Abstract
Context: Alzheimer’s disease (AD) is a devastating neurodegenerative disorder that affects millions of elderly people worldwide. However, no efficient therapeutic method for AD has yet been developed. Recently, Salvia miltiorrhiza Bunge (Lamiaceae), a well-known traditional Chinese medicine which is widely used for treating cardio-cerebrovascular, exerts multiple neuroprotective effects and is attracting increased attention for the treatment of AD.
Objective: The objective of this study is to discuss the neuroprotective effects and neurogenesis-inducing activities of S. miltiorrhiza components.
Methods: A detailed search using major electronic search engines (such as Pubmed, ScienceDirect, and Google Scholar) was undertaken with the search terms: Salvia miltiorrhiza, the components of S. miltiorrhiza such as salvianolic acid B, salvianolic acid A, danshensu, tanshinone I, tanshinone IIA, cryptotanshinone, dihydrotanshinone, and neuroprotection.
Results: Salvia miltiorrhiza components exert multiple neuroprotective potentials relevant to AD, such as anti-amyloid-β, antioxidant, anti-apoptosis, acetylcholinesterase inhibition, and anti-inflammation. Moreover, S. miltiorrhiza promotes neurogenesis of neural progenitor cells/stem cells in vitro and in vivo.
Conclusions: The properties of S. miltiorrhiza indicate their therapeutic potential in AD via multiple mechanisms. In addition, S. miltiorrhiza provides lead compounds for developing new drugs against AD.
Introduction
Alzheimer’s disease (AD) is an age-related neurodegenerative disorder characterized by progressive cognitive and memory impairment, senile plaques, neurofibrillary tangles, and neuronal cell death (Cummings, Citation2004). Although the etiology of AD remains unclear, a number of hypotheses have been proposed to explain the pathogenesis of AD, such as the amyloid hypothesis, oxidative stress hypothesis, and APOE genotype (Butterfield, Citation2002; Butterfield et al., Citation2007; Huebbe et al., Citation2007). The accumulated amyloid-β (Aβ) is widely regarded as the primary pathogenic species causing AD (Kim & Tsai, Citation2009). The deposition of abnormal Aβ peptides causes oxidative injury and inflammation, which contributes to AD pathogenesis. Therefore, Aβ, inflammation, and reactive oxygen species have become potential targets of AD therapy (Helmuth, Citation2002). Memory impairment is related to cholinergic neuronal dysfunction in AD (Coyle et al., Citation1983; Whitehouse et al., Citation1981), thus improving cholinergic function is a therapeutic target in treating AD. Two classes of drugs have been approved to treat AD: acetylcholinesterase inhibitors, such as tacrine, donepezil, galantamine, and rivastigmine, and an N-methyl-d-aspartate receptor antagonist, memantine. The former protects the neurotransmitter acetylcholine against breaking down and compensates for the loss of neurons, and the latter blocks the action of another neurotransmitter glutamate, which is overproduced in AD brains and can overexcite neurons to death. Both types of drugs cannot stop progressive neuritic dystrophy, thus limiting their clinical efficacy (Helmuth, Citation2002). Therefore, alternative agents to treat AD are necessary.
The roots of Salvia miltiorrhiza Bunge (Lamiaceae) (known as “danshen” in Chinese) have been widely used to treat cardiovascular and cerebrovascular diseases (Zhou et al., Citation2005). According to their structural characteristics, the main components of S. miltiorrhiza can be classified into two groups. The first group contains water-soluble polyphenolic compounds such as salvianolic acid B (Sal B), salvianolic acid A (Sal A), and danshensu. The second group contains lipophilic chemicals, such as tanshinone I, tanshinone IIA, cryptotanshinone, and dihydrotanshinone (Chen et al., Citation2006; Ren et al., Citation2004; Wang et al., Citation2007). Their corresponding structures are presented in . Both groups contribute to the biological activities of S. miltiorrhiza. Studies have shown that S. miltiorrhiza components have multiple neuroprotective potentials that are relevant to AD, such as anti-Aβ (Cao et al., Citation2013; Durairajan et al., Citation2008; Mei et al., Citation2009), antioxidant (Wang & Xu, Citation2005), anti-apoptosis (Chen et al., Citation2012; Tian et al., Citation2008), anti-inflammation (Chen et al., Citation2007; Jiang et al., Citation2013; Joe et al., Citation2012), and enhancement of cholinergic signaling (Kim et al., Citation2007; Liu & Wu, Citation1999; Wong et al., Citation2010). In addition, S. miltiorrhiza induces neurogenesis of neural progenitor cells/stem cells in vitro and in vivo (Guo et al., Citation2010; Hu et al., Citation2011; Zhang et al., Citation2012; Zhuang et al., Citation2012). Given that S. miltiorrhiza has been widely used in clinical practice, this plant has important implications in treating AD.
Neuroprotective effects of S. miltiorrhiza
Accumulated evidence suggests that many components of S. miltiorrhiza such as Sal B, Sal A, danshensu, tanshinone I, tanshinone IIA, cryptotanshinone, and dihydrotanshinone have the activities of inhibiting Aβ aggregation, antioxidant, anti-inflammation, enhancing cholinergic signaling, and decreasing cell apoptosis (), and exert neuroprotective effects.
Figure 2. Schematic overview of some neuroprotective pathways of Salvia miltiorrhiza. STC inhibit Aβ aggregation and amyloid plaques formation; Cts increases α-secretase activity and promotes sAPPα generation; Tan IIA decreases tau phosphorylation via calcium and p35/cdk5 pathways; Sal A, Sal B, SBE, Tan IIA, and Danshensu exhibit neuroprotective effects against oxidative injury via multiple pathways and decrease apoptosis. Aβ, amyloid-β; STC, salvianolic acid A salvianolic acid B, tanshinone I, tanshinone IIA, and cryptotanshinone; Cts, cryptotanshinone; Tan IIA, tanshinone IIA; Sal A, salvianolic acid A; Sal B, salvianolic acid B; SBE, salvianic borneol ester.
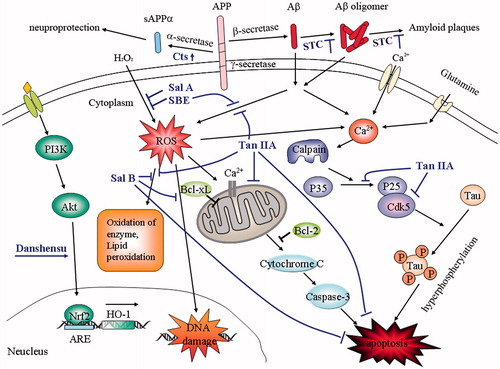
Anti-Aβ
AD is characterized by extracellular plaques of Aβ peptide aggregates, intraneuronal accumulation of neurofibrillary tangles, and neural loss (Mott & Hulette, Citation2005). Abnormal Aβ aggregation may be the primary cause of AD pathogenesis (Hardy & Selkoe, Citation2002). Senile plaques are formed when amyloid precursor protein (APP) is cleaved to Aβ peptides by β- and γ-secretase, and then soluble Aβ monomers aggregate to β-sheet-rich oligomers and insoluble amyloid fibrils. As such, any step involved in the processes of Aβ production, aggregation, and clearance is a potential therapeutic target for anti-AD. Several components derived from S. miltiorrhiza have exhibited anti-Aβ effects. For example, Sal B, the most abundant salvianolic acid in S. miltiorrhiza (Zhong et al., Citation2009), inhibits Aβ1–40 aggregation, destabilizes preformed Aβ fibrils, and prevents Aβ-induced cytotoxicity in human neuroblastoma SH-SY5Y cells (Durairajan et al., Citation2008). Meanwhile, Sal A inhibits Aβ aggregation by blocking the formation of β-sheets from α-helices, disaggregating preformed Aβ fibrils, protecting SH-SY5Y cells against Aβ42-induced toxicity, and alleviating Aβ-induced paralysis in transgenic Caenorhabditis elegans (Rhabditida: Rhabditidae) strain CL4176 (Cao et al., Citation2013). These results suggest that Sal A and B have therapeutic potential in the treatment of AD.
Tanshinones have been used to improve blood circulation in the treatment of cardiovascular diseases in China. A recent report suggested that tanshinone I and tanshinone IIA can inhibit amyloid formation, disassemble Aβ fibrils, and protect SH-SY5Y cells from Aβ-induced toxicity (Wang et al., Citation2013). Further analysis revealed that tanshinones I and IIA prefer to bind to the C-terminal β-sheet of Aβ pentamer and prevent Aβ aggregation (Wang et al., Citation2013). In addition, cryptotanshinone also inhibits Aβ aggregation and reduces Aβ-induced cytotoxicity in SH-SY5Y cells (Mei et al., Citation2012). Thus, tanshinone I, tanshinone IIA, and cryptotanshinone are likely to be promising therapeutics for AD.
Aside from Aβ peptide, which is a major component of senile plaques, APP can also be cleaved by α-secretase within the Aβ sequence. The cleaved products then generate a secreted APP fragment (sAPPα) and preclude Aβ generation. Thus, inhibiting Aβ generation by modulating APP proteolysis is regarded as a potential target for AD therapy (Haass, Citation2004). Cryptotanshinone decreases amyloid plaque deposition and improves spatial learning and memory of APP/presenilin-1 transgenic mice (Mei et al., Citation2009). Further studies have shown that cryptotanshinone promotes APP metabolism toward the non-amyloidgenic product pathway by activating the phosphatidylinositol 3-kinase (PI3K) pathway, upregulating ADAM1 protein expression, and increasing α-secretase activity (Mei et al., Citation2010). In addition, sAPPα exhibits various neurotrophic and neuroprotective effects (Mattson, Citation1997), such as promoting neural survival and improving synapse formation and long-term potentiation (Taylor et al., Citation2008; Zhang et al., Citation2009).
Aβ, glutamate, and oxidative stress can cause calcium influx, thus activating calpain (a calcium-activated proteolytic enzyme), which induces the cleavage of p35 to p25. The binding of p25 and cyclin-dependent kinase 5 (cdk5) leads to hyperphosphorlating tau, which induces apoptosis in primary neurons (Lee et al., Citation2000). Tanshinone IIA protects neurons against Aβ25–35-induced cytotoxicity by increasing neuron viability, decreasing phosphorylated tau expression, reducing p35 to p25 conversion, inhibiting cdk5 translocation from the nucleus into the cytoplasm, and suppressing cdk5 activity. These protective functions may involve the calcium and p35/cdk5 pathways (Shi et al., Citation2012).
Antioxidant
Although the mechanisms involved in Aβ-mediated neurotoxicity remain unclear, studies have shown that Aβ directly causes reactive oxygen species (ROS) production. ROS cause DNA damage, enzyme oxidation, lipid peroxidation, and cell apoptosis. Thus, ROS have been implicated in AD pathogenesis (Radak et al., Citation2011) and have become research targets in preventing and treating AD. Using antioxidants is a promising preventive or therapeutic strategy to suppress oxidative-dependent and Aβ-mediated cytotoxicity. Tanshinone IIA has been found to protect rat cortical neurons against Aβ25–35-induced oxidative stress and apoptosis by inhibiting lipid peroxidation, antioxidant enzyme activities and ROS generation, decreasing mitochondrial membrane potential, and releasing cytochrome c from mitochondria (Liu et al., Citation2010). Sal A protects SH-SY5Y cells against H2O2-induced injury by increasing their stress tolerance via inhibition of the mitogen-activated protein kinase and Akt signaling pathways (Wang & Xu, Citation2005). Sal A also reverses 1-methyl-4-phenylpyridinium ion (an inhibitor of mitochondrial complex I)-induced cytotoxicity by decreasing ROS and Bax/Bcl-2 ratio elevation (Wang & Xu, Citation2005). Salvianic borneol ester, a compound based on the S. miltiorrhiza formulas, has been found to destabilize Aβ oligomers as well as to protect SH-SY5Y and motor neuron hybridoma cells VSC 4.1 against H2O2-induced toxicity (Han et al., Citation2011). These compounds may be effective in treating AD associated with oxidative stress.
Studies have shown that Sal B exhibits neuroprotective activity that is mediated by its antioxidative property. For example, Sal B protects PC12 cells against H2O2-induced cytotoxicity by reducing lipid peroxidation, preventing loss of antioxidant enzyme activities, inhibiting increases in intracellular [Ca2+] level and caspase-3 activity, and decreasing apoptosis (Liu et al., Citation2007). Moreover, Sal B protects neural stem cells (NSCs) derived from mouse bone marrow against H2O2 injury by reducing lactate dehydrogenase leakage and inducing brain-derived neurotrophic factor (BDNF) production (Zhang et al., Citation2012). In Aβ25–35 peptide-induced AD model mice, Sal B exhibits antioxidative and anti-inflammatory effects as well as improves memory impairment in passive avoidance tasks (Lee et al., Citation2013). These results suggest that Sal B may be a potential candidate for AD therapy.
Recently, activating NFE2 p45-related factor (Nrf2) signaling to activate enzymes against ROS has been regarded as a new neuroprotection strategy (Calabrese et al., Citation2010). The activated Nrf2 binds to the antioxidant-response element, promotes expression of detoxifying and antioxidant enzymes such as heme oxygenase-1 (HO-1), and exhibits neuroprotective effects against oxidative injury. Danshensu (β-3,4-dihydroxyphenyl-lactic acid), a water-soluble active compound isolated from Radix S. miltiorrhiza, exhibits neuronal protection in neurotoxin-treated rats (Chen et al., Citation2006). Further studies have shown that danshensu protects PC12 cells and zebrafish against 6-hydroxydopamine-induced oxidative stress by enhancing HO-1 expression through activation of the PI3K/Akt/Nrf2 signaling pathway (Chong et al., Citation2013).
Anti-apoptosis
Aβ has been shown to induce apoptosis of neurons directly or indirectly in vitro and in vivo, and apoptosis is crucial in neuronal loss in the brain of AD patients (Su et al., Citation1994; Yagami et al., Citation2002). Tanshinone IIA attenuates apoptosis induced by middle cerebral artery occlusion via decreasing caspase-3 protein expression and increasing Bcl-2 protein expression in the ischemic cortex (Chen et al., Citation2012). Tanshinone IIA also suppresses Aβ-induced apoptosis in cortical neurons by activating the Bcl-xL pathway (Qian et al., Citation2012).
In addition, Aβ25–35 decreases the expression of the brain–pancreas relative protein (BPRP) that is a protein associated with cerebral ischemia (Chen et al., Citation2003). Sal B protects PC12 cells against Aβ25–35-induced neurotoxicity by reversing BPRP reduction and suppressing accumulation of ROS, calcium influx, and apoptosis (Lin et al., Citation2006). Salvia miltiorrhiza extract protects SH-SY5Y cells against Aβ25–35-induced neurotoxicity via inhibiting oxidative stress and the mitochondria-dependent apoptotic pathway (Yu et al., Citation2014). Sal B also protects against 6-hydroxydopamine-induced apoptosis in SH-SY5Y cells by inhibiting the elevation of intracellular ROS and [Ca2+] levels, decreasing caspase-3 activity, increasing extracellular signal-regulated kinase (ERK)1/2 phosphorylation, and preventing the decrease of mitochondrial membrane potential and Bcl-2 (Tian et al., Citation2008).
Enhancing cholinergic signaling
Memory impairment is an important AD symptom that is related to a central cholinergic neuronal dysfunction (Coyle et al., Citation1983). Thus, improving cholinergic function is a target in AD therapy. Restoring cholinergic neurotransmission can be achieved by inhibiting acetylcholinesterase (AChE) or activating the postsynaptic receptors (such as GABAA receptors; Kim et al., Citation2011). AChE is the major cholinesterase in the brain that hydrolyzes the endogenous neurotransmitter ACh. AChE inhibitors, such as tacrine, donepezil, galantamine, and rivastigmine, have been employed in AD treatment. They elevate ACh levels, improve the function of central cholinergic synapses, and prevent neuronal degeneration (Ren et al., Citation2004). However, the earlier mentioned drugs can produce adverse effects such as nausea, vomiting, diarrhea, dizziness, and weight loss (Hansen et al., Citation2008), or even dose-dependent hepatotoxicity (caused by tacrine; Watkins et al., Citation1994). Therefore, novel AChE inhibitors with fewer adverse effects can be valuable alternatives in AD treatment. Diterpenoid compounds extracted from the root of S. miltiorrhiza, such as tanshinone I, tanshinone IIA, cryptotanshinone, and dihydrotanshinone, are AChE inhibitors (Ren et al., Citation2004). Tanshinone IIA and cryptotanshinone exhibit anti-amnesic activity in the Morris water maze (Liu & Wu, Citation1999; Wong et al., Citation2010). Kim et al. (Citation2007) confirmed that tanshinone congeners attenuate scopolamine (a cholinergic blockade)-induced learning and memory impairments in mouse on passive avoidance tasks partly by enhancing cholinergic signaling via inhibition of AChE and activation of GABAA/benzodiazepine receptor. Sal B also has GABAA receptor antagonistic properties. It reverses the cognitive impairments caused by scopolamine or Aβ25–35 in mouse during passive avoidance, Y-maze, and the Morris water maze tasks. This ameliorating effect is mediated partly by antagonistic GABAA receptor signaling (Kim et al., Citation2011). Moreover, these compounds have been consumed for many years without any severe adverse effects (Liu et al., Citation2001), and they can provide useful information for developing anti-amnesic drugs.
Anti-inflammation
Chronic inflammation occurs in the brain of AD patients and contributes to AD pathogenesis. AD plaques cause microglial activation, and activated microglia release excitotoxins, which can increase inflammation. Some clinical studies suggest that anti-inflammatory drugs may slow down the progress or delay the onset of AD (Akiyama et al., Citation2000). Nitric oxide (NO) overproduction is involved in neuroinflammation and contributes to AD progression. Inhibiting NO can slow down the disease thus NO has been a target in therapeutic strategies for AD (Fernandez et al., Citation2010; Togo et al., Citation2004). NO is synthesized by NO synthase (NOS) enzymes, and HO-1 regulates inducible NOS (iNOS) expression and NO production. Tanshinone IIA, Sal B, and PF2401-SF (a standardized fraction of S. miltiorrhiza) suppress iNOS expression and NO production via increasing HO-1 expression and exhibit anti-inflammatory effects on lipopolysaccharide (LPS)-activated RAW 264.7 macrophages (Chen et al., Citation2007; Jiang et al., Citation2013; Joe et al., Citation2012). Further studies show that tanshinone IIA and cryptotanshinone inhibit LPS-induced NO production in RAW 264.7 macrophages by inhibiting NF-κB and activating the ERK signaling pathway (Choi et al., Citation2004). Tanshinone IIA inhibits iNOS and inflammatory cytokines (IL-1β, IL-6, and TNF-α) expression via activation of estrogen receptor in LPS-activated RAW 264.7 cells (Fan et al., Citation2009). Moreover, cryptotanshinone suppresses inflammation by inhibiting cyclooxygenase-2 enzymatic activity, and consequently reducing prostaglandin E2 (an important inflammation mediator) synthesis in LPS and phorbolmyristate acetate-stimulated human U937 promonocytes (Jin et al., Citation2006). The anti-inflammatory activities of danshen extracts would likely help in slowing the progression or delaying the onset of AD.
Peripheral macrophages remove Aβ in vivo by phagocytosis, which can provide a novel strategy to treat AD (Mildner et al., Citation2011; Wisniewski et al., Citation1991). Danshen prevents increases in intracellular ROS by inducing HO-1 expression via the PI3K/Akt, MEK1, and Nrf2 signaling pathways; it also exhibits cytoprotective activity in RAW 264.7 macrophages (Lee et al., Citation2012).
Neurogenesis-inducing activities of S. miltiorrhiza
Neural cell loss, learning, and memory disability are the main characteristics of AD. Neurons are terminally differentiated cells and cannot regenerate after injury. To date, no effective AD treatment has yet been reported. Recently, significant interest has been given to NSC therapy of AD because neurogenesis continues in the adult brain. Neural progenitor cells in the subventricular zone (SVZ) and the dentate gyrus of the hippocampus can proliferate and differentiate into neurons, astrocytes, and oligodendrocytes (Eriksson et al., Citation1998). NSC therapy replaces damaged neuronal cells by mobilizing endogenous stem cells or transplanting exogenous stem cells (Limke & Rao, Citation2002), thus providing a promising approach to treat AD. Neurogenesis in the adult brain is insufficient to stop neural loss. Therefore, regulating NSC proliferation and differentiation into functional neurons is an important factor in using NSCs to treat AD. Recent research has indicated that Sal B increases proliferation of mouse embryonic cortex NSCs by accumulating G2/S phase cells, as well as promotes neurite outgrowth and neural differentiation of mouse cortex NSCs (Guo et al., Citation2010). Sal B also promotes proliferation of rat embryonic cortex NSCs in a dose- and time-dependent manner by activating the PI3K/Akt signaling pathway. The Morris water maze test shows that post-ischemic treatment with Sal B improves cognitive impairment after stroke in rats (Zhuang et al., Citation2012). These results suggest that Sal B is a potential candidate in mobilizing endogenous stem cells to treat neurodegenerative diseases.
For transplanting exogenous stem cells, bone marrow is a potent resource to produce NSCs. NSCs derived from bone marrow cells (BM-NSCs) exhibit morphological properties and differentiation ability comparable with those of NSCs derived from the SVZ (Yang et al., Citation2010). However, the rate of stem cell survival and differentiation after transplantation to central neural system is poor (Mimura et al., Citation2005), and antileptics that could enhance neural differentiation are required. Fortunately, Sal B promotes BM-NSCs proliferation and neural differentiation, as well as induces BDNF production by BM-NSCs. BDNF is beneficial to cell survival and differentiation in unfavorable environments (Zhang et al., Citation2012). Hu et al. (Citation2011) found that S. miltiorrhiza efficiently induces differentiation of rat mesenchymal stem cells (MSCs) into neurons with neurophysiological functions, including action potentials, endocytosis, and exocytosis in response to high potassium concentration. Moreover, Sal B provides neuroprotection to spinal cord injury and improves the survival of MSCs in vitro and in vivo (Bi et al., Citation2008). Thus, transplanting MSCs induced with S. miltiorrhiza may be an effective way in the treatment of neurodegenerative diseases.
Induced pluripotent stem cells (iPSCs) have the potential of differentiation into neural lineages, and thus have been regarded as a promising source for treating nervous system disorders (Almeida et al., Citation2013). Salvia miltiorrhiza enhances the differentiation of mouse iPSCs into NSCs and neurons in vitro, and promotes the survival and neural differentiation of transplanted iPSCs-derived NSCs in vivo, as well as improves function recovery after their transplantation to the rat ischemic brain tissues (Shu et al., Citation2014). It is worth noting that 1 or 5 µg/ml S. miltiorrhiza simulates the neurogenesis from iPSCs, while 10 µg/ml S. miltiorrhiza has no significant effects on it. It indicates that neurogensis-inducing effects of S. Miltiorrhiza have an optimal range of concentrations (Shu et al., Citation2014). It may be also related to the different effects of S. Miltiorrhiza active components. For example, Ren et al. (Citation2013) reported that hydrophilic and lipophilic components of S. Miltiorrhiza had opposite effects on human umbilical vein endothelial cells (HUVECs): the former promoted and the latter inhibited the proliferation and the migration of HUVECs. Our results show that cryptotanshinone inhibits the proliferation of human embryonic stem cells-derived neural stem cells in vitro because it is an inhibitor of Stat3 (unpublished data).
Conclusion
AD is a complex neurodegenerative disorder that involves multiple pathogenetic factors; “multitarget-directed ligand” design strategy (i.e., one agent modulates multiple targets simultaneously) provides new methods to treat AD (Bolognesi et al., Citation2009; Ji & Zhang, Citation2008). Salvia miltiorrhiza and its active components, such as cryptotanshinone, tanshinone I, tanshinone IIA, Sal B, Sal A, and danshensu, exhibit multiple neuroprotective effects. In addition, S. miltiorrhiza and Sal B exhibit neurogenesis-inducing activities. These components have been used in clinical practice as drugs with efficient blood–brain barrier penetration (Ren et al., Citation2004; Yu et al., Citation2011), and thus, may be effective in preventing and treating AD. Moreover, more anti-AD drugs are expected to be developed according to the structures and actions of S. miltiorrhiza components.
Declaration of interest
The authors report that they have no conflicts of interest. This work was supported by grants from National Natural Science Foundation of China (No. 31100987), a project of Shandong Province Higher Educational Science and Technology Program (J12LE03), and Shandong University of Technology projects for the development of young teachers.
References
- Akiyama H, Barger S, Barnum S, et al. (2000). Inflammation and Alzheimer’s disease. Neurobiol Aging 21:383–421
- Almeida S, Gascon E, Tran H, et al. (2013). Modeling key pathological features of frontotemporal dementia with C9ORF72 repeat expansion in iPSC derived human neurons. Acta Neuropathol 126:385–99
- Bi XB, Deng YB, Gan DH, Wang YZ. (2008). Salvianolic acid B promotes survival of transplanted mesenchymal stem cells in spinal cord-injured rats. Acta Pharmacol Sin 29:169–76
- Bolognesi ML, Matera R, Minarini A, et al. (2009). Alzheimer’s disease: New approaches to drug discovery. Curr Opin Chem Biol 13:303–8
- Butterfield DA. (2002). Amyloid beta-peptide (1-42)-induced oxidative stress and neurotoxicity: Implications for neurodegeneration in Alzheimer’s disease brain: A review. Free Radic Res 36:1307–13
- Butterfield DA, Reed T, Newman SF, Sultana R. (2007). Roles of amyloid beta-peptide-associated oxidative stress and brain protein modifications in the pathogenesis of Alzheimer’s disease and mild cognitive impairment. Free Radic Biol Med 43:658–77
- Calabrese V, Cornelius C, Dinkova-Kostova AT, et al. (2010). Cellular stress responses, the hormesis paradigm, and vitagenes: Novel targets for therapeutic intervention in neurodegenerative disorders. Antioxid Redox Signal 13:1763–811
- Cao YY, Wang L, Ge H, et al. (2013). Salvianolic acid A, a polyphenolic derivative from Salvia miltiorrhiza Bunge, as a multifunctional agent for the treatment of Alzheimer’s disease. Mol Divers 17:515–24
- Chen J, Wang F, Lee FS, et al. (2006). Separation and identification of water-soluble salvianolic acids from Salvia miltiorrhiza Bunge by high-speed counter-current chromatography and ESI-MS analysis. Talanta 69:172–9
- Chen JX, Wang SR, Zhao MJ, et al. (2003). Effect of different drug dosage to activate blood circulation and to nourish qi on cardiac function and structure of congestive heart failure rats after acute myocardial infarction. Zhongguo Zhong Yao Za Zhi 28:446–9
- Chen TH, Hsu YT, Chen CH, et al. (2007). Tanshinone IIA from Salvia miltiorrhiza induces heme oxygenase-1 expression and inhibits lipopolysaccharide-induced nitric oxide expression in RAW 264.7 cells. Mitochondrion 7:101–5
- Chen Y, Wu X, Yu S, et al. (2012). Neuroprotective capabilities of Tanshinone IIA against cerebral ischemia/reperfusion injury via anti-apoptotic pathway in rats. Biol Pharm Bull 35:164–70
- Choi HS, Cho DI, Choi HK, et al. (2004). Molecular mechanisms of inhibitory activities of tanshinones on lipopolysaccharide-induced nitric oxide generation in RAW 264.7 cells. Arch Pharm Res 27:1233–7
- Chong CM, Zhou ZY, Razmovski-Naumovski V, et al. (2013). Danshensu protects against 6-hydroxydopamine-induced damage of PC12 cells in vitro and dopaminergic neurons in zebrafish. Neurosci Lett 543:121–5
- Coyle JT, Price DL, DeLong MR. (1983). Alzheimer’s disease: A disorder of cortical cholinergic innervation. Science 219:1184–90
- Cummings JL. (2004). Alzheimer’s disease. N Engl J Med 351:56–67
- Durairajan SS, Yuan Q, Xie L, et al. (2008). Salvianolic acid B inhibits Abeta fibril formation and disaggregates preformed fibrils and protects against Abeta-induced cytotoxicty. Neurochem Int 52:741–50
- Eriksson PS, Perfilieva E, Bjork-Eriksson T, et al. (1998). Neurogenesis in the adult human hippocampus. Nat Med 4:1313–17
- Fan GW, Gao XM, Wang H, et al. (2009). The anti-inflammatory activities of Tanshinone IIA, an active component of TCM, are mediated by estrogen receptor activation and inhibition of iNOS. J Steroid Biochem Mol Biol 113:275–80
- Fernandez AP, Pozo-Rodrigalvarez A, Serrano J, Martinez-Murillo R. (2010). Nitric oxide: Target for therapeutic strategies in Alzheimer’s disease. Curr Pharm 16:2837–50
- Guo G, Li B, Wang Y, et al. (2010). Effects of salvianolic acid B on proliferation, neurite outgrowth and differentiation of neural stem cells derived from the cerebral cortex of embryonic mice. Sci China Life Sci 53:653–62
- Haass C. (2004). Take five – BACE and the gamma-secretase quartet conduct Alzheimer’s amyloid beta-peptide generation. EMBO J 23:483–8
- Han M, Liu Y, Zhang B, et al. (2011). Salvianic borneol ester reduces beta-amyloid oligomers and prevents cytotoxicity. Pharm Biol 49:1008–13
- Hansen RA, Gartlehner G, Webb AP, et al. (2008). Efficacy and safety of donepezil, galantamine, and rivastigmine for the treatment of Alzheimer’s disease: A systematic review and meta-analysis. Clin Interv Aging 3:211–25
- Hardy J, Selkoe DJ. (2002). The amyloid hypothesis of Alzheimer’s disease: Progress and problems on the road to therapeutics. Science 297:353–6
- Helmuth L. New therapies. (2002). New Alzheimer’s treatments that may ease the mind. Science 297:1260–2
- Hu L, Yu J, Li F, et al. (2011). Effects of Salvia miltorrhiza in neural differentiation of rat mesenchymal stem cells with optimized protocol. J Ethnopharmacol 136:334–40
- Huebbe P, Schaffer S, Jofre-Monseny L, et al. (2007). Apolipoprotein E genotype and alpha-tocopherol modulate amyloid precursor protein metabolism and cell cycle regulation. Mol Nutr Food Res 51:1510–17
- Ji HF, Zhang HY. (2008). Multipotent natural agents to combat Alzheimer’s disease. Functional spectrum and structural features. Acta Pharmacol Sin 29:143–51
- Jiang WY, Jeon BH, Kim YC, et al. (2013). PF2401-SF, standardized fraction of Salvia miltiorrhiza shows anti-inflammatory activity in macrophages and acute arthritis in vivo. Int Immunopharmacol 16:160–4
- Jin DZ, Yin LL, Ji XQ, Zhu XZ. (2006). Cryptotanshinone inhibits cyclooxygenase-2 enzyme activity but not its expression. Eur J Pharmacol 549:166–72
- Joe Y, Zheng M, Kim HJ, et al. (2012). Salvianolic acid B exerts vasoprotective effects through the modulation of heme oxygenase-1 and arginase activities. J Pharmacol Exp Ther 341:850–8
- Kim D, Tsai LH. (2009). Bridging physiology and pathology in AD. Cell 137:997–1000
- Kim DH, Jeon SJ, Jung JW, et al. (2007). Tanshinone congeners improve memory impairments induced by scopolamine on passive avoidance tasks in mice. Eur J Pharmacol 574:140–7
- Kim DH, Park SJ, Kim JM, et al. (2011). Cognitive dysfunctions induced by a cholinergic blockade and Abeta 25-35 peptide are attenuated by salvianolic acid B. Neuropharmacology 61:1432–40
- Lee MS, Kwon YT, Li M, et al. (2000). Neurotoxicity induces cleavage of p35 to p25 by calpain. Nature 405:360–4
- Lee SE, Jeong SI, Yang H, et al. (2012). Extract of Salvia miltiorrhiza (Danshen) induces Nrf2-mediated heme oxygenase-1 expression as a cytoprotective action in RAW 264.7 macrophages. J Ethnopharmacol 139:541–8
- Lee YW, Kim DH, Jeon SJ, et al. (2013). Neuroprotective effects of salvianolic acid B on an Aβ25–35 peptide-induced mouse model of Alzheimer’s disease. Eur J Pharmacol 704:70–7
- Limke TL, Rao MS. (2002). Neural stem cells in aging and disease. J Cell Mol Med 6:475–96
- Lin YH, Liu AH, Wu H, et al. (2006). Salvianolic acid B, an antioxidant from Salvia miltiorrhiza, prevents Abeta(25-35)-induced reduction in BPRP in PC12 cells. Biochem Biophys Res Commun 348:593–9
- Liu CS, Chen NH, Zhang JT. (2007). Protection of PC12 cells from hydrogen peroxide-induced cytotoxicity by salvianolic acid B, a new compound isolated from Radix Salviae miltiorrhizae. Phytomedicine 14:492–7
- Liu J, Yang CF, Wasser S, et al. (2001). Protection of Salvia miltiorrhiza against aflatoxin-B1-induced hepatocarcinogenesis in Fischer 344 rats dual mechanisms involved. Life Sci 69:309–26
- Liu T, Jin H, Sun QR, et al. (2010). The neuroprotective effects of tanshinone IIA on beta-amyloid-induced toxicity in rat cortical neurons. Neuropharmacology 59:595–604
- Liu XJ, Wu WT. (1999). Effects of ligustrazine, tanshinone II A, ubiquinone, and idebenone on mouse water maze performance. Zhongguo Yao Li Xue Bao 20:987–90
- Mattson MP. (1997). Cellular actions of beta-amyloid precursor protein and its soluble and fibrillogenic derivatives. Physiol Rev 77:81–132
- Mei Z, Situ B, Tan X, et al. (2010). Cryptotanshinione upregulates alpha-secretase by activation PI3K pathway in cortical neurons. Brain Res 1348:165–73
- Mei Z, Yan P, Situ B, et al. (2012). Cryptotanshinione inhibits beta-amyloid aggregation and protects damage from beta-amyloid in SH-SY5Y cells. Neurochem Res 37:622–8
- Mei Z, Zhang F, Tao L, et al. (2009). Cryptotanshinone, a compound from Salvia miltiorrhiza modulates amyloid precursor protein metabolism and attenuates beta-amyloid deposition through upregulating alpha-secretase in vivo and in vitro. Neurosci Lett 452:90–5
- Mildner A, Schlevogt B, Kierdorf K, et al. (2011). Distinct and non-redundant roles of microglia and myeloid subsets in mouse models of Alzheimer’s disease. J Neurosci 31:11159–71
- Mimura T, Dezawa M, Kanno H, Yamamoto I. (2005). Behavioral and histological evaluation of a focal cerebral infarction rat model transplanted with neurons induced from bone marrow stromal cells. J Neuropathol Exp Neurol 64:1108–17
- Mott RT, Hulette CM. (2005). Neuropathology of Alzheimer’s disease. Neuroimaging Clin N Am 15:755–65, ix
- Qian YH, Xiao Q, Xu J. (2012). The protective effects of tanshinone IIA on beta-amyloid protein (1-42)-induced cytotoxicity via activation of the Bcl-xL pathway in neuron. Brain Res Bull 88:354–8
- Radak Z, Zhao Z, Goto S, Koltai E. (2011). Age-associated neurodegeneration and oxidative damage to lipids, proteins and DNA. Mol Aspects Med 32:305–15
- Ren G, Fan X, Liang Q, et al. (2013). Screening and evaluation of traditional Chinese medicine by microarray expression analysis. J Ethnopharmacol 147:564–9
- Ren Y, Houghton PJ, Hider RC, Howes J. (2004). Novel diterpenoid acetylcholinesterase inhibitors from Salvia miltiorhiza. Planta Med 70:201–4
- Shi LL, Yang WN, Chen XL, et al. (2012). The protective effects of tanshinone IIA on neurotoxicity induced by beta-amyloid protein through calpain and the p35/Cdk5 pathway in primary cortical neurons. Neurochem Int 61:227–35
- Shu T, Pang M, Rong L, et al. (2014). Effects of Salvia miltiorrhiza on neural differentiation of induced pluripotent stem cells. J Ethnopharmacol 153:233–41
- Su JH, Anderson AJ, Cummings BJ, Cotman CW. (1994). Immunohistochemical evidence for apoptosis in Alzheimer’s disease. Neuroreport 5:2529–33
- Taylor CJ, Ireland DR, Ballagh I, et al. (2008). Endogenous secreted amyloid precursor protein-alpha regulates hippocampal NMDA receptor function, long-term potentiation and spatial memory. Neurobiol Dis 31:250–60
- Tian LL, Wang XJ, Sun YN, et al. (2008). Salvianolic acid B, an antioxidant from Salvia miltiorrhiza, prevents 6-hydroxydopamine induced apoptosis in SH-SY5Y cells. Int J Biochem Cell Biol 40:409–22
- Togo T, Katsuse O, Iseki E. (2004). Nitric oxide pathways in Alzheimer’s disease and other neurodegenerative dementias. Neurol Res 26:563–6
- Yagami T, Ueda K, Asakura K, et al. (2002). Gas6 rescues cortical neurons from amyloid protein-induced apoptosis. Neuropharmacology 43:1289–96
- Yang J, Yan Y, Ciric B, et al. (2010). Evaluation of bone marrow-and brain-derived neural stem cells in therapy of central nervous system autoimmunity. Am J Pathol 177:1989–2001
- Yu H, Yao L, Zhou H, et al. (2014). Neuroprotection against Aβ25-35-induced apoptosis by Salvia miltiorrhiza extract in SH-SY5Y cells. Neurochem Int 75:89–95
- Yu PF, Wang WY, Eerdun G, et al. (2011). The role of P-glycoprotein in transport of danshensu across the blood-brain barrier. Evid Based Complement Alternat Med 2011:713523
- Wang Q, Yu X, Patal K, et al. (2013). Tanshinones inhibit amyloid aggregation by amyloid-beta peptide, disaggregate amyloid fibrils, and protect cultured cells. ACS Chem Neurosci 4:1004–15
- Wang X, Morris-Natschke SL, Lee KH. (2007). New developments in the chemistry and biology of the bioactive constituents of Tanshen. Med Res Rev 27:133–48
- Wang XJ, Xu JX. (2005). Salvianic acid A protects human neuroblastoma SH-SY5Y cells against MPP+-induced cytotoxicity. Neurosci Res 51:129–38
- Watkins PB, Zimmerman HJ, Knapp MJ, et al. (1994). Hepatotoxic effects of tacrine administration in patients with Alzheimer’s disease. JAMA 271:992–8
- Whitehouse PJ, Price DL, Clark AW, et al. (1981). Alzheimer disease: Evidence for selective loss of cholinergic neurons in the nucleus basalis. Ann Neurol 10:122–6
- Wisniewski HM, Barcikowska M, Kida E. (1991). Phagocytosis of beta/A4 amyloid fibrils of the neuritic neocortical plaques. Acta Neuropathol 81:588–90
- Wong KK, Ho MT, Lin HQ, et al. (2010). Cryptotanshinone, an acetylcholinesterase inhibitor from Salvia miltiorrhiza, ameliorates scopolamine-induced amnesia in Morris water maze task. Planta Med 76:228–34
- Zhang F, Zheng W, Pi R, et al. (2009). Cryptotanshinone protects primary rat cortical neurons from glutamate-induced neurotoxicity via the activation of the phosphatidylinositol 3-kinase/Akt signaling pathway. Exp Brain Res 193:109–18
- Zhang N, Kang T, Xia Y, et al. (2012). Effects of salvianolic acid B on survival, self-renewal and neuronal differentiation of bone marrow derived neural stem cells. Eur J Pharmacol 697:32–9
- Zhong GX, Li P, Zeng LJ, et al. (2009). Chemical characteristics of Salvia miltiorrhiza (Danshen) collected from different locations in China. J Agric Food Chem 57:6879–87
- Zhou L, Zuo Z, Chow MS. (2005). Danshen: An overview of its chemistry, pharmacology, pharmacokinetics, and clinical use. J Clin Pharmacol 45:1345–59
- Zhuang P, Zhang Y, Cui G, et al. (2012). Direct stimulation of adult neural stem/progenitor cells in vitro and neurogenesis in vivo by salvianolic acid B. PLoS One 7:e35636