Abstract
Context: Doxorubicin (DOX)-loaded folate-targeted poly(3-hydroxybutyrate-co-3-hydroxyoctanoate) [P(HB-HO)] nanoparticles [DOX/FA-PEG-P(HB-HO) NPs] were prepared by the W1/O/W2 solvent extraction/evaporation method for applications in cancer treatment. However, the biodistribution, pharmacokinetics, and targeting of the nanoparticles (NPs) have not yet been studied.
Objective: The biodistribution, pharmacokinetics, and targeting of DOX/FA-PEG-P(HB-HO) NPs were evaluated in female BALB/c nude mice bearing HeLa tumors.
Materials and methods: Three DOX formulations were injected into the tail vein of the mice at a dosage of 5 mg/kg. At each time point, blood and various tissues were collected. All samples were then processed and analyzed by a validated high performance liquid chromatographic (HPLC) method.
Results: The t1/2 values of DOX/P(HB-HO) NPs and DOX/FA-PEG-P(HB-HO) NPs were 2.7- and 3.5-times higher than that of free DOX. No significant difference (p > 0.05) was found in Cmax between the NPs and free DOX. The Tmax values of the two NPs were prolonged from 0.25 to 1 h. The AUC0–t values were 1.55- and 3.05-folds higher than that of free DOX, and MRT increased to 15.99 h for DOX/P(HB-HO) NPs and 25.14 h for DOX/FA-PEG-P(HB-HO) NPs. For DOX/FA-PEG-P(HB-HO) NPs, the DOX content in the tumors were 10.81- and 3.33-times higher than those for free DOX and DOX/P(HB-HO) NPs at 48 h, respectively.
Discussion and conclusions: DOX/FA-PEG-P(HB-HO) NPs displayed reduced cardiac toxicity and improved bioavailability. Moreover, the NPs exhibited a significant extent of DOX accumulation in the tumors, thus suggesting that folate-targeted NPs could effectively transport into HeLa tumors with satisfying targeting.
Introduction
Doxorubicin (DOX), an anthracycline glycoside antibiotic (Mukhopadhyay et al., Citation2009), is an effective chemotherapeutic agent (Chen et al., Citation2001) that is widely used in treating several human carcinomas, including cervical cancer, breast cancer, acute lymphoblastic leukemia, lymphoma, etc. (Zhang et al., Citation2007). The anticancer mechanism of DOX may be attributed to its interactions with DNA by the intercalation model, which inhibits the synthesis of nucleic acids (Hu et al., Citation2009). However, cardiac toxicity and myelosuppression are the main side effects of chemotherapy (Oh et al., Citation1999; Octavia et al., Citation2012). Altered free DOX formulations (such as microspheres, NPs, micelles, and liposomes) can reduce side effects, prolong retention time, and improve bioavailability. MyocetTM (Ekab Pharmaceuticals, Cyprus, Greece), which is a liposomal formulation, has a similar efficacy and improved toxicity profile compared with DOX (Harris et al., Citation2002). Tan et al. (Citation2005) fabricated DOX-loaded double-walled microspheres with sustained and controlled release properties for the drug. However, the large sizes (50–300 µm) of the microspheres hinder intravenous injection. Folate receptor-targeted poly(d,l-lactic-co-glycolic acid) (PLGA)-poly(ethylene glycol) (PEG) DOX micelles with superior cytotoxicity and targeting have been demonstrated by Yoo and Park (Citation2004).
Poly(3-hydroxybutyrate-co-3-hydroxyoctanoate) [P(HB-HO)] is one of the latest polyhydroxyalkanoates (PHAs) fermented by Sinorhizobium fredii Chen. (CCTCC no. AB 92049) belongs to the family of Rhizobiaceae (Zhao et al., Citation2006). And P(HB-HO) is used as a drug carrier because of its biodegradation (Guo et al., Citation2009) and biocompatibility (Dong et al., Citation2008). P(HB-HO) microspheres (Zhang et al., Citation2014) and folate-targeted NPs (Zhang et al., Citation2010) were prepared in our previous study.
The study aims to introduce a new sensitive and precise HPLC method for the quantification of DOX and to evaluate and compare the pharmacokinetics, biodistribution, and targeting of DOX-loaded folate-targeted P(HB-HO) NPs [DOX/FA-PEG-P(HB-HO) NPs], free DOX, and DOX-loaded P(HB-HO) NPs [DOX/P(HB-HO) NPs] in the plasma and tissues of female BALB/c nude mice bearing HeLa tumors.
Materials and methods
Chemicals and reagents
P(HB-HO) (Mw 1.85 × 105) containing 10% mol of 3-hydroxyoctanoate units was fermented by Sinorhizobium fredii. DOX (C27H29NO11.HCl, Mw 579.98) was purchased from Wanle Pharmaceuticals Inc. (Shenzhen, China). HPLC grade acetonitrile was acquired from DIMA Technology Inc. (Lake Forest, CA). Other chemicals were of analytical grade. Distilled water was produced by the Milli-Q Plus System (Millipore Corporation, Bedford, MA).
DOX/P(HB-HO) NPs (223.2 ± 5.1 nm) and DOX/FA-PEG-P(HB-HO) NPs (241.6 ± 9.3 nm) for cancer treatment applications were prepared by the W1/O/W2 solvent extraction/evaporation method, as described previously (Zhang et al., Citation2010). Briefly, P(HB-HO) [or FA-PEG-P(HB-HO)] was dissolved in chloroform containing emulsifiers. DOX in aqueous solution (W1) was added to the P(HB-HO) [or FA-PEG-P(HB-HO)] solution (O), and the mixture was sonicated using a sonicator. This primary emulsion (W1/O) was then added to an aqueous phase containing an emulsifier (W2), and the mixture was sonicated to form the W1/O/W2 emulsion. The resulting double emulsion was stirred and centrifuged. Finally, the sample was washed and freeze-dried to obtain the NPs powder.
Chromatographic conditions
The chromatographic system (Waters 1525 series, Westbrook, CT) primarily consisted of a Waters 1525 binary pump and a Waters 2487 dual λ absorbance detector set at 254 nm. The analytical column was a Thermo Hypersil Gold (250 mm × 4.6 mm, 5 µm, Waltham, MA). The mobile phase consisted of acetonitrile and distilled water (adjusted to pH 3.0 with phosphoric acid) with a volumetric ratio of 32/68. The flow rate was 1.0 mL·min−1, and the column temperature was 30 °C.
Experimental animals
All experiments were carried out in compliance with the ethical standards required by law and according to the guidelines for the use of experimental animals in China. The protocol approval number of the certification for using and caring the experimental animals is SCXK (jing) 2009-0004.
Female BALB/c nude mice (4–6 weeks of age) were purchased from Chinese Academy of Medical Sciences (Beijing, China) and were maintained under a pathogen-free condition. These mice were subcutaneously inoculated with HeLa cells (a human cervical carcinoma cell line provided by Cell Bank of the Chinese Academy of Sciences) in the right limb armpits. HeLa cells with overexpressed folate receptors on the cell surfaces were incubated in RPMI 1640 medium supplemented with 10% FBS and 1% antibiotics (penicillin–streptomycin solution) at 37 °C, 5% CO2. The cells were briefly trypsinized and washed three times with PBS, resuspended in normal saline at 1 × 107 cells/20 µL, and then injected into the armpits. Mice were administered with DOX formulations (5 mg/kg) 10 d following injection with HeLa cells and had a median tumor volume of 50 mm3.
Pharmacokinetics, biodistribution, and tumor targeting of nanoparticles in vivo
The pharmacokinetics, biodistribution, and tumor targeting of DOX/FA-PEG-P(HB-HO) NPs were studied in HeLa tumor-bearing mice. On the 10th day after inoculation of tumor cells, 54 mice were randomly divided into three treated groups. Various formulations of DOX (group I: DOX/P(HB-HO) NPs; group II: DOX/FA-PEG-P(HB-HO) NPs; group III: free DOX) were injected into the tail veins of the tumor-bearing mice at a dosage of 5 mg/kg. Blood was collected from three mice and centrifuged at 3000 rpm for 10 min to separate the plasma at selected times (t = 0.25, 1, 4, 12, 24, and 48 h). The animals were then sacrificed, and the tissues (heart, liver, spleen, lungs, kidneys, and tumor) were collected, weighted, blotted with filter paper towel to remove the residual blood, and homogenized in 1 mL of PBS (pH 7.4). All samples were stored at −20 °C until analysis. Plasma and tissues from non-dosed mice (n = 9) were also collected for the preparation of standard curves.
Sample preparation
DOX extraction from plasma (0.5 mL) and tissues (0.5 mL homogenate) was performed by adding 3 mL of chloroform–methanol (4:1, v/v) mixture with continuous stirring for 2 min. After centrifugation (3000 rpm, 10 min), the organic phases were collected and evaporated to dryness at 30 °C under a flow of nitrogen. The dry residues were dissolved in 200 µL of methanol and then centrifuged. 30 µL of the sample was injected into the HPLC column for analysis.
Foundation of DOX content determination
Aqueous stock solutions of DOX were prepared and stored at 4 °C. The working solutions of DOX were prepared by appropriate dilutions of the stock solutions at various concentrations. A total of 50 µL of each working solution was added to each normal untreated mice plasma (0.5 mL) or tissue homogenate (0.5 mL). The final concentrations of the calibration samples used to draw the standard-curves in plasma and tissues ranged from 2.22 to 40 µg/mL. The samples were then processed as described in the “Sample preparation” section. The peak areas of DOX (A) and the concentrations of DOX (C) were utilized to plot the calibration curves.
To assess the intra- and inter-day precision of the assay, blank plasma or tissue homogenate samples with three DOX solutions at low, medium, and high concentrations (3.03, 18.18, and 36.36 µg/mL or 2.22, 13.33, and 26.67 µg/mL) were prepared according to the steps described in the Section of “Sample preparation”. The intra-day precision was determined by analyzing each concentration five times. The inter-day precision was determined by analyzing the samples for five consecutive days. The precision of the assay was assessed by calculating the relative standard deviation (RSD) for each concentration level.
DOX solutions with different concentrations were added into the blank plasma or tissue homogenate samples. The samples were then processed as mentioned above and were analyzed five times for each concentration using HPLC. The recovery was calculated as follows:
Results
Foundation of DOX content determination
The typical HPLC chromatograms of the DOX samples are shown in . Under the above chromatographic conditions, DOX was eluted at 4.5 min during the 10 min run-time, and no interfering peaks of endogenous components in the plasma and tissues were observed at the retention time (). The linearity of the method was statistically confirmed and was validated for DOX in plasma and various tissues over the concentration ranges of 3.03–40 and 2.22–30 µg/mL, respectively. The standard curves generated over these concentration ranges were linear with correlation coefficients (R) greater than 0.9970 (). As previously defined, the limit of detection (LOD) was approximately 50 ng/mL.
Figure 1. HPLC chromatograms of DOX in different samples. (A) DOX solution; (B) DOX in plasma sample; (C) DOX in heart sample; (D) DOX in liver sample; (E) DOX in spleen sample; (F) DOX in lung sample; (G) DOX in kidney sample; (H) DOX in tumor sample.
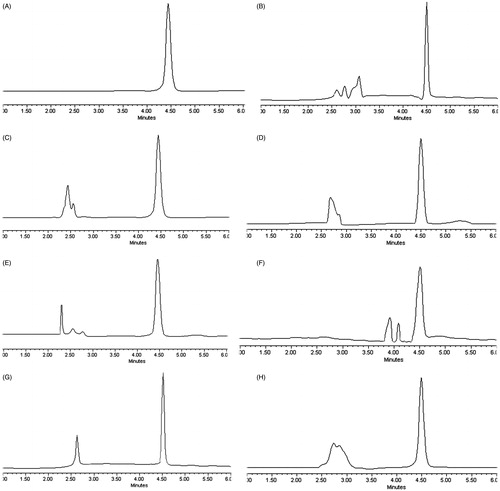
Table 1. Calibration curve of DOX in plasma and different tissues.
The intra- and the inter-day precision of the method were evaluated using three DOX solutions at low, medium, and high concentrations (3.03, 18.18, and 36.36 µg/mL for plasma or 2.22, 13.33, and 26.67 µg/mL for tissues), and the results are listed in . The intra- and inter-day RSD values ranged from 0.16 to 6.88% and 0.2 to 7.17%, respectively. These data suggested that the method had excellent accuracy and precision.
Table 2. Accuracy and precision of the method for determination of DOX contents.
The results of the method recovery are shown in . The average recoveries of DOX in the plasma, heart, liver, spleen, lungs, kidneys and tumor were 99.15 ± 3.26%, 99.43 ± 0.75%, 99.01 ± 1.67%, 96.42 ± 2.87%, 99.85 ± 4.32%, 99.31 ± 2.21%, and 97.81 ± 2.17%, respectively. There was no significant difference (p > 0.05) in the recovery methods for determination of DOX contents in different biological samples. The average recoveries of DOX in the plasma and various tissues were more than 96%, and the RSD ranged from 0.75 to 4.33%.
Table 3. Recovery of the method for determination of DOX contents.
Pharmacokinetics, biodistribution, and tumor targeting of nanoparticles in vivo
In this paper, the plasma and tissue levels of the DOX formulations were determined following a single injection of DOX/P(HB-HO) NPs, DOX/FA-PEG-P(HB-HO) NPs or free DOX (5 mg/kg) in female BALB/c nude mice bearing HeLa tumors. The plasma drug concentration–time curves (mean ± SD) of DOX are presented in , and the corresponding pharmacokinetic parameters are listed in . The t1/2 values of DOX/P(HB-HO) NPs and DOX/FA-PEG-P(HB-HO) NPs were 2.7- and 3.5-times higher than that of free DOX. No significant difference (p > 0.05) was found in the Cmax between the NPs and free DOX. The Tmax values of the two NPs were prolonged from 0.25 to 1 h, whereas the AUC0–t values of the two NPs were 1.55- and 3.05-folds higher than that of free DOX. Moreover, MRT increased to 15.99 and 25.14 h for DOX/P(HB-HO) NPs and DOX/FA-PEG-P(HB-HO) NPs, respectively. From the above results, DOX/P(HB-HO) NPs and DOX/FA-PEG-P(HB-HO) NPs displayed improved pharmacokinetic profiles compared with free DOX in vivo. The distribution of different DOX formulations in HeLa tumor-bearing mice () was determined at the three time points of 4, 24, and 48 h. In the experiment, the DOX levels in the plasma and tissues gradually decreased at different rates. The DOX contents in the tumor, liver, and kidneys decreased more slowly than that in the plasma and other tissues. A significantly lower DOX contents (p < 0.05) in the heart was detected for the NPs groups (0.85 ± 0.37 or 0.52 ± 0.11 µg/mL) than for the free DOX group (1.86 ± 0.87 µg/mL) at 24 h (). The level of the free DOX in the tumors peaked at 1.74 ± 0.89 µg/mL at 4 h and dramatically decreased to 0.32 ± 0.09 µg/mL at 48 h (). For DOX/FA-PEG-P(HB-HO) NPs in the tumors, the DOX contents maximally increased at 24 h (5.39 ± 0.39 µg/mL) and then slowly decreased to 3.46 ± 0.19 µg/mL at 48 h (). However, for DOX/P(HB-HO) NPs in the tumor, the DOX level reached a peak level of 3.58 ± 0.98 µg/mL at 24 h and gradually decreased to 1.04 ± 0.38 µg/mL at 48 h (). The DOX concentrations in the tumors for the DOX/FA-PEG-P(HB-HO) NPs group were 10.81- and 3.33-times higher than those of the free DOX and DOX/P(HB-HO) NPs groups at 48 h, respectively.
Figure 2. Mean DOX concentration–time curves in plasma after intravenous administration of free DOX, DOX/P(HB-HO) NPs and DOX/FA-PEG-P(HB-HO) NPs to tumor-bearing mice (n = 3) ((A) 0–48 h; (B) 0–12 h).
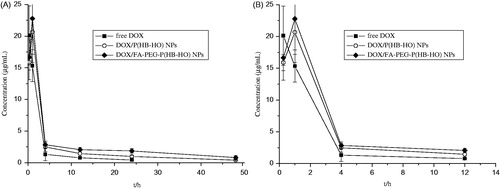
Figure 3. Biodistribution of free DOX, DOX/P(HB-HO) NPs and DOX/FA-PEG-P(HB-HO) NPs in blood, heart, liver, spleen, lung, kidney, and tumor at different times ((A) 4 h, (B) 24 h, (C) 48 h) after intravenous administration to tumor-bearing mice (n = 3). *p < 0.05 compared with free DOX; **p < 0.01 compared with free DOX.
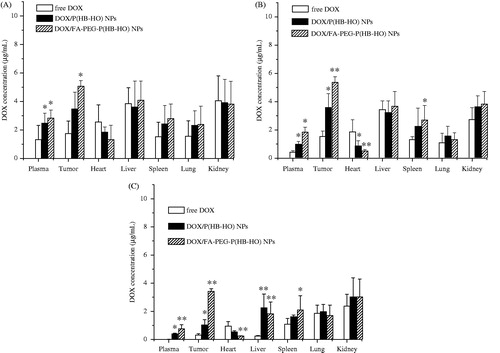
Table 4. Main pharmacokinetic parameters of DOX formulations after i.v. administration.
Discussion
To date, many analysis methods for DOX, such as fluorescence assay (Bibby et al., Citation2005; Wang et al., Citation2006), radiolabelled assay (Ogawara et al., Citation2008), LC/MS assay (Arnold et al., Citation2004; DiFrancesco et al., Citation2007), and other methods (Hu et al., Citation2007; Kim & Wainer, Citation2010), have been reported. However, several problems may be encountered in these assay methods, such as comparatively difficult procedures, complex compositions of the mobile phases, or high costs in equipment. Favorable chromatographic conditions should include the following: baseline separation of the analyte from other exogenous materials, a mobile phase with a simple composition and an appropriate pH value, brief procedure, and relatively good accuracy and precision.
In this paper, the HPLC analysis of different DOX formulations in biological samples was developed and exploited. In the experiment, a simple mobile phase consisting of acetonitrile and distilled water (adjusted to pH 3.0 with phosphoric acid) was used. The chromatographic conditions and procedures were easily controlled. According to the HPLC conditions adopted, DOX was eluted at 4.5 min within 10 min without any interfering exogenous materials in the plasma and tissues. The values of the linearity and specificity demonstrated that the peak areas and DOX concentrations displayed perfect linear relationships and that the assay had adequate specificity. In this study, the accuracy and precision values met the requirements for the determination of DOX contents in biological samples. The favorable results showed that the simple method developed for plasma and tissue handling exhibited relatively high accuracy and recovery, and was suitable for extracting and measuring the DOX contents.
The applicability of the assay method was demonstrated in this study for the pharmacokinetics, biodistribution, and targeting of free DOX, DOX/P(HB-HO) NPs and DOX/FA-PEG-P(HB-HO) NPs in female BALB/c nude mice bearing HeLa tumors. The three DOX formulations reached the major tissues after intravenous administration. The DOX levels in the plasma, heart, spleen, and lungs rapidly decreased compared with those in the tumors, liver, and kidneys. This phenomenon might be related to the half-life, targeting, and sustained release effects. Compared with free DOX, the circulation and action time of the two NPs in vivo was prolonged, and their bioavailability also improved (Kalaria et al., Citation2009). This result might be attributed to the encapsulation of the drug into NPs, protecting them from rapid elimination after administration. This protection thereby allows the drug to be transported through blood vessel walls into other tissues. The DOX contents in the heart for the NPs was lower than that for free DOX, thus indicating that the cardiac toxicity of DOX might be substantially reduced by the formulation of NPs. Similar toxicities for DOX/P(HB-HO) NPs and DOX/FA-PEG-P(HB-HO) NPs were observed compared with those shown in previous studies. It was very obvious that the distribution of DOX in the heart for liposomal DOX preparations was significantly reduced at 0.5 h compared with the free DOX preparations (Wang et al., Citation2006). Furthermore, significantly lower DOX contents for the DOX micelles and DOX/FOL micelles were detected in the heart than for free DOX at 24 h (Yoo & Park, Citation2004). This consequence was encouraging because cardiac toxicity is a unique characteristic of DOX chemotherapies.
The DOX contents in the tumors reached a peak level at 4 h and dramatically decreased at 48 h for free DOX. In contrast, when DOX/P(HB-HO) NPs or DOX/FA-PEG-P(HB-HO) NPs were administered, the DOX contents remained at a high level up to 48 h. NPs tend to accumulate in the solid tumors through the enhanced permeability and retention effect (EPR) (Acharya & Sahoo, Citation2011), thus resulting reduced DOX concentrations in the blood and heart. These results are in good agreement with those of previous studies on cardiac toxicity. The DOX contents in the tumors for the DOX/FA-PEG-P(HB-HO) NP group were much higher than that for the free DOX and DOX/P(HB-HO) NP groups at 48 h. The folate-targeted NPs exhibited a greater extent of DOX accumulation in the tumors than DOX/P(HB-HO) NPs, thus suggesting that DOX/FA-PEG-P(HB-HO) NPs were more effectively transported and actively targeted into HeLa tumors than DOX/P(HB-HO) NPs because of the receptor-mediated endocytosis (Cho et al., Citation2008).
Conclusion
A sensitive, precise, and reproducible HPLC method was developed and adopted to quantify DOX in biological specimens, including plasma, heart, liver, spleen, lungs, kidneys, and tumor. This assay utilized a brief and convenient external standard method and only involved the rapid simple pre-treatment of liquid–liquid extraction for samples. Moreover, the method displayed sufficient sensitivity and precision in determining the DOX contents in biological samples. The HPLC method developed in the present study has been successfully applied in evaluating the pharmacokinetics, biodistribution, and targeting of DOX/FA-PEG-P(HB-HO) NPs in female BALB/c nude mice bearing HeLa tumors. In vivo studies confirmed that the circulation and bioavailability of the NPs were improved, and that the folate-targeted NPs were more effectively transported and actively targeted into HeLa tumors than non-targeted NPs because of the receptor-mediated endocytosis.
Declaration of interest
The authors report that they have no conflicts of interest. This work was supported by the Doctorate Science Funds of Taiyuan University of Science and Technology [20122007]; and the Natural Science Foundation for Young Scientists of Shanxi Province, China [2013021011-7].
References
- Acharya S, Sahoo SK. (2011). PLGA nanoparticles containing various anticancer agents and tumour delivery by EPR effect. Adv Drug Deliv Rev 63:170–83
- Arnold RD, Slack JE, Straubinger RM. (2004). Quantification of doxorubicin and metabolites in rat plasma and small volume tissue samples by liquid chromatography/electrospray tandem mass spectroscopy. J Chromatogr B 808:141–52
- Bibby DC, Talmadge JE, Dalal MK, et al. (2005). Pharmacokinetics and biodistribution of RGD-targeted doxorubicin-loaded nanoparticles in tumor-bearing mice. Int J Pharm 293:281–90
- Chen CL, Thoen KK, Uckun FM. (2001). High-performance liquid chromatographic methods for the determination of topoisomerase II inhibitors. J Chromatogr B 764:81–119
- Cho K, Wang X, Nie SM, et al. (2008). Therapeutic nanoparticles for drug delivery in cancer. Clin Cancer Res 14:1310–16
- DiFrancesco R, Griggs JJ, Donnelly J, et al. (2007). Simultaneous analysis of cyclophosphamide, doxorubicin and doxorubicinol by liquid chromatography coupled to tandem mass spectrometry. J Chromatogr B 852:545–53
- Dong YF, Xie H, Guo Y, et al. (2008). Biocompatibility of poly(3-hydroxybutyrate-co-hydroxyocatanoate). J Clin Reh Tissue Eng Res 12:1870–2
- Guo Y, Dong YF, Chen Z, et al. (2009). Study of P(HB-HO) as scaffold for bone tissue engineering. J Funct Mater 3:459–66
- Harris L, Batist G, Belt R, et al. (2002). Liposome-encapsulated doxorubicin compared with conventional doxorubicin in a randomized multicenter trial as first-line therapy of metastatic breast carcinoma. Cancer 94:25–36
- Hu FQ, Liu LN, Du YZ, et al. (2009). Synthesis and antitumor activity of doxorubicin conjugated stearic acid-g-chitosan oligosaccharide polymeric micelles. Biomaterials 30:6955–63
- Hu T, Le QH, Wu ZY, et al. (2007). Determination of doxorubicin in rabbit ocular tissues and pharmacokinetics after intravitreal injection of a single dose of doxorubicin-loaded poly-β-hydroxybutyrate microspheres. J Pharm Biomed 43:263–9
- Kalaria DR, Sharma G, Beniwal V, et al. (2009). Design of biodegradable nanoparticles for oral delivery of doxorubicin: In vivo pharmacokinetics and toxicity studies in rats. Pharm Res 26:492–501
- Kim HS, Wainer IW. (2010). Simultaneous analysis of liposomal doxorubicin and doxorubicin using capillary electrophoresis and laser induced fluorescence. J Pharm Biomed 52:372–6
- Mukhopadhyay P, Rajesh M, Bátkai S, et al. (2009). Role of superoxide, nitric oxide, and neroxynitrite in doxorubicin-induced cell death in vivo and in vitro. Am J Physiol-Heart C 296:H1466–83
- Octavia Y, Tocchetti CG, Gabrielson KL, et al. (2012). Doxorubicin-induced cardiomyopathy: From molecular mechanisms to therapeutic strategies. J Mol Cell Cardiol 52:1213–25
- Ogawara K, Un K, Minato K, et al. (2008). Determinants for in vivo anti-tumor effects of PEG liposomal doxorubicin: Importance of vascular permeability within tumors. Int J Pharm 359:234–40
- Oh I, Lee K, Kwon HY, et al. (1999). Release of adriamycin from poly(γ-benzyl-L-glutamate)/poly(ethylene oxide) nanoparticles. Int J Pharm 181:107–15
- Tan EC, Lin R, Wang CH. (2005). Fabrication of double-walled microspheres for the sustained release of doxorubicin. J Colloid Interface Sci 291:135–43
- Wang JC, Liu XY, Lu WL, et al. (2006). Pharmacokinetics of intravenously administered stealth liposomal doxorubicin modulated with verapamil in rats. Eur J Pharm Biopharm 62:44–51
- Yoo HS, Park TG. (2004). Folate receptor targeted biodegradable polymeric doxorubicin micelles. J Control Release 96:273–83
- Zhang C, Dong YF, Zhao LQ. (2014). Preparation and characterization of novel microparticles based on poly (3-hydroxybutyarte-co-3-hydroxyoctanoate). J Microencapsul 31:9–15
- Zhang C, Zhao LQ, Dong YF, et al. (2010). Folate-mediated poly(3-hydroxybutyrate-co-3-hydroxyoctanoate) nanoparticles for targeting drug delivery. Eur J Pharm Biopharm 76:10–16
- Zhang ZP, Lee SH, Feng SS. (2007). Folate-decorated poly(lactide-co-glycolide)-vitamin E TPGS nanoparticles for targeted drug delivery. Biomaterials 28:1889–99
- Zhao LQ, Xiao JF, Feng T, et al. (2006). Synthesis of poly (3-hydroxybutyrate-co-3-hydroxyoctanoate) by a Sinorhizobium fredii strain. Lett Appl Microbiol 42:344–9