Abstract
Context: Capsaicin (CAP) is an effective drug in the treatment of pain and cancer. However, its practical administration has been limited due to poor aqueous solubility and bioavailability, as well as strong gastrointestinal irritation.
Objective: The objective of this study is to improve the solubility and oral bioavailability of CAP by reducing irritation via hydroxypropyl-β-cyclodextrin (HP-β-CD) inclusion complex formulation, in vitro and in vivo analysis.
Materials and methods: The complex (CAP-HP-β-CD) was developed via the magnetic stirring method and characterized using ultraviolet (UV) absorption spectrometry, infrared radiation (IR) spectroscopy, and differential scanning calorimetry (DSC). Rats were treated with CAP (90 mg × kg−1) or CAP-HP-β-CD (corresponding to 90 mg × kg−1 CAP) by gavage, and all the plasma samples were analyzed with high performance liquid chromatography (HPLC).
Results: The results of UV, IR, and DSC showed that an acceptable CAP-HP-β-CD (encapsulation efficiency, 75.83%; drug loading, 7.44%) was formulated. In vitro release study of CAP-HP-β-CD revealed that the cumulative release of CAP from HP-β-CD encapsulation was obviously enhanced (above 80% increases). Similarly, the in vivo pharmacokinetics parameters also increased, Cmax (from 737.94 to 1117.57 ng × mL−1), AUC0– (from 5285.9 to 7409.8 ng × h × mL−1) or relative bioavailability (139.38%). The gastric irritation bioassay further showed that the CAP-HP-β-CD was far better than free CAP.
Discussion and conclusion: CAP exhibited significant aqueous solubility and oral bioavailability, as well as minimal irritation effect after forming inclusion complex with HP-β-CD. Therefore, these findings could provide an equally important alternative option for the clinical use of CAP.
Introduction
Capsaicin (trans-8-methy-N-vanilly-6-nonenamide, CAP, ) is the main pungent ingredient isolated from Chili pepper (Caterina et al., Citation1997). Regarding its physicochemical properties, CAP is a kind of off-white microcrystal with strong pungent taste and irritation. It can freely dissolve in organic solvents like alcohol, diethyl ether, phenol, acetone, dioxane, methylbenzene, and aqueous alkali, but to a lesser extent in water at room temperature. CAP is associated with various complicated pharmacological actions such as the prevention and treatment of cardiovascular system diseases (Bencsik et al., Citation2008), anti-obesity (Joo et al., Citation2010), and protection of gastrointestinal tract (Szallasi & Blumberg, Citation1999), as well as antitumor actions (Lu et al., Citation2010) and analgesia (Menendez et al., Citation2004). However, lower water solubility and significant first pass effect of liver lead to poor in vivo bioavailability of CAP. The strong irritation further limits its practical applications. Therefore, there is a pressing need to develop CAP using modern technology to enhance its aqueous solubility and bioavailability, with concurrent reduction of its gastrointestinal irritation.
Currently, cyclodextrins (CD), especially the β-CD, has been widely used as an excipient to improve the aqueous solubility of undissolved drug (Fraceto et al., Citation2014; Strickley, Citation2004). But there are still some awaiting properties of β-CD that need to be improved, such as low aqueous solubility (18.5 g L−1 at 25 °C), difficult in vivo metabolism, and nephrotoxicity (Del Valle, Citation2004) which is the main reason for its limited practical application. Hydroxypropyl-β-cyclodextrin (HP-β-CD), amorphism powder, a derivative of β-CD has several advantages including excellent absorption and bioavailability (Shulman et al., Citation2011), strong solubilizing power (Ghosh et al., Citation2011; Yuvaraja & Khanam, Citation2014), and stability (Miyake et al., Citation2000), less toxicity (de Araujo et al., Citation2008) and irritation of tissues (Vega et al., Citation2012), and well tolerated in animals (de Paula et al., Citation2011). Most importantly, it is the first β-CD derivative to get approval from United States Food and Drug Administration (USFDA) as an intravenous administration. Furthermore, it has been certified as both oral and intravenous excipient in Europe, Japan, and United States.
Inclusion technology is a kind of specific molecular coating structure or clathrate, in the form of guest molecules (CAP) enveloped with holey structure of host molecules (HP-β-CD). Therefore, the prepared clathrate is non-chemical bond type complex, which is also called inclusion complex (Zhang & Ma, Citation2013). This technology can change the properties, thus physical, chemical, and even biological, of the guest molecules. Its drug applications are mainly reflected in the promotion of improved solubility, stability, bioavailability, and absorption in vivo, then simultaneously decreasing the irritation and toxicity of gastrointestinal tract and other tissues. Furthermore, masking any objectionable odor and the solidification of liquid drugs (Sharma & Sharma, Citation2010; Wang & Han, Citation2013; Zhang & Ma, Citation2013).
To the best of our knowledge, the oral administration of CAP-HP-β-CD in animal model has not been reported by other studies. Therefore, the inclusion complex of CAP-HP-β-CD was prepared and evaluated both in vitro and in vivo. The magnetic stirring was adopted as the preparation method and the conditions optimized by Design-Expert statistical software (Stat-Ease, Inc., Minneapolis, MN). The CAP-HP-β-CD was characterized using spectrometry of ultraviolet (UV) and infrared radiation (IR) as well as the differential scanning calorimetry (DSC). Additionally, the inclusion mechanism between CAP and HP-β-CD was preliminarily expounded by the measurement of phase solubility and the thermodynamic study. In supporting the fact that CAP possesses outstanding aqueous solubility and oral bioavailability, in vitro and in vivo studies were performed after the complex formation, coupled with the irritation test of gastric mucosa in rats.
Materials and methods
Chemicals and reagents
CAP (purity > 99.95%) was purchased from Zhengzhou Lion Biological Technology Co., Ltd (Henan, China). HP-β-CD was purchased from Xian Lide Biology and Chemical Engineering Co., Ltd (Shanxi, China). Alpha-naphthol and methanol (both are HPLC grade) were obtained from Sinopharm Chemical Reagent Co., Ltd (Shanghai, China). Acetonitrile (HPLC grade) was purchased from Honeywell Burdick & Jackson (Muskegon, MI). All other chemicals were of analytical reagent grade and obtained commercially. Double-distilled water was used throughout the study and produced by a Millipore water purification system (Millipore Corporation, Bedford, MA).
Instrumentation and chromatographic conditions
Concentrations of CAP and other samples were analyzed using HPLC with a Symmetric C18 column (5 μm, 4.6 × 150 mm2) at a detection wavelength of 280 nm at 30 °C. The injection volume was 20 μL.
In vitro release samples were also analyzed using the HPLC apparatus which included a pump (LC-20ATVP; Shimadzu) and an UV detector (SPD-20AVP; Shimadzu Corporation, Kyoto, Japan). The mobile phase consisted of methanol–water–1% phosphoric acid (pH 3.19 ± 0.02; 70:30) at a flow rate of 1.0 mL × min−1.
Blood samples were analyzed using a pump (LC-10AD; Shimadzu Corporation, Kyoto, Japan) and an UV detector (SPD-20 A; Shimadzu Corporation, Kyoto, Japan). The mobile phase was acetonitrile–water (43:57) at a flow rate of 1.0 mL × min−1.
Experimental animals
Healthy male Sprague–Dawley rats (about 300 g) were obtained from Laboratory Animal Center of Jiangsu University (Jiangsu, China). The rats were maintained under standard laboratory conditions with a dark/light cycle of 12 h. They were acclimatized to laboratory conditions for 3 d on standard dry pellet diet and water ad libitum. All rats were fasted overnight before the experiments, but allowed access to water ad libitum. The use and care protocols of rats were reviewed and approved by the Ethic Committee of Jiangsu University.
Selected method and optimization
There are five main methods employed (Branchu et al., Citation1999; Chen et al., Citation2010; Ghosh et al., Citation2011; Peroche et al., Citation2005; Sapkal et al., Citation2007; Zhou et al., Citation2012) in previous studies to prepare the inclusion complex of cyclodextrin, including precipitation and co-precipitation method (or known as saturated water solution method), spray-drying process, grinding method, magnetic stirring method, and ultrasonic method. The two former methods were exempted at the beginning due to its inappropriateness for the drug in this study. In selecting the optimum preparation parameters of inclusion complex among the other three procedures, the results of drug loading and encapsulation efficiency were used as the deciding criteria. They were calculated by the formulas shown below:
where DL and EE represent drug loading and encapsulation efficiency, respectively. M, M1, and M2 stand for mass of drug in the inclusion complex, mass of inclusion complex, and total mass of drug, respectively. The optimum condition was obtained using the experimental data and Design-Expert statistical software (Design-Expert 8.0.5 b, Stat-Ease, Inc., Minneapolis, MN).
The molar ratio is an important parameter of inclusion complex; simultaneously it has a close relationship with equilibrium constant. In this study, the measurement of molar ratio was performed using equimolar series method (also known as continuous variation method) with ultraviolet spectrophotometer.
The detailed method is as described below: 2.0 g CAP and 9.46 g HP-β-CD were accurately weighed into separate volumetric flasks and each dissolved with 1 mL ethyl alcohol. Aqueous solutions of CAP and HP-β-CD with the same concentration (0.655 mol × mL−1) were also each prepared by water dilution up to 100 mL. A series of mixtures were prepared with different molar ratios of CAP to HP-β-CD (1:3, 1:2, 2:3, 1:1, 3:2, 2:1, and 3:1, respectively). UV absorbance of each sample was measured at 280 nm. Similar molar ratios were set up for both CAP and HP-β-CD blank solutions by compensating with water. Then the absorbance difference (ΔA) for each sample was calculated and expressed as
where A1 is the UV absorbance change between the CAP-HP-β-CD and the HP-β-CD blank solution; and A2 is the UV absorbance change between the CAP-HP-β-CD and the CAP blank solution. The most appropriate molar ratio of CAP-HP-β-CD will be the largest value of ΔA. Every sample was prepared and measured in triplicate.
Characterization of CAP-HP-β-CD
Ultraviolet absorption spectrometry
Ultraviolet (UV) absorption spectrometry is one of the substance characterization criteria. In this study, three samples (free CAP, 1 mg; CAP-HP-β-CD, 5.878 mg; HP-β-CD, 4.878 mg) were weighed precisely and each diluted to 10 mL. After oscillation for 10 min, all samples were centrifuged at 3000 rpm for 10 min. Then an UV wavelength scanning between 200 and 400 nm was performed after millipore filtration (0.45 μm).
Infrared radiation spectroscopy
Detection of the presence of guest molecule in cyclodextrin is difficult when using infrared radiation (IR) spectroscopy. But the situation changes when the drug contains a carbonyl. The characteristic absorption peak of carbonyl between 1700 and 1680 cm−1 would be covered or shifted when the drug is wrapped up in cyclodextrin. The IR spectra were recorded in the 4000–400 cm−1 region using tablets prepared with pressed-disk technique (2 mg sample + 600 mg KBr).
Differential scanning calorimetry
Thermal analysis can distinguish the inclusion complex from the physical mixture and further represent the specific heat effect induced by molecular clathration. According to the physical–chemical properties of CAP, the DSC was suitable for CAP-HP-β-CD. Therefore, the measurement of DSC curves was performed with a heating rate of 10 °C × min−1 in a temperature ranging from 25 to 200 °C, and 2 mg each of CAP, HP-β-CD, CAP/HP-β-CD, and CAP-HP-β-CD was weighed.
Phase solubility and thermodynamic study
The phase solubility was used in establishing the effect of HP-β-CD on the solubility of CAP. Briefly, a series of aqueous solutions of HP-β-CD (from 0% to 16%) were prepared and supersaturated with excess CAP. After shaking for 10 min, the various concentrations were sealed and put into oscillator with thermostatic water bath at different independent temperatures (25 °C, 37 °C, and 45 °C) concurrently for 3 d at a rotating speed of 120 rpm. The supernatant (100 μL) was diluted in water to a volume of 10 mL. Then microporous membrane (0.45 μm) filtration was used to filter the resulting solutions. Triplicate samples of each filtrate were injected into the HPLC as described under the instrumentation and chromatographic conditions. Subsequently, the phase solubility graphs at different temperatures were obtained with the concentration of CAP (ordinate) and HP-β-CD (abscissa).
In vitro release study of CAP-HP-β-CD
Dissolution test for determining the drug release was established according to the second method in appendix XC of Chinese Pharmacopoeia (2010 edition). All materials (CAP, HP-β-CD, CAP-HP-β-CD and CAP/HP-β-CD) each containing 40 mg of CAP was precisely weighed. The dissolution tester was employed at 100 rpm with a temperature of 37 °C. Each material was added to the dissolving media including HCl (pH 1.2, 600 mL) and H3PO4 (pH 7.4, 600 mL) solutions.
Dissolved samples (approximately 3 mL) were collected at 1, 3, 5, 10, 20, 40, and 60 min right after the addition of materials. The products were subject to HPLC analysis following millipore filtration (0.45 μm) as described under the instrumentation and chromatographic conditions. The cumulative release rates at different times were calculated using drug concentrations obtained according to the calibration curve.
In vivo pharmacokinetic study
Intragastric administration
Twelve rats were randomly and equally divided into two groups. Free CAP (serving as control) and CAP-HP-β-CD were each separately suspended in 0.5% (w/v) CMC–Na solution to obtain a final concentration of 9 mg × mL−1. The two groups of rats were respectively given CAP at a dose of 90 mg × kg−1 and inclusion complex, corresponding to a dose of 90 mg × kg−1 CAP, via gavage. After oral administration, the blood samples (0.6 mL) were collected at the desired times (5, 15, 30 min, 1, 2, 4, 6, 8, and 12 h) into heparinized centrifuge tubes and immediately centrifuged at 8000 rpm for 10 min.
Treatment of plasma sample
A 200 μL aliquot of plasma was mixed with 50 μL α-naphthol (10 μg × mL−1; internal standard, IS), 10 μL methanol, 400 μL water, and 500 μL acetonitrile, and vortexing for 1 min. The solution was then extracted with 1.5 mL each of acetic ether and cyclohexane for CAP, followed by vortexing for 3 min. After 10 min centrifugation at 3000 rpm, 2.5 mL of the supernatant was transferred to another container and evaporated to dryness via nitrogen using a 37 °C water bath. The residue was dissolved in 100 μL of methanol and mixed for 1 min. Following centrifugation at 3000 rpm for 5 min, 20 μL of the supernatant was injected into the HPLC system for analysis.
Data analysis
Pharmacokinetic parameters for CAP in rats were calculated by using BAPP 2.3 pharmacokinetic software package (supplied by the Center of Drug Metabolism and Pharmacokinetics, China Pharmaceutical University). The relative bioavailability (Fr) was calculated according to the following equation:
where T means the tested preparation, and R means the reference preparation. All statistical analysis was carried out using Student’s t-test and one-way analysis of variance with the least significant difference post hoc test to compare the two data sets.
Gastric mucosa irritation test
The gastric mucosa irritation test was performed in rats divided into three groups (n = 3) for intragastric administration. The division included physiological saline (10 mL × kg−1), suspension of free CAP group (90 mg × kg−1) and aqueous solution of CAP-HP-β-CD group (with 90 mg × kg−1 content of CAP). The rats were sacrificed for anatomy after 2 h of oral administration, and the intact stomachs were removed after ligations of pylorus and cardia. All the samples were then fixed with 10% formaldehyde solution, dehydrated, and embedded in paraffin. The total gastric histopathological sections were observed under light microscope (Olympus CKX41, Olympus, Miami, FL) equipped with computer-controlled digital camera after HE staining.
Results
Choice of method and its optimization
Drug loading and encapsulation efficiency are the two most important parameters for evaluating the quality of inclusion complex. presents the results of these two parameters for CAP-HP-β-CD as prepared by the different methods. The inclusion complex obtained via the grinding method gave lower drug loading (4.46%) and encapsulation efficiency (44.74%) values. However, other methods including ultrasonic (6.31% and 63.88%) and magnetic stirring (6.65% and 68.22%) recorded higher values for the respective two parameters. The value of ΔA was the greatest when the molar ratio of CAP to HP-β-CD is 1:1, which implies that the change in ultraviolet absorption was the maximum ().
Table 1. The drug loading and encapsulation efficiency of CAP-HP-β-CD inclusion complexes prepared by different methods (n = 3).
Table 2. Results of inclusion ratio of inclusion complex by continuous variation method (n = 3).
Characterization of inclusion complex
The following indices including UV, IR, and DSC can be used to verify the successful loading of CAP using HP-β-CD. The changes in UV absorption spectrum of CAP for the different samples are as shown in . The solubility of CAP in the aqueous solution was greatly improved after adding HP-β-CD, which resulted in very high UV absorption spectrum of CAP-HP-β-CD along the other samples.
Figure 2. The ultraviolet absorption spectrum of CAP in aqueous solution before and after the adding of HP-β-CD: (a) CAP-HP-β-CD; (b) CAP; (c) HP-β-CD.
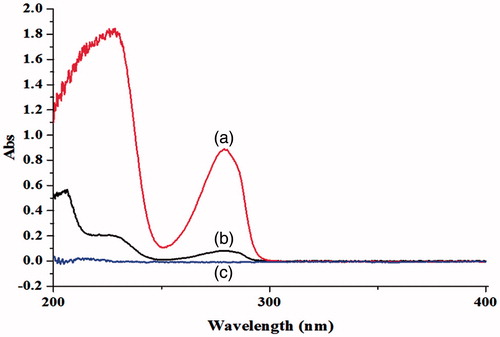
demonstrates the IR spectra of CAP, HP-β-CD, CAP-HP-β-CD complex and CAP/HP-β-CD mixture. The free drug (CAP) possesses a clear carbonyl stretching vibration peak in 1680–1700 cm−1, but the absorption peak of its physical mixture became less because of HP-β-CD, and this peak even disappeared in the inclusion complex.
Figure 3. The IR spectra of CAP, HP-β-CD, CAP-HP-β-CD inclusion complex, and physical mixture of CAP/HP-β-CD.
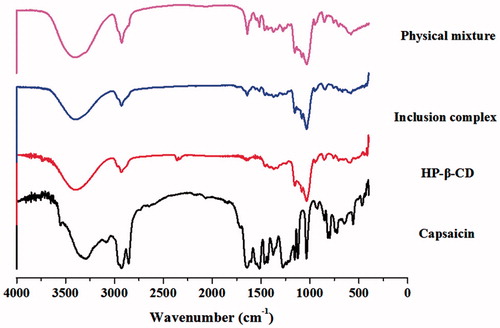
The DSC-thermograms of the various materials are as shown in . There was an obvious endothermic peak of CAP around 59 °C, and a broad dehydrated peak of HP-β-CD appeared around 83 °C. The two peaks coexisted in the form of superposition with less intensity in the DSC-thermogram of the physical mixture. However, there was only an endothermic peak of HP-β-CD in the DSC curve of CAP-HP-β-CD.
Preliminary study on formation mechanism of CAP-HP-β-CD
The equilibrium phase solubility diagrams of CAP in different concentrations of HP-β-CD at different temperatures are as shown in . The concentration of CAP increased with that of HP-β-CD and the test temperature. The higher concentrations of either HP-β-CD or the temperature provided a better solubility for CAP. There was even a 147.77-fold increase in solubility of CAP in the aqueous solutions of HP-β-CD with a concentration of 16% at 37 °C (data not shown).
Figure 5. The equilibrium phase solubility diagrams of CAP with different concentrations of HP-β-CD in distilled water at different temperatures (n = 3).
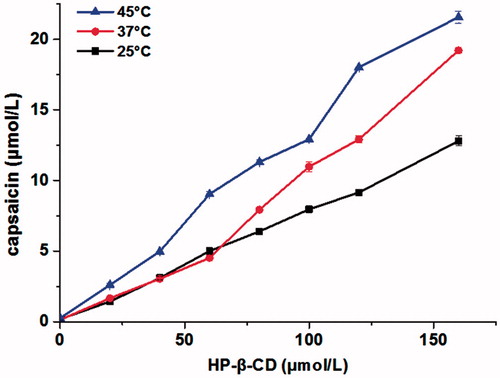
The linearity of equilibrium phase solubility diagram for CAP with various HP-β-CD concentrations at different temperatures was analyzed using linear regression and the resulted equations are listed in . In addition, the thermodynamic parameters of CAP-HP-β-CD at different temperatures were also shown in . The determination of K value at different temperatures can be used for thermodynamic study on the reaction of CAP and HP-β-CD. Therefore, the coefficients of determination (R2) were 0.998 (25 °C), 0.978 (37 °C), and 0.998 (45 °C), which indicated that the phase solubility diagram possessed a good linearity. Here, the K value was calculated from the slope of phase solubility diagram according to the following equation:
where S0 is the intrinsic solubility of CAP at different temperatures in the absence of HP-β-CD (Ghosh et al., Citation2011).
Table 3. Thermodynamic parameters of CAP-HP-β-CD inclusion complex at different temperatures.
Change in Gibbs free energy (ΔG) is a criterion for ascertaining whether the reaction occurred spontaneously, which was calculated according to the equation (Pollard, Citation2010) .
Van’Hoff equation:
is also very useful in calculating the other two thermodynamic parameters, enthalpy change (ΔH) and entropy change (ΔS), which are the criteria for reflecting the spontaneity of reactions. Both parameters were calculated according to the regression equation Y = −2122X + 13.32 obtained from the linear regression of Van’Hoff equation, where X and Y were 1/T and ln K, respectively.
In vitro release study of CAP-HP-β-CD
In this study, the dissolution rates were calculated according to the regulations of Chinese Pharmacopoeia (2010 edition), and the dissolution profiles of CAP from different samples are shown in . The dissolution rate of CAP from CAP-HP-β-CD was clearly higher and faster than that of CAP/HP-β-CD mixture and CAP (). Thus, the cumulative release of free CAP was 4.78% in HCl solution (pH 1.2) and 10.22% in H3PO4 solution (pH 7.4) within 60 min. Additionally, the cumulative release of CAP from the CAP/HP-β-CD mixture in both vehicles (pH 1.2 and 7.4) was virtually stable and low (only about 8.5%). However, even though the cumulative release of CAP from the CAP-HP-β-CD was similar in the two vehicles, there was an enhanced acceleration and later some level of stability. Thus, the cumulative release reached 85% in 1 min and subsequently more than 98% within 3 min.
In vivo pharmacokinetic analysis of CAP-HP-β-CD
and show the changes and differences between CAP and CAP-HP-β-CD based on the pharmacokinetic parameters in the plasma of CAP after intragastric administration. Free CAP was absorbed quickly and the maximum concentration (Cmax, 737.94 ± 36.77 ng × mL−1) came at 0.50 h (tmax). CAP-HP-β-CD had a higher Cmax (1117.57 ± 106.55 ng × mL−1) with a tmax of 0.53 h. Regarding the AUC, the AUC0–12 h was enhanced from 4007.37 to 5585.66 ng × h × mL−1 and that of AUC0–n increased from 5285.9 to 7409.8 ng × h × mL−1. In addition, the relative oral bioavailability of CAP-HP-β-CD was 139.38% as compared with the free CAP. The standard curve of CAP was calculated (data not shown) using the least square method and the regression equation was Y = 0.0001X − 0.0036 (R2 = 0.99947, n = 3) with a linear range of 125–4000 ng × mL−1. Here, the X and Y refer to the alconcentration of CAP in plasma and As/Ai (peak area ratio of CAP and IS obtained from HPLC), respectively (Wu et al., Citation2014).
Table 4. Pharmacokinetic parameters after intragastric administration with compartmental model (mean, n = 6).
Gastric mucosa irritation test of CAP-HP-β-CD
As shown in , the normal organizational structure (physiological saline treatment group) of gastric mucosa was intact and smooth, and the cellular morphologies were clear and aligned. However, the photomicrograph of free CAP treatment group was different from the saline group. An obvious irritation was observed, such as the incomplete organizational structure, inflammatory cell infiltrations, cellular structures disruption, and cell nucleus appeared rhexis and pyknosis. With the inclusion complex treatment group, its organizational structure was still comparatively intact, mostly clear cellular morphologies, no appearance of inflammatory cell infiltration and only a slight observed pyknosis of the cell nucleus.
Discussion
The results of drug loading and encapsulation efficiency of CAP-HP-β-CD based on the different methods indicated that magnetic stirring was the best choice for preparing the inclusion complex. The optimized preparation obtained using the software was as described below: CAP and HP-β-CD, with mass and molar ratios of 4.878:1 and 1:1, respectively, were mixed for 3.18 h on water bath at 79.2 °C. The resulted CAP-HP-β-CD gave the best encapsulation efficiency of 75.83% with the best drug loading of 7.44%.
The results () indicated that the acting forces present in CAP-HP-β-CD (the molar ratio is 1:1) were the strongest which agrees with previous report investigating oxybutynin-HP-β-CD inclusion complex using similar molar ratio (Zhang et al., Citation2011). The inclusion process is a reversible equilibrium reaction that can be promoted by increasing the molar ratio of HP-β-CD to CAP, which leads to an increase in the encapsulation efficiency. Simultaneously, the drug loading is expected to decrease in this situation. Consequently, the two parameters were co-referred in this study as standards in choosing and optimizing the preparation condition. The software gave a simulative result which pointed out once again that the optimal molar ratio of CAP to HP-β-CD was 1:1.
The UV absorption spectrum demonstrated that clathration has occurred between HP-β-CD and CAP. In addition, a red shift was observed at the maximum absorption wavelength, which is the most common phenomenon in inclusion complex (Gassensmith et al., Citation2007; Zhou et al., Citation2009). The similar IR spectra () of HP-β-CD and inclusion complex indicate the formation of new phase between CAP and HP-β-CD (Bernard et al., Citation2012), and the DSC-thermograms () demonstrated that a new phase which is different from the other three substances has been formed (Velasco et al., Citation2004).
The equilibrium phase solubility diagrams () implied that the water solubility of CAP was improved by encapsulating it in HP-β-CD which supports other studies (Taupitz et al., Citation2013). The solubility also increased in proportion to the concentration of HP-β-CD at different temperatures. According to the classification method of Higuchi and Connors (Chen et al., Citation2007; Higuchi & Connors, Citation1965), the phase solubility diagram of the CAP-HP-β-CD system matches AL type, which demonstrated that the inclusion complex (with molar ratio of 1:1) agrees with the previous optimal conditions.
The equilibrium constant (K) is one of the most important parameters in the pharmaceutical research, which determines the properties of inclusion complex. The measurement of K value can quantitatively describe the equilibrium process and reflect the affinity between cyclodextrin and drug molecule. Generally, the stability of inclusion complex is in direct proportion to K value. Therefore, the results () indicated that the CAP-HP-β-CD was relatively stable. Moreover, the calculations showed that ΔG < 0, ΔH < 0, ΔS > 0 in the inclusion reaction process. These findings agree with the fact that the inclusion reaction did not only occur spontaneously but also was based on enthalpy and entropy dual driven mechanism (Cheneke et al., Citation2012). Additionally, the values of both ΔH and ΔS were less than those witnesses in the usual chemical reactions, which further indicated that the inclusion reaction is a physical process (Li et al., Citation2004). Consequently, all forms of chemical bonds, such as covalent bonds, ionic bonds, and coordination bonds, were non-existent in the formation process of CAP-HP-β-CD. Thus, the inclusion process had no influence on the chemical properties of the drug.
Nevertheless, there were three acting forces in the inclusion reaction of host (HP-β-CD) and guest molecules (CAP), including hydrophobic interaction, Van der Waals' force and hydrogen bond (Okubo & Kitano, Citation1989). Based on the relationship between the thermodynamic parameters and the respective driving forces (), it can be concluded that the possible mechanism associated with the inclusion reaction was hydrophobic interaction, which has a direct link with the holey structure of HP-β-CD. This specific holey structure possesses hydrophobicity (inside) and hydrophility (outside). Therefore, there was a synergistic effect between the hydrophobicity of CAP and HP-β-CD to replace the water molecules inside the holey structure of HP-β-CD with the CAP molecule. All these reactions caused an increase in the number of free moving molecules which was the main factor of enhancive entropy. Besides, the encapsulation process might occur with other factors like certain chemical groups and substituents (such as nitro group and amino group) which could promote the inclusion complex formation. Therefore, the comprehensive details of inclusion mechanism still need further studies.
Table 5. Relationship between thermodynamic parameters and types of driving force.
Generally, slightly soluble drug possesses a poor bioavailability because of low absorption in vivo, which is limited by its dissolution rate (Liu et al., Citation2013; Sapkal et al., Citation2007). Therefore, a good bioavailability is usually obtained by improving its dissolution and release rate right from the beginning. The results of in vitro release study demonstrated that the dissolution rate of CAP could be improved significantly by the use of inclusion technique. Thus, the aqueous solubility of CAP was clearly enhanced because of the solubilizing action of HP-β-CD, and the decrease in crystallinity of the drug which could be another reason (Szejtli, Citation1994).
Focusing on the purpose of improving the bioavailability and absorption of CAP, one prerequisite was that the pharmacokinetic parameters of intragastric administration should be known in establishing the basic kinetic knowledge (Chen et al., Citation2010). The results of in vivo pharmacokinetic analysis implied that HP-β-CD could promote the in vivo absorption of CAP. However, there was no significant difference between free CAP and CAP-HP-β-CD for t1/2, MRT, and tmax, as well as the decreasing trend of the concentration-time graph after 1 h of oral administration. A brief comparison of CAP inclusion complex and previously reported micelles formulation (Zhu et al., Citation2014) is presented in . From the obtained data, the Cmax of free CAP in both studies were similar, but that of CAP-HP-β-CD was higher than CAP-loaded micelles. Although the relative bioavailability and encapsulation efficiency of CAP inclusion complex is somehow lower than CAP-loaded micelles, CAP-HP-β-CD possesses its own advantages as a conventional dosage form. For instance, the most prominent characteristics of this formulation include its easy preparation and less consuming raw materials, which make it suitable for industrial production. In addition, this study also provided valuable information on CAP, such as inclusion mechanism and bioavailability. Relating to the gastric mucosa irritation test, its findings illustrated that CAP-HP-β-CD can reduce the gastric mucosa irritation of CAP effectively.
Table 6. Comparison of CAP HP-β-CD inclusion complex and micelle previously reported by our group.
Conclusion
The preparation, characterization, and in vivo and in vitro studies of CAP-HP-β-CD were successfully carried out in this study, as well as gastric irritation test and simple showcasing of inclusion mechanism. The findings indicated that CAP-HP-β-CD possesses not only excellent solubilizing power and oral bioavailability in vivo but also minimal irritation effect. In throwing more light on the above report, further pharmacokinetic and mechanistic studies in vivo are currently under to provide more comprehensive information about the application of CAP and its inclusion complex.
Declaration of interest
This work was supported by the National Natural Science Foundation of China (30973677, 81373371), National “Twelfth Five-Year” Plan for Science & Technology Support (2012BAD36B01), the Doctoral Fund of Ministry of Education of China (20113227110012), Special Funds for 333 (BRA2013198) and 331 projects, Industry-University-Research Institution Cooperation (JHB2012-37, GY2012049, and GY2013055) in Jiangsu Province and Zhenjiang City, and China Postdoctoral Science Foundation (2014M551523). In addition, the authors also thank the Jiangsu University Ethics Committee for the kind guidance in the animal experiments. The authors report that they have no conflicts of interest.
References
- Bencsik P, Kupai K, Giricz Z, et al. (2008). Cardiac capsaicin-sensitive sensory nerves regulate myocardial relaxation via S-nitrosylation of SERCA: Role of peroxynitrite. Br J Pharmacol 153:488–96
- Bernard J, Seidl M, Mayer E, Loerting T. (2012). Formation and stability of bulk carbonic acid (H2CO3) by protonation of tropospheric calcite. Chemphyschem 13:3087–91
- Branchu S, Forbes RT, York P, et al. (1999). Hydroxypropyl-beta-cyclodextrin inhibits spray-drying-induced inactivation of beta-galactosidase. J Pharm Sci 88:905–11
- Caterina MJ, Schumacher MA, Tominaga M, et al. (1997). The capsaicin receptor: A heat-activated ion channel in the pain pathway. Nature 389:816–24
- Chen S, Zhu J, Ma F, et al. (2007). Preparation and characterization of solid dispersions of dipyridamole with a carrier “copolyvidonum Plasdone S-630”. Drug Dev Ind Pharm 33:888–99
- Chen XY, Sun X, Ren K, et al. (2010). Enhanced aqueous solubility and bioavailability of capsaicin by the preparation of an inclusion complex. Arzneimittel-Forsch 60:571–4
- Cheneke BR, Indic M, van den Berg B, Movileanu L. (2012). An outer membrane protein undergoes enthalpy-and entropy-driven transitions. Biochemistry 51:5348–58
- de Araujo DR, Tsuneda SS, Cereda CM, et al. (2008). Development and pharmacological evaluation of ropivacaine-2-hydroxypropyl-beta-cyclodextrin inclusion complex. Eur J Pharm Sci 33:60–71
- de Paula WX, Denadai AML, Santoro MM, et al. (2011). Supramolecular interactions between losartan and hydroxypropyl-beta-CD: ESI mass-spectrometry, NMR techniques, phase solubility, isothermal titration calorimetry and anti-hypertensive studies. Int J Pharm 404:116–23
- Del Valle EMM. (2004). Cyclodextrins and their uses: A review. Process Biochem 39:1033–46
- Fraceto LF, Grillo R, Sobarzo-Sanchez E. (2014). Cyclodextrin inclusion complexes loaded in particles as drug carrier systems. Curr TopMed Chem 14:518–25
- Gassensmith JJ, Arunkumar E, Barr L, et al. (2007). Self-assembly of fluorescent inclusion complexes in competitive media including the interior of living cells. J Am Chem Soc 129:15054–9
- Ghosh A, Biswas S, Ghosh T. (2011). Preparation and evaluation of silymarin beta-cyclodextrin molecular inclusion complexes. J Young Pharmacists 3:205–10
- Higuchi T, Connors KA. (1965). Phase-solubility techniques. Adv Chem Instrum 4:117–212
- Joo JI, Kim DH, Choi JW, Yun JW. (2010). Proteomic analysis for antiobesity potential of capsaicin on white adipose tissue in rats fed with a high fat diet. J Proteome Res 9:2977–87
- Li J, Xiao H, Li J, Zhong Y. (2004). Drug carrier systems based on water-soluble cationic beta-cyclodextrin polymers. Int J Pharm 278:329–42
- Liu QY, Zhang ZH, Jin X, et al. (2013). Enhanced dissolution and oral bioavailability of tanshinone IIA base by solid dispersion system with low-molecular-weight chitosan. J Pharm Pharmacol 65:839–46
- Lu HF, Chen YL, Yang JS, et al. (2010). Antitumor activity of capsaicin on human colon cancer cells in vitro and colo 205 tumor xenografts in vivo. J Agric Food Chem 58:12999–3005
- Menendez L, Lastra A, Hidalgo A, Baamonde A. (2004). The analgesic effect induced by capsaicin is enhanced in inflammatory states. Life Sci 74:3235–44
- Miyake K, Arima H, Hirayama F, et al. (2000). Improvement of solubility and oral bioavailability of rutin by complexation with 2-hydroxypropyl-beta-cyclodextrin. Pharm Dev Tech 5:399–407
- Okubo T, Kitano H. (1989). Inclusion process of ionic detergents with cyclodextrins as studied by the conductance stopped-flow method. J Phys Chem 93:3721–3
- Peroche S, Degobert G, Putaux JL, et al. (2005). Synthesis and characterisation of novel nanospheres made from amphiphilic perfluoroalkylthio-beta-cyclodextrins. Eur J Pharm Biopharm 60:123–31
- Pollard TD. (2010). A guide to simple and informative binding assays. Mol Biol Cell 21:4061–7
- Sapkal NP, Kilor VA, Bhusari KP, Daud AS. (2007). Evaluation of some methods for preparing gliclazide beta-cyclodextrin inclusion complexes. Trop J Pharm Res 6:833–40
- Sharma MC, Sharma S. (2010). Studies on the preparation, characterization, solubility and stability of cefadroxil-beta-cyclodextrin inclusion complexes. J Optoelectron Adv M 12:411–15
- Shulman M, Cohen M, Soto-Gutierrez A, et al. (2011). Enhancement of naringenin bioavailability by complexation with hydroxypropoyl-beta-cyclodextrin. PloS One 6:e18033
- Strickley RG. (2004). Solubilizing excipients in oral and injectable formulations. Pharm Res 21:201–30
- Szallasi A, Blumberg PM. (1999). Vanilloid (capsaicin) receptors and mechanisms. Pharmacol Rev 51:159–212
- Szejtli J. (1994). Medicinal applications of cyclodextrins. Med Res Rev 14:353–586
- Taupitz T, Dressman JB, Buchanan CM, Klein S. (2013). Cyclodextrin–water soluble polymer ternary complexes enhance the solubility and dissolution behaviour of poorly soluble drugs. Case example: Itraconazole. Eur J Pharm Biopharm 83:378–87
- Vega E, Egea MA, Calpena AC, et al. (2012). Role of hydroxypropyl-beta-cyclodextrin on freeze-dried and gamma-irradiated PLGA and PLGA-PEG diblock copolymer nanospheres for ophthalmic flurbiprofen delivery. Int J Nanomed 7:1357–71
- Velasco J, Andersen ML, Skibsted LH. (2004). Evaluation of oxidative stability of vegetable oils by monitoring the tendency to radical formation: A comparison of electron spin resonance spectroscopy with the Rancimat method and differential scanning calorimetry. Food Chem 85:623–32
- Wang YQ, Han BH. (2013). Cyclodextrin-based porous nanocapsules. Chin J Chem 31:569–76
- Wu QB, Li HT, Leng YJ, et al. (2014). Determination of muscone in rats plasma following oral administration of artificial musk: Using of combined headspace gas chromatography-mass spectrometry. J Spectrosc 2014:906–28
- Yuvaraja K, Khanam J. (2014). Enhancement of carvedilol solubility by solid dispersion technique using cyclodextrins, water soluble polymers and hydroxyl acid. J Pharm Biomed 96:10–20
- Zhang JX, Ma PX. (2013). Cyclodextrin-based supramolecular systems for drug delivery: Recent progress and future perspective. Adv Drug Deliv Rev 65:1215–33
- Zhang PL, Pan CY, Tang KW, Li HJ. (2011). Inclusion behavior of oxybutynin with hydroxypropyl-beta-cyclodextrin. J Cent South Univ T 18:1897–901
- Zhou CJ, Li LF, Liu Y, et al. (2012). Study on the inclusion complex of rutin/sulfobutylether-beta-cyclodextrin. Future Mater Res Ind Appl Parts 1 and 2, 455–6, 1177–81
- Zhou YY, Sun JY, Yu HP, et al. (2009). Inclusion complex of riboflavin with cucurbit[7]uril: Study in solution and solid state. Supramol Chem 21:495–501
- Zhu Y, Peng W, Zhang JJ, et al. (2014). Enhanced oral bioavailability of capsaicin in mixed polymeric micelles: Preparation, in vitro and in vivo evaluation. J Funct Foods 8:358–66