Abstract
Context: Annona crassiflora Mart. (Annonaceae) is a medicinal plant that is widely used in folk medicine, which leads to its investigation as a potential source of new pharmacological principles.
Objective: This study describes the anti-inflammatory, antiallodynic, and antimutagenic/chemopreventive activities of the leaves A. crassiflora methanolic extract. Its antimutagenic mode of action was analyzed in a plant or animal experimental model.
Materials and methods: Total flavonoids were quantified by spectrophotometry at 415 nm and its composition was analyzed by 1H NMR spectra. Animals received orally, 30, 100, and 300 mg/kg of extract in both tests, carrageenan-induced paw edema and myeloperoxidase activity. Animals were treated with 100 and 300 mg/kg, in all the analyzed tests, pleural cell migration and protein exudation, carrageenan-induced cell migration into the pouch, induction of joint inflammation and carrageenan-induced allodynia response in the mouse paw. To evaluate the antimutagenic/chemopreventive activity through the Allium cepa test, we used 5, 10, and 15 mg/L of extract, and for the micronucleus test in the peripheral blood, we used the dose of 15 mg/kg.
Results: The fractionation of the ethyl acetate (EA) fraction, resulting from the partition of the methanol extract of the A. crassiflora, afforded through chromatographic methods resulted in the isolation of kaempferol 3-O-β-glucoside and kaempferol 3-O-β-diglucoside. Oral treatment with 100 and 300 mg/kg of extract significantly inhibited the carrageenan-induced edema formation, with inhibitions of 53 ± 7% and 47 ± 10%; in MPO activity, the observed inhibitions were 60 ± 7% for 100 mg/kg treatment and 63 ± 7% for 300 mg/kg. The ACME reduced significantly the total leukocytes (an inhibition of 78 ± 9% with 100 mg/kg and 90 ± 7% with 300 mg/kg) and protein levels (approximately 100% inhibition with both doses) in the pleurisy model. In carrageenan-induced leukocyte migration into the pouch, the extract inhibited leukocyte migration only when administered 300 mg/kg per dose (the reduction was 43 ± 5%). Pretreatment with extract failed to reduce the zymosan-induced edema formation and did not inhibit the carrageenan-induced mechanical allodynia. Damage reduction in Allium cepa tested with different concentrations (5, 10, and 15 mg/L) was 66.17, 75.75, and 69.19% for the pre-treatment; 72.72, 33.33, and 22.22% for the simple simultaneous treatment; 100.50, 93.93, and 102.52% for the simultaneous treatment with pre-incubation; 89.39, 79.79, and 84.34%; for the post-treatment, and 86.36, 81.31, and 93.43% for the continuous treatment. The antimutagenic evaluation in the micronucleous test showed a damage reduction of 75.00 and 64.58% for the pre-treatment and simultaneous protocols, respectively. The post-treatment protocol increased the cyclophosphamide effects in 45.83%.
Conclusion: These results suggest that this medicinal plant has chemopreventive and anti-inflammatory therapeutic potential.
Introduction
Tissue lesions usually develop into inflammation, which can cause pain as the edema (formed by increased vascular permeability and extravasation of plasma rich in proteins) compresses the nerve endings. The pain may also be caused by increased sensitivity stimulated by chemical mediators (Kantarci et al., Citation2005; Kumar et al., Citation2006; Medzhitov, Citation2010).
The resolution of inflammatory process requires the recruitment of specific cells, an increased rate of cell division, and the production of inflammatory mediators. The excess of these mediators leads to apoptosis inhibition. This phenomenon is particularly important for elimination of cells with genetic damage, hence the maintenance of this contribute to the development of cancer (Barreto et al., Citation2011).
According to the World Health Organization (WHO), it is estimated that there will be approximately 27 million new cases of cancer worldwide in 2030, and this estimate stimulates the search for natural compounds that can prevent mutagenesis, carcinogenesis, and inflammation and for tests that allow the rapid, safe, and low-cost screening of substances with these potentials.
Natural products are candidates for these tests because the insertion of food compounds or phytopharmaceuticals may be an important alternative for the treatment of inflammation and the prevention of cancer in countries that do not have sufficient resources to address these important public health issues.
The genus Annona L. (Annonaceae) is empirically used for the treatment of inflammatory disorders, and our research group showed that extracts, fractions, and isolated compounds are anti-inflammatory natural agents (Formagio et al., Citation2013a,Citationb). Fruits from this genus are widely used in the traditional herbal medicine, such as for herbal healing; however, their toxic and pharmacological effects on inflammatory states have not been scientifically investigated (Roesler et al., Citation2010).
Annona crassiflora Mart. is widely used in folk medicine. Its leaves, bark, fruits, and seeds are used as stimulant, astringent, antisyphilitic, and antidiarrheal agents and also as antivenin (Corrêa, Citation1926; Cruz, Citation1979; Suleiman et al., Citation2008; Vilar et al., Citation2011). In addition, many other species of the same family are traditionally employed as ethno-medicaments against cancer (Cochrane et al., Citation2008; Fedel-Miyasato et al., Citation2014; Graham et al., Citation2000; Vilar et al., Citation2011).
Therefore, the present study evaluates the antimutagenic/chemopreventive, anti-inflammatory, and antiallodynic activities of the A. crassiflora methanolic extract (ACME) and describes its antimutagenic mode of action in a plant and animal experimental model.
Materials and methods
Plant material and preparation of the Annona crassiflora methanolic extracts
The leaves of A. crassiflora were collected from Areira farm, which is located in the Brazilian cerrado of Mato Grosso do Sul state (Dourados, MS, Brazil), in November 2010. The city of Dourados is located at 22°14′16″S and 54°48′02″W and at an average altitude of 452 m. The species was identified by Prof. Dr. Zefa Valdevino Pereira of the Department of Biological and Environmental Sciences of the Federal University of Grande Dourados (Universidade Federal da Grande Dourados, UFGD). A voucher specimen was deposited at the herbarium of this university under the registration number DDMS 4596.
The dried and ground leaves (820 g) were subjected to maceration in methanol at room temperature. The evaporation of the solvent under vacuum in a rotary evaporator and its subsequent lyophilization yielded the methanolic extract (58 g).
Determination of total flavonoids
A volume of 1.50 mL of 95% ethanol, 0.10 mL of 10% aluminum chloride (AlCl3·6H2O), 0.10 mL of sodium acetate (NaC2H3O2·3H2O) (1 mol/L), and 2.80 mL of distilled water were added to 500 µL of the extract. The mixture was allowed to react at room temperature for 40 min and was read using a spectrophotometer at a wavelength of 415 nm. The same procedure was used to analyze the blank (Lin & Tang, Citation2007). To calculate the concentration of flavonoids, an analytical curve was prepared (2.5, 5.0, 10.0, 20.0, 25.0, 50.0, 100.0, and 125.0 µg) using quercetin as the standard, and the respective absorbances were measured. These data were analyzed through linear regression, and a straight-line equation was obtained and used for the analysis of the real samples. The results are expressed in mg of quercetin equivalents per gram of extract. The quercetin curve equation was y = 12.341x + 0.009, and its correlation coefficient was R = 0.9977. All tests were performed in triplicate.
Extraction and isolation of constituents
Part of the extract (29.74 g) was dissolved in a 1:1 mixture of MeOH/H2O (200 mL) and successively extracted with hexane (3 × 50 mL), CHCl3 (3 × 50 mL), and EtOAc (3 × 50 mL). After evaporation of the solvents on a rotary evaporator, the hexane (H: 4.07 g), chloroform (C: 3.54 g), ethyl acetate (EA: 4.89 g), and hydromethanol (MA: 1.24 g) fractions were obtained. The resulting fraction of the partition into ethyl acetate (EA) was subjected to successive silica gel column chromatography (EtOAc and EtOAc/MeOH in increasing polarity) and Sephadex (H2O, 1:1 H2O/MeOH and MeOH). The 1H NMR spectra of the subfractions obtained in greater quantity showed the presence of flavonoids 1 and 2 as the major components.
Kaempferol 3-O-β-glucoside (1): 1H NMR δH (300 MHz, CD3OD): δ (H, mult., J in Hz, int.): 6.19 (H-6, d, J = 2.1 Hz, 1H), 6.42 (H-8, d, J = 2.1 Hz, 1H), 8.06 (H-2′, H-6′, d, J = 8.7 Hz, 2H), 6.85 (H-3′, H-5′, d, J = 8.7 Hz, 2H), 5.38 (H-1″, d, J = 7.8 Hz, 1H); NMR 13C/DEPT δc (75.5 MHz, CD3OD): 156.5 (C-2, C), 133.4 (C-3, C), 177.8 (C-4, C), 161.5 (C-5, C), 98.9 (C-6, CH), 164.4 (C-7, C), 93.9 (C-8, C), 156.6 (C-9, C), 104.2 (C-10, C), 121.3 (C-1′, C), 131.3 (C-2′, CH), 115.4 (C-3′, CH), 160.2 (C-4′, C), 115.4 (C-5′, CH), 131.3 (C-6′, CH), 101.9 (C-1″, CH), 71.4 (C-2″, CH), 73.4 (C-3″, CH), 68.2 (C-4″, CH), 76.1 (C-5″, CH), 60.4 (C-6″, CH2).
Kaempferol 3-O-β-diglucoside (2): 1H NMR δH (300 MHz, CD3OD): δ (H, mult., J in Hz, int.): 6.45 (H-6, d, J = 2.1 Hz, 1H), 6.78 (H-8, d, J = 2.1 Hz, 1H), 7.78 (H-2′, H-6′, d, J = 8.7 Hz, 2H), 6.90 (H-3′, H-5′, d, J = 8.7 Hz, 2H), 5.29 (H-1″, d, J = 7.5 Hz, 1H), 5.15 (H-1′″, d, J = 7.8 Hz, 1H); NMR 13C/DEPT δc (75.5 MHz, CD3OD): 158.0 (C-2, C), 134.7 (C-3, C), 178.1 (C-4, C), 161.1 (C-5, C), 99.7 (C-6, CH), 161.9 (C-7, C), 94.8 (C-8, C), 156.3 (C-9, C), 106.0 (C-10, C), 120.5 (C-1′, C), 131.0 (C-2′, CH), 115.6 (C-3′, CH), 160.3 (C-4′, C), 115.6 (C-5′, CH), 131.0 (C-6′, CH), 95.6 (C-1″, CH), 73.9 (C-2″, CH), 78.6 (C-3″, CH), 71.2 (C-4″, CH), 78.3 (C-5″, CH), 62.3 (C-6″, CH2), 102 (C-1′″, CH), 70.5 (C-2′″, CH), 70.4 (C-3′″, CH), 71.7 (C-4′″, CH), 74.0 (C-5″, CH), 62.6 (C-6′″, CH2).
Evaluation of anti-inflammatory and antiallodynic activities
Experiments were conducted using male and female Swiss mice (20–30 g), maintained on a 12 h light/dark cycle in a room kept at a constant temperature of 22 ± 1 °C, controlled humidity (60–80%) with food and water provided ad libitum. The animals were acclimatized to the experimentation room for at least 2 h before testing and were used only once throughout the experiments. All experimental procedures were carried out in accordance with the guidelines of the U.S. National Institute of Health and were approved for research by the Ethics Committee on Laboratory Animals of UFGD sob o protocol no. 005/2010. Carrageenan was purchased from Sigma Chemical Co. (St. Louis, MO). Other drugs and reagents were of analytical grade.
Carrageenan-induced paw edema
Different groups of mice were orally treated with ACME (30, 100, and 300 mg/kg), or with dexamethasone by subcutaneous route (1 mg/kg) or vehicle. After 1 h, the animals received a 50 μL subcutaneous (s.c.) injection of carrageenan (300 µg/paw), into the right hindpaw, suspended in sterile 0.9% saline. The contralateral paw received only saline and was used as the control. The paw volume was measured using a digital plethysmometer at different time points (0.5, 1, 2, and 4 h) after the injection of carrageenan. Results were expressed in μm and the difference between basal and post-injection values was quantified as edema (Kassuya et al., Citation2009).
Determination of myeloperoxidase activity
To investigate whether oral treatment with ACME or vehicle could affect the cellular migration induced by carrageenan, the myeloperoxidase activity was measured into the mouse paw following experiments described in carrageenan-induced paw edema. Animals were euthanized 4 h after carrageenan injection. For myeloperoxidase (MPO) activity, the paw skins were homogenized in 5% (w/v) of 80 mM phosphate buffer, pH 5.4, containing 0.5% of hexadecyltrimethylammonium bromide. The homogenate was centrifuged at 3200 rpm and 4 °C for 20 min. Aliquots (30 μL) of each supernatant were mixed with 100 μL of phosphate buffer 80 mM, 85 μL of phosphate buffer 0.22 mol/L, and 15 μL of 0.017% H2O2 on a 96-well plate. The reaction was triggered with 20 μL of 3,3,3-tetramethylbenzidine (dissolved in N,N-dimethylformamide). The plate was kept at 37 °C for 3 min, after which the reaction was stopped by adding 30 μL of sodium acetate 1.46 mol/L, pH 3.0. The enzymatic activity was determined by measuring the optical density at 630 nm and it was expressed as mOD per mg of protein.
Pleural cell migration and protein exudation
Different groups of animals treated with ACME (100 and 300 mg/kg, per oral) or dexamethasone (1.0 mg/kg, subcutaneously, positive control), vehicle, and naive (0.9% negative control) were administered orally by gavage, in different groups of mice. Pleurisy was induced by the intrathoracic injection of 100 µL of 1% carrageenan as previously described (Henriques et al., Citation1990). The carrageenan was diluted in saline buffered. Briefly, an adapted needle was inserted into the right side of the thoracic cavity of the animals to enable intrathoracic (i.t.) administration of carrageenan. Control mice received an equal volume (100 µL) of sterile, pyrogenfree saline. After 4 h, the animals were killed and the thoracic cavity was washed with 1 mL of phosphate-buffered saline (PBS). The exudate volume was measured at room temperature, and an aliquot of 20 μL was diluted in the Turk solution (1:20) and used to determine the total number of leukocytes in a Neubauer chamber. For differential counting of leukocytes, the remaining fluid was centrifuged at 3200 rpm for 20 min, and the cells were resuspended. The protein exudation was evaluated directly from the lavage by Bradford’s reaction, using the commercially available Bradford kit (Bioagency, São Paulo, Brazil). Total and differential cell counts were performed under light microscopy and the results are reported as the number of cells per ml of pleural fluid.
Carrageenan-induced cell migration into the pouch
Air pouch model of inflammation was performed as described previously (Garcia-Ramallo et al., Citation2002), with some modifications. Mice were briefly anesthetized with cetamine and xylasine and 4 mL of sterile air was injected with a seringle subcutaneously in the back of mice, on day 0. On the 3rd day, a second injection of 2 mL of sterile air was performed into the preformed pouch. On the 6th day, animals were treated with vehicle, dexamethasone (0.5 mg/kg s.c.) as the positive control drug or ACME (100 and 300 mg/kg orally) and 1 h later the inflammatory response was induced by a injection of a solution of carrageenan 0.1% (250 µL) directly into the pouch. Four hours after the inflammatory stimulus, mice were euthanized and the pouches were washed with 2 mL of phosphate-buffered saline (PBS) containing heparin (10 UI/mL). Exudates were collected and total cells were counted on the Neubauer chamber under light microscopy. Exsudates were then centrifuged at 1000 g for 2 min at 4 °C and the pellet suspended in 1 mL of 3% albumin and added to previously prepared slides. Cells were stained with Rosenfeld and analyzed under light microscopy and 100 cells were counted.
Induction of joint inflammation
Groups of mice were orally treated with ACME (100 and 300 mg/kg) or vehicle. Another group of mice was treated subcutaneously with the anti-inflammatory drug dexamethasone (1 mg/kg). Joint inflammation was induced by intra-articular (i.art.) injection of zymosan (500 µg per cavity in 25 µL sterile saline) by inserting a needle through the suprapatellar ligament into the left knee joint cavity, as previously described (Conte et al., Citation2008; Penido et al., Citation2006). Control animals received an intra-articular injection of an equal volume of sterile saline.
Carrageenan-induced allodynia response in the mouse paw
The mechanical threshold with electronic analgesimeter (similar to von Frey hairs analysis) was measured (Chaplan et al., Citation1994; Kassuya et al., Citation2007). The mechanical threshold was expressed in milligrams (mg) and mechanical allodynia (measure after the intraplantar injection of carrageenan – 300 μg/paw) was considered as a decrease in the threshold when compared with the response of the same paws before treatment. Mice were pretreated (60 min before, per oral) with the ACME (100 and 300 mg/kg) and received a 20 μL s.c. injection into the right hind paw of carrageenan (300 μg/paw) suspended in sterile 0.9% saline. Control animals received a similar volume of vehicle (10 mL/kg) by oral route or directly into the paw (20 μL). The mechanical threshold was registered 3–4 h after injection of carrageenan.
Statistical analysis
Data are presented as mean ± S.E. Differences between groups was evaluated by analyses of variance (one-way ANOVA) followed by the Newman–Keuls test. The number of animals per group is indicated in the legends. Statistical differences were considered to be significant at p < 0.05. Asterisks denote significant difference compared with the vehicle-treated group.
Toxicogenetic and chemopreventive evaluations
To induce DNA damage in Allium cepa, methyl methanesulfonate (MMS) at a concentration of 10 mg/L was used. The Annona crassiflora methanolic extract (ACME) diluted in 1% methanolic solution was tested at concentrations of 5, 10, and 15 mg/L.
In the micronucleus assay with mouse peripheral cells, the agent used to induce DNA damage was cyclophosphamide at a dose of 100 mg/kg of corporeal weight (c.w.). This agent was injected intraperitoneally (i.p.), and the ACME, which was diluted in 1% solution, was tested at a concentration of 15 mg/kg c.w. and administered through oral gavage.
Experimental design – Allium cepa test
Commercial seeds of Allium cepa (TOP SED – garden/blue line®; Lot: 020595) were used. These were germinated at room temperature in Petri dishes, covered with filter paper, and soaked with 3 mL of distilled water or solution. The seeds were then subjected to different treatments and protocols to evaluate the mutagenicity and antimutagenicity of the treatment ().
Figure 1. Treatments to evaluate the mutagenicity and antimutagenicity of A. crassiflora methanolic extract (ACME). Groups: negative control = distilled water 120 h; MMS = aqueous solution of methyl methanesulfonate; pre-treatment (48 h ACME + 48 h MMS), simultaneous simple (48 h distilled water + 48 h of association of ACME and MMS), simultaneous with pre-incubation (48 h distilled water + 48 h MMS with ACME pre-incubated for 1 h at 37 °C in the oven), post-treatment (48 h MMS + 48 h ACME), continuous (48 h ACME + 48 h MMS) without washing the seeds.
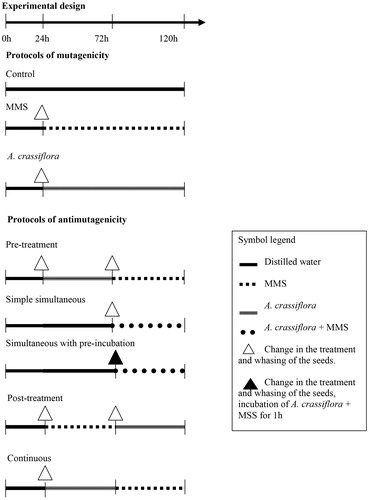
The seeds were subjected to the following treatments for 120 h of cultivation:
Mutagenicity protocols:
(I) Negative control: The seeds were cultivated for 120 h in 1% methanol solution.
(II) Positive control (MMS): The seeds were cultivated in distilled water for the first 24 h and then in dishes containing aqueous MMS solution for a period of 96 h.
(III) ACME: The seeds cultivated in distilled water for the first 24 h and then in ACME solutions (three different concentrations: 5, 10, and 15 mg/L) for 96 h.
Antimutagenicity protocols:
(IV) Pre-treatment: The seeds were cultivated in distilled water for the first 24 h, in the ACME solution for 48 h, and then in a dish containing MMS for 48 h.
(V) Simple simultaneous: The seeds were grown in distilled water for 72 h and then in a cultivation plate containing the MMS and ACME solutions, which were added simultaneously for a period of 48 h.
(VI) Simultaneous with pre-incubation: The MMS and ACME solutions were pre-incubated at 37 °C for 1 h and subsequently transferred to the cultivation plate. The seeds were first germinated in distilled water for 72 h and then germinated in the prepared mixture for 48 h.
(VII) Post-treatment: The seeds were grown in distilled water for 24 h, in a plate containing the aqueous MMS solution for a period of 48 h, and then in a plate containing the ACME for 48 h.
(VIII) Continuous: The seeds were germinated in distilled water for 24 h and then in a plate containing the ACME for 48 h. The aqueous MMS solution was then added to the plate, and the seeds were cultivated in the mixture for 48 h.
Between each of the treatments, before the transfer of the seeds from one plate to the other, with the exception of the continuous phase, the seeds were washed twice in distilled water to remove the compounds from the previous treatments.
Preparation of slides
According to the protocol described by Mauro et al. (Citation2014), which was slightly modified, after 120 h of cultivation, the roots were harvested at noon and subjected to fixative solution (acetic acid:ethanol 3:1) for at least 6 h. Subsequently, the roots were subjected to acid hydrolysis to expose the genetic material (1 N HCl at 60 °C for 6 min). The DNA was then stained with Schiff's reagent for 2 h in the dark. The root tips were cut with a scalpel to extract the meristematic region. The cytoplasmic staining was performed by adding a drop of acetic carmine (2%). The material was then covered with a coverslip, and the meristems were gently crushed with tweezers. For the preparation of permanent slides, the whole slide was soaked in liquid nitrogen to remove the coverslip. Then, with the aid of synthetic resin (Permout®), a new coverslip was placed on the biological material.
Microscopic and statistical analysis
A total of 5000 cells per treatment (1000 cells/slide) were analyzed under a light microscope with 400× magnification. During the analysis, the cells were classified into interphase, prophase, metaphase, anaphase, and telophase. For each of these phases, the number of chromosomal alterations was quantified, and the presence of micronuclei was evaluated.
To obtain the mitotic index (MI), the number of dividing cells (prophase, metaphase, anaphase, and telophase) was divided by the total number of analyzed cells:
The calculation of the reduction percentage in the mitotic index (%RDMI), as suggested by Fiskesjö (Citation1993), was calculated using the following formula:
The percentage of damage reduction (DR%), as suggested by Manoharan and Banerjee (Citation1985) and Waters et al. (Citation1990), was obtained by the following calculation:
The statistical analysis was performed using the Chi-square test, and differences were considered to be statistically significant if p < 0.05.
Experimental design – Swiss mouse
Thirty Swiss female mice (n = 5/group) of reproductive age were used. The mice were obtained from the Animal Facility of the Center for Biological and Health Sciences (Centro de Ciências Biológicas e da Saúde, CCBS) located at the Federal University of Mato Grosso do Sul (Universidade Federal do Mato Grosso do Sul, UFMS), Campo Grande, MS, Brazil. The animals were maintained in propylene boxes covered with wood shavings and fed a commercial diet (Nutrivital®) and filtered water ad libitum. The temperature and light were controlled using 12 h photoperiods (12 h of light/dark) with a temperature of 22 ± 2 °C and a humidity of 55% ± 10 in a ventilated AESLO® shelf (ESLO, Frankfurt, Germany). The experiment was conducted according to the standards of Brazil's National Council for the Control of Animal Experimentation (Conselho Nacional de Controle de Experimentação Animal – CONCEA) and with the approval of the Ethics Committee on Animal Use (CEUA/UFMS) under protocol no. 311/2011.
The animals were treated and the collections of biological material were performed according to the following protocol ():
Control group: On the first and second days, the animals received 0.1 mL/10 g (c.w.; oral gavage) 1% methanol solution and then 0.1 mL/10 g (c.w.; i.p.) saline solution.
Cyclophosphamide group: The animals were administered saline solution and 1% methanol solution on the first day and 1% methanol solution and cyclophosphamide on the second day.
Annona crassiflora methanolic extract: The animals were administered 1% methanol solution and saline solution on the first day and ACME and saline solution on the second day.
Pre-treatment: The animals received the ACME and saline solution on the first day and 1% methanol solution and cyclophosphamide on the second day.
Simultaneous: The animals were administered 1% methanol solution and saline solution on the first day and ACME and cyclophosphamide on the second day.
Post-treatment: The animals received 1% methanol solution and cyclophosphamide on the first day and ACME and saline solution on the second day.
The collection of peripheral blood was performed by puncture of the tail vein 24 h after the administration of the test compounds on the second day.
Micronucleus test in peripheral blood
The protocol described by Hayashi et al. (Citation1990) was used with some modifications (Oliveira et al., Citation2009a). First, 40 μL of peripheral blood were deposited on a pre-coated slide with 20 μL of acridine orange (1.0 mg/mL). A coverslip was then placed over the biological material, and the slide was placed in a freezer (−20 °C) for a minimum period of 7 d. The material was evaluated using a fluorescence microscope (Motic®, BA410FL, Microscopes Plus Ltd., Hertfordshire, UK) at 400× magnification with a 420–490 nm excitation filter and a 520 nm barrier filter.
Two thousand cells/animal were analyzed, and the statistical analysis was performed using ANOVA/Tukey’s test (p < 0.05). The percentage of damage reduction was calculated according to the previous description.
Results
Phytochemistry
The ACME exhibited a high content of flavonoids in 427.30 mg of quercetin/g of sample. The fractionation of the ACME leaves for the identification of possible flavonoids resulted in the isolation of kaempferol 3-O-β-glucoside and kaempferol 3-O-β-diglucoside. The structures of the isolated compounds were elucidated based on the spectroscopic data analysis obtained through 1H and 13C NMR and DEPT, COSY, HMQC, and HMBC experiments.
Evaluation of anti-inflammatory and antiallodynic activities
Effects of oral ACME administration on carrageenan-induced paw edema
The injection of carrageenan into the paw induced edema starting 30 min after administration and peaking 2 h after administration (). Oral treatment with ACME (100 and 300 mg/kg) significantly inhibited the carrageenan-induced edema formation. The inhibition percentages achieved with ACME doses of 100 and 300 mg/kg were 53 ± 7% and 47 ± 10%, respectively (). In addition, the inhibition observed in the dexamethasone-treated group 2 h after the injection of carrageenan into the paw was 44 ± 9% ().
Figure 3. Effect of oral administration of ACME on carrageenan-induced paw edema in mice. Animal received ACME (30, 100, or 300 mg/kg, p.o.), dexamethasone (DEX – 1 mg/kg, s.c.) or vehicle and after 1 h, an intraplantar injection of carrageenan (300 µg/paw) was performed. In (A), the inhibition induced by 30, 100, and 300 mg/kg of ACME and DEX in paw edema (µm) at 4 h after carrageenan injection is shown. In (B), bars show the effect of different doses of 30, 100, and 300 mg/kg of ACME and DEX in increasing of myeloperoxidase (MPO) activity at 6 h after carrageenan injection is shown ACME and DEX. The bars express the mean ± SE of five animals, compared with the vehicle (V) versus the treated group. *p < 0.05, **p < 0.01, one-way ANOVA followed by the Student–Newman–Keuls test.
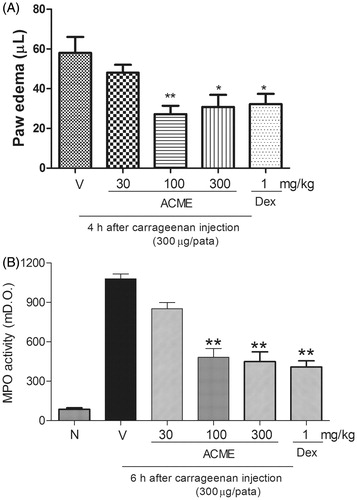
The injection of carrageenan (300 µg/paw) increased MPO activity 6 h after administration; therefore, oral treatment with ACME (100 and 300 mg/kg) altered the increase in MPO activity induced by carrageenan with inhibition percentages of 60 ± 7% and 63 ± 7%, respectively. The positive control (dexamethasone) was able to induce the inhibition of MPO activity compared with the control group () with a reduction of 67 ± 5%.
Effects of ACME on carrageenan-induced pleural cell migration and protein exudation
The results are expressed as leukocytes × 106, and the plasma leakage results are shown as mg of proteins/mL (). The administration of carrageenan into the pleural cavity of the animals induced an increase in the total leukocyte numbers () and an increase in protein extravasation () 4 h after injection. ACME significantly reduced the increase in total leukocytes (inhibition of 78 ± 9% with 100 mg/kg and 90 ± 7% with 300 mg/kg) and the increase in protein levels (approximately 100% inhibition with both doses) induced by the administration of carrageenan into the pleural cavity (). The subcutaneous administration of dexamethasone (1.0 mg/kg, s.c.) 1 h before the administration of carrageenan into the pleural cavity also reduced the increases in total leukocytes (inhibition of 93 ± 4%) and protein levels (100%) induced by carrageenan.
Figure 4. Effects of ACME on total leukocytes (A) and protein extravasation (B) induced by carrageenan in the pleural cavity of mice. Animal received the oral treatment with ACME (100 or 300 mg/kg), or vehicle, and after 1 h, they received an intrapleural injection of Cg (100 μL of a 1% solution/cavity). Control animals received only the vehicles and Naïve animal did not received carrageenan or ACME treatment. Animals were killed after Cg injection. The bars express the mean ± SEM of five animals, compared with the vehicle (V) versus the treated group. **p < 0.01, ***p < 0.001, one-way ANOVA followed by the Student–Newman–Keuls test. #p < 0.001.
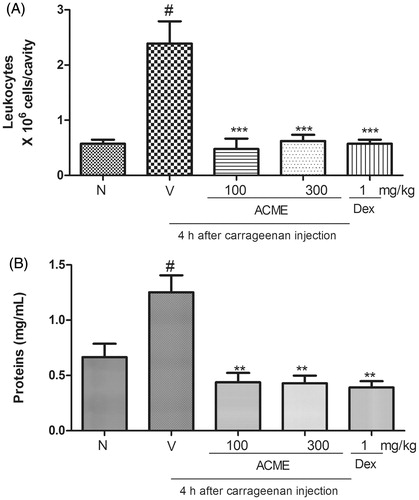
Effect of ACME on carrageenan-induced leukocyte migration into the pouch
The injection of carrageenan into the pouch of sixth-day-old mice promoted plasma leakage (p < 0.001) and the migration of leukocytes, particularly polymorphonuclear leukocytes (). The analysis of variance showed significant differences in the levels of leukocyte migration between the groups. The analysis of the inflammatory activity of ACME (100–300 mg/kg) indicated that this extract inhibited leukocyte migration only when administered at a dose of 300 mg/kg, which resulted in 43 ± 5% inhibition. Dexamethasone, which was used as a positive control, reduced leukocyte infiltration into the pouch by 100% 4 h after carrageenan injection ().
Figure 5. Effect of ACME on carrageenan-induced leukocyte migration and plasma leakage into the air pouch. Mice (n = 5) were pretreated 1 h before with ACME (10–300 mg/kg p.o.), dexamethasone (1 mg/kg, s.c., diluted in saline) or vehicle. Pouches were washed with PBS-containing heparin. Cells were counted and plasma leakage was analyzed. Results are expressed as cell 106/cavity. **p < 0.01, ***p < 0.001, #p < 0.001, compared with the vehicle group. Difference between groups were analyzed by analysis of variance (one-way ANOVA) followed by the Newman–Keuls test.
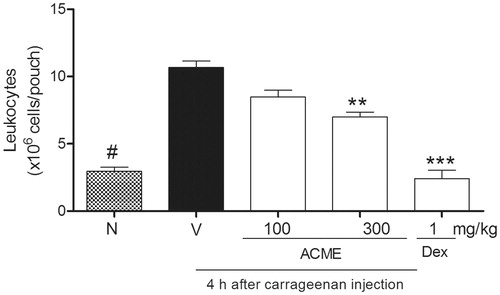
Effects of ACME on zymosan-induced articular inflammatory response
According to previous results (Conte et al., Citation2008), zymosan injection induces a significant articular inflammatory response characterized by marked articular edema formation with a six-fold increase in the knee diameter of the control group compared with the naïve group (Penido et al., Citation2006). The results showed that pre-treatment with ACME (in both doses tested) failed to reduce the zymosan-induced edema formation, whereas the subcutaneous treatment with dexamethasone inhibited edema formation by 59 ± 7%.
Effects of ACME on carrageenan-induced mechanical allodynia
The intraplantar injection of 20 µL of carrageenan (300 µg/paw) induced a reduction of 98 ± 1% in the nociceptive threshold level. The carrageenan-induced mechanical allodynia peaked within 3–4 h after injection. The oral administration of ACME (both doses: 100 and 300 mg/kg) did not inhibit the carrageenan-induced mechanical allodynia.
Toxicogenetic and chemopreventive evaluations
Allium cepa test
The Allium cepa test showed that the ACME is not mutagenic and exhibits significant antimutagenic activity. The damage reduction percentages obtained with concentrations of 5, 10, and 15 mg/L were 66.17, 75.75, and 69.19% for the pre-treatment, 72.72, 33.33, and 22.22% for the simple simultaneous treatment, 100.50, 93.93, and 102.52% for the simultaneous treatment with pre-incubation, 89.39, 79.79, and 84.34% for the post-treatment, and 86.36, 81.31, and 93.43% for the continuous treatment ().
Table 1. Distribution of chromosomal aberrations, MI, MIDR, and DR% in Allium cepa treatments associated to A. crassiflora methanolic extract.
The mitotic indexes for the control and MMS groups were 3.70 and 3.57, respectively. The evaluation of the mutagenicity of ACME revealed mitotic indexes of 3.76, 4.12, and 4.79 for doses of 5, 10, and 15 mg/L, respectively. Soon after the administration, a small reduction percentage in the mitotic index (3.51%) obtained for MMS was obtained in comparison with the control. For ACME, the reduction percentages in the mitotic index were −1.61, −11.35, and −29.46% for concentrations of 5, 10, and 15 mg/L, respectively ().
The antimutagenicity evaluations revealed that the only protocol that resulted in a lower MI compared with the control was the pretreatment protocol with a concentration of 15 mg/L. However, the dose of 5 mg/L resulted in an improvement in the mitotic index for the pre-treatment, simultaneous with pre-incubation, post-treatment, and continuous protocols; the respective mitotic indexes were 4.86, 5.36, 3.79, 5.86, and 5.07, respectively, and the reduction percentages in the mitotic index ranged from −2.43 to −58.38% ().
With the concentration of 10 mg/L, all the protocols showed improvements in the mitotic index. For the pre-treatment, simple simultaneous, simultaneous with pre-incubation, post-treatment, and continuous protocols, the mitotic indexes were 6.07, 4.29, 5.02, 4.49, and 5.03, and the reduction percentages in the mitotic index were −64.05, −15.95, −35.68, −21.35, and −35.95%, respectively ().
At a concentration of 15 mg/L, only the pre-treatment protocol showed a reduced mitotic index value of 2.00, and the reduction percentage in the mitotic index was 45.95%. For the simple simultaneous, simultaneous with pre-incubation, post-treatment, and continuous treatment protocols, the mitotic indices were 4.58, 4.31, 5.06, and 5.35, and the reduction percentages in the mitotic indices were −23.78, −16.49, −36.76, and −44.59%, respectively. These data show that the ACME favors cell division ().
Swiss mouse test
The evaluation of the initial weight, final weight, and weight gain of the experimental animals, as well as the assessment of the absolute and relative weight of the lungs, heart, liver, spleen, and kidneys, showed no statistically significant differences ().
Table 2. Initial, final, and body weight gain of the animals; absolute and relative weights of the organs of animals treated with A. crassiflora methanolic extract and/or cyclophosphamide (mean ± S.E.).
The ACME was not mutagenic, as determined through the mouse test. The antimutagenic evaluation showed damage reduction percentages of 75.00 and 64.58% for the pretreatment and simultaneous protocols, respectively. The post-treatment protocol exhibited a potentiation of the cyclophosphamide effects by 45.83% ().
Table 3. Total frequency, frequency mean ± S.E., percentage of damage reduction related to tests for mutagenicity and antimutagenicity in micronucleus test in peripheral blood of Swiss female mice.
Discussion
The results of the present study confirmed and also largely extended the previous findings by demonstrating that the Annona crassiflora extract exhibits important chemoprevention and antimutagenic activities also when systemically administered. Furthermore, the methanolic extract was found to effectively interfere with the inflammatory response induced by carrageenan, as demonstrated by the effects associated with paw inflammation, myeloperoxidase activity, pleural cell migration, protein exudation, cell migration into the pouch, and induction of joint inflammation. The present findings also provide the first demonstration of the anti-inflammatory action of the methanolic extract and provide some insights into its inability to induce mutagenesis and exhibit antimutagenic activity.
With respect to the extract and the identified compounds of A. crassiflora, only a few studies have investigated their antibacterial, antileishmanial, trypanocidal, larvicidal, molluscicidal, genotoxicity, mutagenic/antimutagenic, and antispasmodic activity (De Omena et al., Citation2007; Dos Santos & Sant'Ana, Citation2001; Mesquita et al., Citation2005; Rodrigues et al., Citation2006; Takahashi et al., Citation2006; Weinberg et al., Citation1993).
The pharmacological pre-clinical studies performed with species, which belong to family Annonaceae, genus Annona, notably A. senegalensis Pers., A. reticulata L., A. muricata L., A. sylvatica A. St.-Hil., and A. dioica A. St.-Hil., have confirmed their folk medicinal properties for the treatment of inflammatory disease (Chavan et al., Citation2010, Citation2011, Citation2012a,Citationb; De Sousa et al., Citation2010; Formagio et al., Citation2013a,Citationb; Yeo et al., Citation2011). Previous studies performed by our research group as well as others have shown that the extract, essential oil, and identified compounds obtained from the leaves and bark of Annona species exert anti-inflammatory and antinociceptive effects (Chavan et al., Citation2010, Citation2011, Citation2012a,Citationb; De Sousa et al., Citation2010; Formagio et al., Citation2013a,Citationb; Yeo et al., Citation2011).
Studies conducted by our group have shown that the methanolic extract of A. sylvatica and the essential oil of A. dioica inhibit inflammatory parameters, such as edema, myeloperoxidase activity, and leukocyte migration (Formagio et al., Citation2013a,Citationb). To the best of our knowledge, the present study provides the first demonstration of the ability of the ACME, which was administered at doses in the range of 30–300 mg/kg through the oral route, to inhibit important inflammatory parameters, such as edema formation, paw inflammation, myeloperoxidase activity, pleural cell migration, protein exudation, and cell migration into the pouch. However, the nature of the active principles responsible for these actions is unclear.
Several models of experimental inflammation have been described and are used to validate natural and synthetic products or compounds. The common substance used is carrageenan, and this compound can be administered in the paw, pleura, and artificial pouches. In the carrageenan-paw, -pleural, and -pouch inflammation models, the oral administration of ACME showed similar efficacy to that observed with the subcutaneous administration of dexamethasone. In the zymosan-induced articular inflammatory model only, dexamethasone and not oral ACME induced efficacy compared with the control group. Most likely the mechanism of action is different from that of steroidal anti-inflammatory drugs and from that of nonsteroidal anti-inflammatory drugs (NSAID) because ACME did not alter the mechanical allodynia induced by carrageenan. Because the compounds contained in ACME are most likely original, and the structural diversity found in the nature could not be discussed in this step of our study, the mechanisms of anti-inflammatory action will be studied in the future.
The chronic inflammatory process obviously contributes to tissue damage and likely DNA damage, as well as a large number of inflammatory diseases. Thus, natural or synthetic agents that inhibits mutagenic and inflammatory process most likely acts through different mechanisms of action. In an attempt to evaluate the effects of the extract in both pathological alterations, there is a necessity to assess different doses for both situations. ACME was shown to be an effective anti-inflammatory agent in doses ranging from 100 to 300 mg/kg, whereas it exhibited chemopreventive effects at a dose of 15 mg/kg. Aspirin and other similar NSAIDS showed anti-inflammatory effects at a dose of 300 mg/kg (Menezes-de-Lima et al., Citation2006), whereas aspirin at doses of 0.5–50 mg/kg is required for micronucleus tests with peripheral blood (Niikawa et al., Citation2001). Thus, the doses that showed anti-inflammation effects and the induction of chemoprevention are equivalent to those found in the literature.
The present study demonstrated that the ACME has no mutagenic activity in either of the experimental models used: Allium cepa and mouse test. This finding was corroborated by Dragano et al. (Citation2010) and Vilar et al. (Citation2008), who demonstrated that neither the pulp nor the ethanol extract of A. crassiflora is mutagenic in mice.
With respect to antimutagenicity, the studies are contradictory for A. crassiflora and do not describe its antimutagenic more of action. To elucidate it, the literature suggests the use of several protocols. In particular, we used the pre-treatment, simple simultaneous, simultaneous with pre-incubation, post-treatment, and continuous protocols for the Allium cepa test and the pre-treatment, simultaneous, and post-treatment protocols for the test in mice. The simple simultaneous and pretreatment protocols indicate both desmutagenic and bio-antimutagenic activity, the simultaneous with pre-incubation protocol indicates desmutagenic activity, and the post-treatment protocol preferentially indicates bio-antimutagenic activity (Antunes & Araújo, Citation2000; De Flora & Ferguson, Citation2005; Fedel-Miyasato et al., Citation2014; Felicidade et al., Citation2014; Ferguson, Citation1994; Flagg et al., Citation1995; Oliveira et al., Citation2006, Citation2007, Citation2009b, Citation2013a,Citationb, Citation2014; Mauro et al., Citation2014, Citation2013; Pesarini et al., Citation2013). The desmutagenic substances chemically or enzymatically inactivate the mutagen in the extra or intracellular medium (De Flora, Citation1998; Ferguson, Citation1994; Hartman & Shankel, Citation1990; Kada & Shimoi, Citation1987; Oliveira et al., Citation2006, Citation2007). Bio-antimutagenic agents are those that modulate the repair and replication of DNA and act at the cellular level by increasing the replication fidelity, stimulating error-free repair, or inhibiting repair systems subject to error (De Flora, Citation1998; Hartman & Shankel, Citation1990; Simic et al., Citation1998).
According to the Allium cepa test, the ACME can exert both desmutagenesis and bio-antimutagenesis activities. This assertion is supported by the high percentage of damage reduction observed with the simultaneous treatment with pre-incubation and post-treatment protocols, which ranged from 93.93 to 102.52% and 79.79 to 89.39%, respectively. In the test with mice, the damage reduction percentages did not confirm the mode of action because the damage reduction percentage obtained with the post-treatment protocol was −45.83%. Thus, it appears that the ACME potentiated the cyclophosphamide effects obtained with this protocol, and the rate of damage increase was approximately 45.83%. This same effect was described by Dragano et al. (Citation2010), who reported an increased rate of micronuclei in the order of 2.32, 61.63, and 60.46% (the calculations were performed by these researchers based on data presented by the study in question) in mice supplemented with 1, 10, and 20% A. crassiflora pulp, respectively.
Another finding that stands out in the Allium cepa data is that the pre-treatment protocol with the smallest dose exhibited an increase in the micronuclei rate of 66.17%. However, this increase was not observed for the same protocol with the other doses. In the test with mice, this protocol showed the best damage reduction percentage (75.00%).
With respect to the potentiation of the effects of the alkylating agent, namely cyclophosphamide, Dragano et al. (Citation2010) and Souza et al. (Citation2012) suggest that these effects occur due to the presence of reducing agents, such as ascorbic acid, in A. crassiflora. Therefore, it is hypothesized that this compound increases the conversion of cyclophosphamide to its active metabolites, thereby increasing the frequency of genomic lesions (micronuclei).
For the simple simultaneous and continuous treatment protocols, through which both desmutagenesis and bio-antimutagenesis can occur, an initial analysis is expected to reveal a sum of the modes of action. However, this was not verified with the Allium cepa test because the damage reduction percentages do not supplant those observed for the simultaneous with pre-incubation treatment protocol, which indicated that only desmutagenesis activity is observed. The evaluation of the simultaneous treatment protocol in mice revealed an intermediate damage reduction percentage, which reinforces the findings obtained with the Allium cepa test.
According to Rank and Nielsen (Citation1997) and Fedel-Miyasato et al. (Citation2014), a comparison of the mutagenicity assays in Allium cepa with tests in rodents shows a concordance of approximately 80%, and therefore allows the confirmation of the antimutagenic mode of action. However, the data obtained in this study do not support this assertion because the post-treatment and simultaneous protocols differ by 130.17 percentage points and 42 percentage points between both modes. Additionally, if analyzed separately, the two models do not indicate the same mode of action mainly due to the inactivity of the post-treatment in the mice model.
The difference in the results between the Allium cepa test and the test in mice can be explained by the differences in the metabolisms of the two organisms. The cytochrome P450 enzyme is responsible for 50% of the metabolism of therapeutic agents, and the comparison of the presence of this enzyme complex leads to the conclusion that plants have a low concentration compared with mammals (Leme & Marin-Morales, Citation2009). Because of this increased metabolism in mammals, it can be hypothesized that the ACME was metabolized more efficiently in mice than in plant, thereby preventing the modulation of the action of enzymes by sufficient amounts of the ACME, which explains the lack of bio-antimutagenic action obtained with the post-treatment protocol.
Fiskesjö (Citation1993) stated that the mitotic index of apical meristems of Allium cepa is considered normal compared with that of chemicals when there is an inhibition of cell division by up to 36%. This researcher also reports that a reduction in mitosis to values greater than 50% reveals that the substance under analysis is toxic to meristematic cells. These findings suggest that the ACME is not toxic to the meristematic culture and that it can improve the mitotic index when associated with MMS, which indicates that the ACME has the ability to modulate events to decrease the toxicity of the alkylating agent.
Based on the reference values reported by Fiskesjö (Citation1993), it is understood that the mitotic index measured through the mutagenicity protocols demonstrates that the ACME has no genotoxic activity. The reduction percentages in the mitotic index were −1.62, −11.35, and −29.46% for concentrations of 5, 10, and 15 mg/L, respectively. Increases in the concentrations resulted in increases in the mitotic index. This finding corroborates the assertion that the substance is not mutagenic even at higher concentrations.
With respect to the antimutagenicity protocols, only the pre-treatment protocol with a concentration of 15 mg/L showed a lower mitotic index with a high rate of decrease in this index (45.95%). It is understood that this protocol (with this concentration) has not proven to be efficient at expressively reducing the toxicity caused by the MMS damage-inducing agent. The other protocols and treatments (at all concentrations) resulted in an increase in the mitotic index, which suggests that ACME is able to reverse the toxicity inducted in cultures by MMS treatment.
Based on the findings, the ACME is considered to have anti-inflammatory therapeutic potential because it is capable of inhibiting paw edema, reducing myeloperoxidase activity, reducing the total leukocyte count and protein extravasation in the pleural cavity, and reducing leukocyte migration and plasma extravasation in the air pouch assay, all of which are effects induced by carrageenan administration. In addition, this extract is not mutagenic in plant and animal models. Moreover, although no correspondence was found between the plant and animal models, as would be expected based on the literature, the ACME has chemopreventive potential and may act mainly through desmutagenesis in mammals. Thus, it is suggested that this medicinal plant has anti-inflammatory and chemopreventive therapeutic potential and does not induce toxicogenetic damage.
Declaration of interest
The authors report that they have no conflicts of interest. The authors alone are responsible for the content and writing of the paper. This research was funded by FUNDECT (Edital Chamada FUNDECT #5/2011 – PPP – Processo #23/200.702/2012 – Termo de Outorga #0207/12 and Edital Chamada FUNDECT No. 14/2009 – Universal – Processo 23/200.151/2010 – Termo de Outorga 0059/11). We also would like to thank CNPq for Rocha R. S. scholarship.
References
- Antunes LMG, Araújo MCP. (2000). Mutagenicidade e antimutagenicidade dos principais corantes para alimentos. Rev Nutr 13:81–8
- Barreto RC, Pereira GAS, Da Costa LJ, Cavalcanti HRBB. (2011). The double role of inflammation in the emergence of cancerous lesions. Rev Bras Cienc Saúde 14:107–14
- Chaplan SR, Bach FW, Pogrel JW, et al. (1994). Quantitative assessment of tactile allodynia in the rat paw. J Neurosci Methods 53:55–63
- Chavan MJ, Kolhe DR, Wakte PS, Shinde DB. (2012a). Analgesic and anti-inflammatory activity of kaur-16-en-19-oic acid from Annona reticulata L. bark. Phytother Res 26:273–6
- Chavan MJ, Wakte PS, Shinde DB. (2011). Analgesic and anti-inflammatory activities of 18-acetoxy-ent-kaur-16-ene from Annona squamosa L. bark. Inflammopharmacology 19:111–15
- Chavan MJ, Wakte PS, Shinde DB. (2010). Analgesic and anti-inflammatory activity of caryophyllene oxide from Annona squamosa L. bark. Phytomedicine 17:149–51
- Chavan MJ, Wakte PS, Shinde DB. (2012b). Analgesic and anti-inflammatory activities of the sesquiterpene fraction from Annona reticulata L. bark. Nat Prod Res 26:1515–18
- Cochrane CB, Nair PK, Melnick SJ, et al. (2008). Anticancer effects of Annona glabra plant extracts in human leukemia cell lines. Anticancer Res 28:965–71
- Conte FP, Barja-Fidalgo C, Verri WA Jr, et al. (2008). Endothelins modulate inflammatory reaction in zymosan-induced arthritis: Participation of LTB4, TNF-alpha, and CXCL-1. J Leukoc Biol 84:652–60
- Corrêa MP. (1926). Dicionário das plantas úteis do Brasil e das exóticas cultivadas. Rio de Janeiro: Imprensa Nacional
- Cruz GL. (1979). Dicionário das plantas úteis do Brasil. Rio de Janeiro: Civilização Brasileira
- De Flora S. (1998). Mechanisms of inhibitors of mutagenesis and carcinogenesis. Mutation Res 402:151–8
- De Flora S, Ferguson LR. (2005). Overview of mechanisms of cancer chemopreventive agents. Mutation Res 591:8–15
- De Omena MC, Navarro DM, De Paula JE, et al. (2007). Larvicidal activities against Aedes aegypti of some Brazilian medicinal plants. Bioresour Technol 98:2549–56
- De Sousa OV, Vieira GD, De Jesus RG, et al. (2010). Antinociceptive and anti-inflammatory activities of the ethanol extract of Annona muricata L. leaves in animal models. Int J Mol Sci 11:2067–78
- Dos Santos AF, Sant'Ana AE. (2001). Molluscicidal properties of some species of Annona. Phytomedicine 8:115–20
- Dragano NRV, Venancio VP, Paula FBA, et al. (2010). Influence of marolo (Annona crassiflora Mart.) pulp intake on the modulation of mutagenic/antimutagenic processes and its action on oxidative stress in vivo. Plant Foods Hum Nutr 65:319–25
- Fedel-Miyasato LES, Formagio ASN, et al. (2014). Antigenotoxic and antimutagenic effects of Schinus terebinthifolius Raddi in Allium cepa and Swiss mice: A comparative study. Genet Mol Res 13:3411–25
- Felicidade I, Lima JD, Pesarini JR, et al. (2014). Mutagenic and antimutagenic effects of crude hydroalcoholic extract of rosemary (Rosmarinus officinalis L.) on cultured meristematic cells Allium cepa. VRI Phytomed 2:30–9
- Ferguson LR. (1994). Antimutagens as cancer chemopreventive agents in the diet. Mutation Res 307:395–410
- Fiskesjö G. (1993). The Allium cepa test in wastewater monitoring. Environ Toxicol Water Qual 8:291–8
- Flagg EW, Coates RJ, Greenberg RS. (1995). Epidemiologic studies of antioxidants and cancer in humans. J Am Coll Nutr 14:419–27
- Formagio AS, Vieira Mdo C, Dos Santos LA, et al. (2013a). Composition and evaluation of the anti-inflammatory and anticancer activities of the essential oil from Annona sylvatica A. St.-Hil. J Med Food 16:20–5
- Formagio ASN, Kassuya CAL, Neto FF, et al. (2013b). The flavonoid content and antiproliferative, hypoglycaemic, anti-inflammatory and free radical scavenging activities of Annona dioica St. Hill. BMC Complement Altern Med 11:13–14
- Garcia-Ramallo E, Marques T, Prats N, et al. (2002). Resident cell chemokine expression serves as the major mechanism for leukocyte recruitment during local inflammation. J Immunol 169:6467–73
- Graham JG, Quinn ML, Fabricant DS, Farnsworth NR. (2000). Plants used against cancer – An extension of the work of Jonathan Hartwell. J Ethnopharmacol 73:347–77
- Hartman PE, Shankel DM. (1990). Antimutagens and anticarcinogens: A survey of putative interceptor molecules. Environ Mol Mutagen 15:145–82
- Hayashi M, Morita T, Kodama Y, et al. (1990). The micronucleus assay with mouse peripheral blood reticulocytes using acridine orange coated slides. Mutat Res 245:245–9
- Henriques MG, Weg VB, Martins MA, et al. (1990). Differential inhibition by two hetrazepine PAF antagonists of acute inflammation in the mouse. Br J Pharmacol 99:164–8
- Kada T, Shimoi K. (1987). Desmutagens and bio-antimutagens: Their modes of action. Bio Essay 7:113–15
- Kantarci OH, Goris A, Hebrink DD, et al. (2005). IFNG polymorphisms are associated with gender differences in susceptibility to multiple sclerosis. Genes Immun 6:153–61
- Kassuya CA, Cremoneze A, Barros LF, et al. (2009). Antipyretic and anti-inflammatory properties of the ethanolic extract, dichloromethane fraction and costunolide from Magnolia ovata (Magnoliaceae). J Ethnopharmacol 124:369–76
- Kassuya CA, Ferreira J, Claudino RF, Calixto JB. (2007). Intraplantar PGE2 causes nociceptive behaviour and mechanical allodynia: The role of prostanoid E receptors and protein kinases. Br J Pharmacol 150:727–37
- Kumar V, Abbas AK, Fausto N. (2006). Robbins & Cotran, Fundamentos de Patologia. 7th ed. Rio de Janeiro: Elsevier
- Leme DM, Marin-Morales MA. (2009). Allium cepa test in environmental monitoring: A review on its application. Mutat Res 682:71–81
- Lin JY, Tang CY. (2007). Determination of total phenolic and flavonoid contents in selected fruits and vegetables, as well as their stimulatory effects on mouse splenocyte proliferation. Food Chem 101:140–7
- Manoharan K, Banerjee MR. (1985). Beta-carotene reduces sister chromatid exchanges induced by chemical carcinogens in mouse mammary cells in organ culture. Cell Biol Int Rep 9:783–9
- Mauro MO, Monreal MTFD, Silva MTP, et al. (2013). Evaluation of the antimutagenic and anticarcinogenic effects of inulin in vivo. Genet Mol Res 12:2281–93
- Mauro MO, Pesarini JR, Marin-Morales MA, et al. (2014). Evaluation of the antimutagenic activity and mode of action of the fructooligosaccharide inulin in the meristematic cells of Allium cepa culture. Genet Mol Res 13:4808–19
- Medzhitov R. (2010). Inflammation: New adventures of an old flame. Cell 140:771–6
- Menezes-de-Lima O Jr, Kassuya CA, Nascimento AF, et al. (2006). Lipoxin A4 inhibits acute edema in mice: Implications for the anti-edematogenic mechanism induced by aspirin. Prostaglandins Other Lipid Mediat 80:123–35
- Mesquita ML, Desrivot J, Bories C, et al. (2005). Antileishmanial and trypanocidal activity of Brazilian Cerrado plants. Mem Inst Oswaldo Cruz 100:783–7
- Niikawa M, Nakamura T, Nagase H. (2001). Suppressive effect of aspirin on chromosome aberration induced by mitomycin C in mice. Biol Pharm Bull 24:964–6
- Oliveira RJ, Pesarini JR, Salles MJS, et al. (2014). Effects of β-glucan polysaccharide revealed by the dominant lethal assay and micronucleus assays, and reproductive performance of male mice exposed to cyclophosphamide. Genet Mol Biol 37:111–19
- Oliveira RJ, Baise E, Mauro MO, et al. (2009a). Evaluation of chemopreventive activity of glutamine by the comet and the micronucleus assay in mice's peripheral blood. Environ Toxicol Pharmacol 28:120–4
- Oliveira RJ, Matuo R, Silva AF, et al. (2007). Protective effect of β-glucan extracted from Saccharomyces cerevisiae, against DNA damage and cytotoxicity in wild-type (k1) and repair-deWcient (xrs5) CHO cells. Toxicol In Vitro 21:41–52
- Oliveira RJ, Salles MJS, Silva AF, et al. (2013a). In vivo evaluation of the antimutagenic and antigenotoxic effects of β-glucan extracted from Saccharomyces cerevisiae in acute treatment with multiple doses. Genet Mol Biol 36:413–24
- Oliveira RJ, Salles MJS, Silva AF, et al. (2009b). Effects of the polysaccharide β-glucan on clastogenicity and teratogenicity caused by acute exposure to cyclophosphamide in mice. Regul Toxicol Pharmacol 53:164–73
- Oliveira RJ, Sassaki ES, Monreal ACD, et al. (2013b). Pre-treatment with glutamine reduces genetic damage due to cancer treatment with cisplatin. Genet Mol Res 12:6040–51
- Oliveira RJ, Ribeiro LR, Silva AF, et al. (2006). Evaluation of antimutagenic activity and mechanisms of action of β-glucan from barley, in CHO-k1 and HTC cell lines using the micronucleus test. Toxicol In Vitro 20:1225–33
- Penido C, Conte FP, Chagas MS, et al. (2006). Antiinflammatory effects of natural tetranortriterpenoids isolated from Carapa guianensis Aublet on zymosan-induced arthritis in mice. Inflamm Res 55:457–64
- Pesarini JR, Zaninetti PT, Mauro MO, et al. (2013). Antimutagenic and anticarcinogenic effects of wheat bran in vivo. Genet Mol Res 12:1646–59
- Rank J, Nielsen MH. (1997). Aliium cepa anaphase-telophase root tip chromosome aberration assay on N-methyl-N-nitrosourea, maleic hydrazide, sodium azide and ethyl methanesulfonate. Mutat Res 390:121–7
- Rodrigues AM, De Paula JE, Degallier N, et al. (2006). Larvicidal activity of some Cerrado plant extracts against Aedes aegypti. J Am Mosq Control Assoc 22:314–17
- Roesler R, Lorencini M, Pastore G. (2010). Brazilian cerrado antioxidant sources: Cytotoxicity and phototoxicity in vitro. Ciênc Tecnol Aliment 30:814–21
- Simic D, Vukovic-Gacic B, Knecevic-Vukcevic J. (1998). Detection of natural bioantimutagens and their mechanisms of action with bacterial assay-system. Mutat Res 402:52–7
- Souza VR, Pereira PAP, Queiroz F, et al. (2012). Determination of bioactive compounds, antioxidant activity and chemical composition of Cerrado Brazilian fruits. Food Chem 134:381–6
- Suleiman MM, Dzenda T, Sani CA. (2008). Antidiarrheal activity of the methanol stem-bark extract of Annona senegalensis Pers. (Annonaceae). J Ethnopharmacol 28:125–30
- Takahashi JA, Pereira CR, Pimenta LP, et al. (2006). Antibacterial activity of eight Brazilian Annonaceae plants. Nat Prod Res 20:21–6
- Vilar JB, Ferreira FL, Ferri PH, et al. (2008). Assessment of the mutagenic, antimutagenic and cytotoxic activities of ethanolic extract of araticum (Annona crassiflora Mart. 1841) by micronucleus test in mice. Braz J Biol 68:141–7
- Vilar JB, Ferri PH, Chen Chen L. (2011). Genotoxicity investigation of araticum (Annona crassiflora Mart., 1841, Annonaceae) using SOS-Induce test and Ames test. Braz J Biol 71:197–202
- Waters MD, Brady AL, Stack HF, Brockman HE. (1990). Antimutagenicity profiles for some model compounds. Mutat Res 238:57–85
- Weinberg Mde L, Pires V, Weinberg J, De Oliveira AB. (1993). Inhibition of drug-induced contractions of guinea-pig ileum by Annona crassiflora seed extract. J Pharm Pharmacol 45:70–2
- Yeo D, Dinica R, Yapi HF, et al. (2011). Evaluation of the anti-inflammatory activity and phytochemical screening of Annona senegalensis leaves. Therapie 66:73–80