s disease. J Physiol Biochem 68:59–69
- Dickson DW, Fujishiro H, Orr C, et al. (2009). Neuropathology of non-motor features of Parkinson disease. Parkinsonism Relat Disord 15:S1–5
- Dringen R. (2000). Metabolism and functions of glutathione in brain. Prog Neurobiol 62:649–71
- Garrett MC, Rosas MJ, Simoes F, et al. (1998). Does timing and dosage of levodopa modify drug-induced dyskinesias andmotor fluctuations? A retrospective analysis. Parkinsonism Relat Disord 4:99–102
- Gaur V, Bodhankar SL, Mohan V, Thakurdesai PA. (2013). Neurobehavioral assessment of hydroalcoholic extract of Trigonella foenum-graecum seeds in rodent models of Parkinson’s disease. Pharm Biol 51:550–7
- González RR, Fernández RF, Vidal JLM, et al. (2011). Development and validation of an ultra-high performance liquid chromatogra-phytandem mass-spectrometry (UHPLC–MS/MS) method for the simultaneous determination of neurotransmitters in rat brain samples. J Neurosci Methods 198:187–94
- Hu SQ, Han RW, Mak SH, Han YF. (2011). Protection against 1-methyl-4-phenylpyridi-nium ion (MPP+)-induced apoptosis by water extract of ginseng (Panax ginseng C. A. Meyer) in SH-SY5Y cells. J Ethnopharmacol 135:34–42
- Jiang ZY, Liu WF, Zhang XM, et al. (2013). Anti-HBV active constituents from Piper longum. Bioorg Med Chem Lett 23:2123–7
- Khan MM, Raza SS, Javed H, et al. (2012). Rutin protects dopaminergic neurons from oxidative stress in an animal model of Parkinson's disease. Neurotox Res 22:1–15
- Kim IS, Koppula S, Park PJ, et al. (2009). Chrysanthemum morifolium Ramat (CM) extract protects human neuroblastoma SH-SY5Y cells against MPP+-induced cytotoxicity. J Ethnopharmacol 126:447–54
- Kim JS, Kim JM, O JJ, Jeon BS. (2010). Inhibition of inducible nitric oxide synthase expression and cell death by (−)-epigallocatechin-3-gallate, a green tea catechin, in the 1-methyl-4-phenyl-1,2,3,6-tetrahydropyridine mouse model of Parkinson’s disease. J Clin Neurosci 17:1165–8
- Kumar H, Lim HW, More SV, et al. (2012). The role of free radicals in the aging brain and Parkinson's disease: Convergence and parallelism. Int J Mol Sci 13:10478–504
- Jenner P. (2007). Oxidative stress and Parkinson's disease. Handb Clin Neurol 83:507–20
- Lee CS, Han ES, Kim YK. (2006). Piperine inhibition of 1-methyl-4-phenylpyri-dinium-induced mitochondrial dysfunction and cell death in PC12 cells. Eur J Pharmacol 537:37–44
- Liu HL, Luo R, Chen XQ, et al. (2013). Tissue distribution profiles of three anti-Parkinsonian alkaloids from Piper longum L. in rats determined by liquidchromatography–tandem mass spectrometry. J Chromatogr B 928:78–82
- Liu JH, Bi Y, Luo R, Wu X. (2011). Simultaneous UFLC-ESI-MS/MS determination of piperine and piperlonguminine in rat plasma after oral administration of alkaloids from Piper longum L.: Application to pharmacokinetic studies in rats. J Chromatogr B 879:2885–90
- Ma J, Shaw VE, Mitrofanis J. (2008). Dose melatonin help save dopaminergic cells in MPTP-treated mice? Parkinsonism Relat Disord 13:1–8
- Mu X, He GR, Yuan X, et al. (2011). Baicalein protects the brain against neuron impairments induced by MPTP in C57BL/6 mice. Pharmacol Biochem Behav 98:286–91
- Naghizadeh B, Mansouri MT, Ghorbanzadeh B, et al. (2013). Protective effects of oral crocin against intracerebroventricular streptozotocin-induced spatial memory deficit and oxidative stress in rats. Phytomedicine 20:537–42
- Pattarini R, Rong Y, Qu C, Morgana JI. (2008). Distinct mechanisms of 1-methyl-4-phenyl-1, 2, 3, 6-tetrahydropyrmidine resistance revealed by transcriptome mapping in mouse striatum. Neuroscience 155:1174–94
- Piyachaturawat P, Glinsukon T, Toskulkao C. (1983). Acute and subacute toxicity of piperine in mice, rats and hamsters. Toxicol Lett 16:351–9
- Prakash B, Shukla R, Singh P, et al. (2010). Efficacy of chemically characterized Piper betle L. essential oil against fungal and aflatoxin contamination of some edible commodities and its antioxidant activity. Int J Food Microbiol 142:114–19
- Schneider JS, Gonczi H, Decamp E. (2003). Development of levodopa-induced dyskinesias in Parkinsonian monkeys may depend upon rate of symptom onset and/or duration of symptoms. Brain Res 990:38–44
- Sowell RA, Owen JB, Butterfield DA. (2009). Proteomics in animal models of Alzheimer’s and Parkinson’s diseases. Ageing Res Rev 8:1–17
- Wang XL, Xing GH, Hong B, et al. (2014). Gastrodin prevents motor deficits and oxidative stress in the MPTP mouse model of Parkinson's disease: Involvement of ERK1/2-Nrf2 signaling pathway. Life Sci 114:77–85
- Xu CL, Qu R, Zhang J, et al. (2013). Neuroprotective effects of madecassoside in early stage of Parkinson's disease induced by MPTP in rats. Fitoterapia 90:112–18
- Zhu KY, Fu Q, Leung KW, et al. (2011). The establishment of a sensitive method in determining different neurotransmitters simultaneously in rat brains by using liquid chromatography-electrospray tandem mass spectrometry. J Chromatogr B 879:737–42
- Zhu Y, Zhang J, Zeng Y. (2012). Overview of tyrosine hydroxylase in Parkinson's disease. CNS Neurol Disord Drug Targets 11:350–8