Abstract
Context: The use of many traditional medicinal plants is often hampered by the absence of a proper biochemical characterization, which is essential to identify the bioactive compounds present in it. The essential oils (EOs) of three native species from the La Rioja province were analyzed: Lippia turbinata Griseb and Lippia integrifolia (Griseb.) Hieron (Verbenaceae), and Clinopodium gilliesii (Benth.) Kuntze (Lamiaceae).
Objective: The aim of this study was to evaluate their EOs antioxidant activity (AA) and their chemical composition.
Materials and methods: EOs were analyzed by gas chromatography-mass spectrometry (GC-MS). To enhance the aqueous solubilization of the EOs, EO–water emulsions were prepared (concentration range of 0.1–6 mg mL−1). AA was determined using ABTS, DPPH, and peroxyl radical scavenging assays, as well as by the β-carotene bleaching test.
Results: Piperitenone oxide was a major constituent in L. turbinata, pulegone and piperitenone oxide in C. gilliesii, and β-caryophyllene in L. integrifolia. Lippia turbinata EO was the most active ABTS and DPPH radical scavenger (SC50 values of 0.40 ± 0.14 and 0.74 ± 0.08 mg mL−1, respectively). Clinopodium gilliesii EO exhibited the highest hydrogen peroxide scavenging activity (SC25 value = 1.52 ± 0.27 mg mL−1). In the β-carotene assay, L. turbinata EO was more effective at inhibiting lipid peroxidation than the other two oils (IC25 value = 0.15 ± 0.04 mg mL−1).
Conclusion: Our results suggest that the AA observed can be justified by the presence of oxygenated monoterpenes, mainly piperitenone oxide. Finally, L. turbinata EO might be used as a safe natural antioxidant and food preservative in the food and cosmetic industries.
Introduction
In animal tissues, oxidants can cause cellular damage through the peroxidation of unsaturated fatty acids, denaturation of proteins, and reactions with nucleic acids and carbohydrates. These damages have been involved in the pathogenesis of several human disorders such as cancer, neurodegenerative disorders, rheumatoid arthritis, and ageing (Pham-Huy et al., Citation2008). In this sense, natural products with antioxidant properties could have great importance as therapeutic agents in a several diseases, since they are effective as radical scavengers and inhibitors of lipid peroxidation (Sen et al., Citation2010).
In recent years, due to toxicological concerns associated with the use of synthetic substances in food, and increasing awareness about natural foods, there has been a growing interest in the use of natural substances as food preservatives and antioxidants (Peschel et al., Citation2006). In this context, many aromatic plants, and particularly their essential oils (EOs), are being evaluated for antioxidant activity (AA) (Martins et al., Citation2014; Mohamed et al., Citation2013; Quiroga et al., Citation2011).
Lippia turbinata, Clinopodium gilliesii, and Lippia integrifolia are three popular aromatic species used widely in many Argentinean regions. In this sense, the province of La Rioja has a rich folk medicine tradition using an ample variety of medicinal and aromatic plants.
Lippia turbinata Griseb (Verbenaceae) or “poleo” is a native shrub from South America, commonly found in the west-central region of Argentina. It is well known for its aromatic-medicinal properties such as its digestive and antispasmodic effects to treat gastrointestinal disorders. It is also used in dyspepsia, oliguria, and dysmenorrhea (Alonso & Desmarchelier, Citation2006; Pascual et al., Citation2001).
Clinopodium gilliesii (Benth.) Kuntze (Lamiaceae) known popularly as “muña-muña” is a native herb which grows in the provinces of Salta, Jujuy, Córdoba, Catamarca, Tucumán, La Rioja, San Luis, and San Juan. It is used in traditional medicine to treat gastric troubles, stomach aches, and female sterility; furthermore, it is also known as an aphrodisiac (Bustos et al., Citation1996; Viturro et al., Citation2000).
Lippia integrifolia (Griseb.) Hieron (Verbenaceae) is a shrub, known popularly as “incayuyo”, which grows in the Northwestern region of Argentina. It is traditionally used to treat dyspepsia, indigestions, and stomachaches, as a diuretic, emmenagogue, and tonic agent (Alonso & Desmarchelier, Citation2006).
The biological activity of these plants has been investigated by several researchers. Although most research has been done with extracts, a few studies have assessed the activity of their EOs. The antibacterial (González & Marioli, Citation2010), antifungal (Bluma & Etcheverry, Citation2008; Passone & Etcheverry, Citation2014), insecticidal (Gleiser & Zygadlo, Citation2007), virucidal (García et al., Citation2003), and antioxidant (Quiroga et al., 2013) activities of L. turbinata EO have been reported, as well as the antifungal, antibacterial (Lima et al., Citation2011), and antiradical (Cabana et al., Citation2013) properties of C. gilliesii, and the antibacterial, antifungal (Lima et al., Citation2011), and insect-repellent (Gleiser et al., Citation2011; Lima et al., Citation2011) activities of L. integrifolia EO.
In the present study, we compared the AA between the EOs extracted from L. turbinata, C. gilliesii, and L. integrifolia, and also analyzed their chemical composition. To our knowledge, this is the first report on both the chemical composition and the antioxidant potential of EOs from L. turbinata, C. gilliesii, and L. integrifolia collected in the La Rioja province (Valle Antinaco-Los Colorados).
Materials and methods
Plant materials and isolation of EOs
The plant specimens, L. turbinata, C. gilliesii, and L. integrifolia, were collected in February 2013 from Valle Antinaco-Los Colorados, province of La Rioja, Argentina (1100–1620 m.a.s.l). Sample copies are kept in the personal collection of the team in the Universidad Nacional de Chilecito. The plants were botanically identified by Lic. Cs. Biol. Gloria S Jamie (Universidad Nacional de Tucumán, Tucumán, Argentina) according to the reference material present in Miguel Lillo Institute Herbarium, Tucumán, Argentina (LIL) and specific literature. The following are the voucher data of Cfr (examined reference material) with studied material: L. turbinata Griseb. Cfr L. turbinata Griseb. Prov. La Rioja LIL 27589, L. integrifolia (Griseb.) Hieron. Cfr L. integrifolia (Griseb.) Hieron. Prov. La Rioja LILL 609723, and C. gilliesii (Benth.) Kuntze Cfr Syn. Satureja parvifolia (Phil.) Mold. Prov. La Rioja LILL 420243.
The air-dried aerial parts of plant samples were submitted to hydrodistillation for 3 h using a Clevenger apparatus. The EOs obtained were stored at 4 °C for subsequent experiments. Yields of the samples were 1.0% for L. turbinata, 1.2% for C. gilliesii and 1.4% for L. integrifolia.
Analysis of EO compounds
The chemical composition of the EOs was analyzed using a gas chromatography-mass spectrometry (GC-MS) carried out on a Shimadzu GC-2010 GCMS-QP2010 (Shimadzu Corporation, Kyoto, Japan) fitted with a DB 5-fused silica column (30 m × 0.25 mm i.d., a film thickness of 0.25 μm). The initial oven temperature was set at 60 °C, then it was increased to 250 °C at 4 °C min−1, and the final temperature was maintained for 5 min. Carrier gas, helium, was adjusted to a linear velocity of 34 cm s−1 (1 mL min−1). The injector temperature was 250 °C, the injection volume of 1 μL, and the split ratio of 1:10. The identity of the oil components was established by comparison of their MS spectra with those reported in Wiley8 and Nist08 libraries and the relative percentage of the individual compounds in each EO was calculated using the total ion current from the MS detector signal.
Preparation of EO emulsions
To enhance the aqueous solubilization of the EOs, stock EO–water emulsions were prepared in two concentrations: 4 mg mL−1 and 10 mg mL−1 by weighing 20 and 50 mg of each oil and adding them to 5 mL of distilled water. The tubes were then shaken using a 40 kHz ultrasonic TESTLAB-BU-01 (Shimadzu Corporation, Kyoto, Japan) for 30 min. The emulsions were diluted and used in a concentration range of 0.1–6 mg mL−1.
DPPH and ABTS radical scavenging assay
DPPH radical scavenging activity was measured as described by Farasat et al. (Citation2013) with minor modifications. Briefly, 95 µL of DPPH solution was added to 105 µL of each EO aliquot previously dissolved in ethanol over a concentration range of 0.1–8.5 mg mL−1. The absorbance of the reaction mixture at 520 nm was measured using a microplate reader (Biotek EL 808, BioTek Instruments, Inc., Shoreline, WA). The SC50 values (concentration of sample required to scavenge 50% DPPH radicals) were calculated using the regression equation prepared from the different EOs concentrations.
The radical scavenging capacity of the samples for the ABTS radical cation was determined as described by Re et al. (Citation1999) with some modifications. About 50 µL of ABTS solution was added to 150 µL of EO emulsions (a concentration range of 0.1–5 mg mL−1). The absorbance was measured at 734 nm (microplate reader Biotek EL 808, BioTek Instruments, Inc., Shoreline, WA) 6 min after initial mixing. Results were expressed as SC50 values (sample concentration required to scavenge 50% ABTS radicals).
Hydrogen peroxide scavenging assay
The ability of the EOs to scavenge hydrogen peroxide was determined according to the method of Ruch et al. (Citation1989). About 100 μL of each EO emulsion (0.5–6 mg mL−1) were added to the reaction mixture containing 900 μL of 2 mM hydrogen peroxide. After 10 min, the absorbance was read against a blank at 230 nm (Spectrophotometer Shimadzu UV-240PC, Shimadzu Corporation, Kyoto, Japan). The SC50 values (concentration that is required to scavenge 50% H2O2 radicals) were calculated.
β-Carotene–linoleic acid assay
AA based on the β-carotene bleaching test was evaluated by measuring the inhibition of the bleaching of β-carotene by the peroxides generated during linoleic acid oxidation (Ordoñez et al., Citation2006). A stock solution of β-carotene–linoleic acid mixture was prepared adding 1 mg β-carotene, 1 mL chloroform, 20 µL linoleic acid, and 200 µL Tween 40. Chloroform was completely evaporated and 40 mL of oxygenated distilled water was added to form a yellowish emulsion. About 100 μL of the EO in methanol solution (0.05–1.8 mg mL−1) was added to 1 mL of β-carotene–linoleic acid stock solution. The absorbance was measured at 470 nm (Spectrophotometer Shimadzu UV-240PC, Shimadzu Corporation, Kyoto, Japan) at 0 min, and after 30, 60, 90, and 120 min incubation in a water bath at 50 °C. IC50 values (concentration required to inhibit 50% β-carotene bleaching) were determined from graphs with AA plotted against EO concentration.
Statistical analysis
The data are presented as mean ± standard deviation of three determinations. Statistical significance was analyzed by the one-way analysis of variance followed by the Tukey test (MINITAB software version 15 for Windows, SPSS Inc., Chicago, IL). p Values < 0.05 were regarded as significant.
Results and discussion
Chemical composition of the EOs
Since the biological activity of EOs is dictated by the sum of its components, we first analyzed their chemical composition. The compositions of L. turbinata, C. gilliesii, and L. integrifollia oils were determined by comparing mass spectra of oil components with those from Wiley and NIST08 libraries, plus two other criteria: retention index (Kovat’s Index) and comparison with literature data (Adams, Citation2001). Volatile oils are very complex compound mixtures in which a variety of mono- and sesquiterpenes can be found. Ten compounds were identified in the EO from L. turbinata, 12 from C. gilliesii and 13 from L. integrifolia, representing 86.6, 78.0, and 68.4% of each total oil, respectively (). The major compounds identified by GC-MS in the L. turbinata EO were piperitenone oxide (63.0%) and limonene (7.2%). While the major components in C. gilliesii EO were pulegone (19.2%), piperitenone oxide (14.7%), isopulegone (12.0%), and menthone (9.7%). GC-MS analysis of L. integrifolia EO showed that β-caryophyllene (15.3%) and terpinen-4-ol (8.7%) were its major constituents ().
Table 1. Main components of the essential oils from L. turbinata, C. gilliesii, and L. integrifolia.
Monoterpenes were dominant in the L. turbinata and C. gilliesii EOs in contrast to the L. integrifolia oil, in which sesquiterpenes were the most abundant constituents. As shown in , monoterpenes (and their derivatives) represent 78.6% of L. turbinata EO, 71.4% being oxygenated monoterpenoids. In C. gilliesii, monoterpenes and monoterpenoids represent 74.5% of the overall EO, 74.0% being oxygenated monoterpenoids. About 43.1% of L. integrifolia EO was identified as sesquiterpenes (or their derivatives), 23.5% being hydrocarbon sesquiterpenes, and 19.6% oxygenated sesquiterpenoids.
Antioxidant activity of the EOs
EOs, as natural sources of antioxidants, have been evaluated for their activity as free radical (FR) scavengers. ABTS and DPPH are stable FR, which have been widely accepted as a tool for estimating FR scavenging activities of antioxidants (Miguel, Citation2010). The percentages of remaining ABTS and DPPH in the presence of all oils at different concentrations are shown in . ABTS and DPPH scavenging activities of all the tested samples were observed to be dose dependent. The SC50 values indicated that L. turbinata EO had the highest AA in ABTS and DPPH tests. In addition, C. gilliesii EO showed a stronger AA than L. integrifolia EO in these tests ().
Figure 1. Free radical scavenging activity of essential oils from L. turbinata, C. gilliesii, and L. integrifolia. The activity was measured by the scavenging of ABTS (A) and DPPH (B) radicals. Values are means ± SD, n = 3.
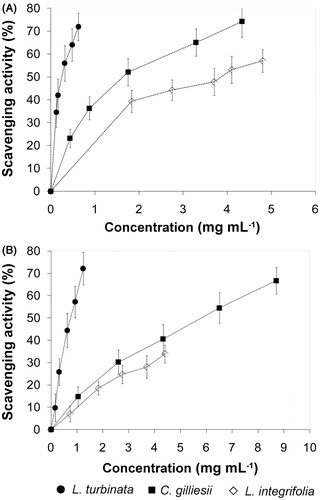
Table 2. Antioxidant activity of L. turbinata, C. gilliesii, and L. integrifolia essential oils.
The SC50 values obtained with the ABTS test were lower than those with the DPPH test (). The differences between the two results can be explained by the mechanism of the reaction involved. The ABTS radical reaction involves electron transfer and takes place at a much faster rate compared with DPPH radicals whose degree of discoloration is attributed to the hydrogen-donating ability of tested compounds (Afoulous et al., Citation2013; Bayala et al., Citation2014).
The most significant FR in biological systems results from oxygen. In this sense, we evaluated the effect of EOs on ROS using the hydrogen peroxide scavenging test. Hydrogen peroxide is not reactive on its own, but, under appropriate conditions, it can form hydroxyl radicals. These radicals are the most reactive oxygen radical types as they can rapidly react with polyunsaturated fatty acids, resulting in the production of peroxyl radicals (Miguel, Citation2010; Sahin & Candan, Citation2013). When the EO hydrogen peroxide inhibition activity was measured, it was observed that C. gilliesii EO was more active than L. integrifolia EO, and the AA of L. turbinata EO was intermediate between them ().
The β-carotene–linoleic acid test is usually used to estimate the potential ability of the antioxidants to delay lipid peroxidation by reacting with the peroxyl radical propagating chains faster than the reaction of these radicals with proteins or fatty acid side chains (Ebrahimabadi et al., Citation2010). The inhibition of β-carotene oxidation in the presence of different EOs is shown in . Considering the IC25 or IC50 values, the activity of L. turbinata EO was higher than that of both C. gilliesii and L. integrifolia oils (). The IC25 values shown also demonstrate that C. gilliesii EO was more effective at inhibiting lipid peroxidation than L. integrifolia EO ().
Figure 2. β-Carotene bleaching assay of L. turbinata, C. gilliesii, and L. integrifolia essential oils. The β-carotene oxidation induced by heat was monitored for 120 min. The maximum concentrations used were 1.13 mg mL−1 for L. turbinata, 1.79 mg mL−1 for C. gilliesii, and 1.38 mg mL−1 for L. Integrifolia.
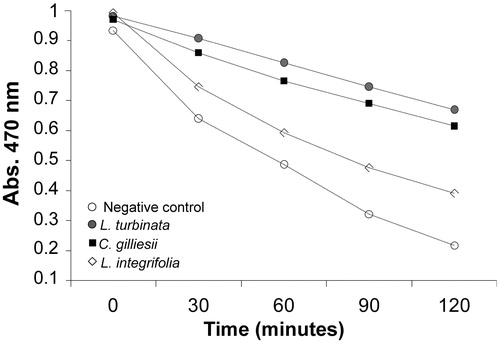
Antioxidant activity versus phytochemical composition
The results obtained revealed that L. turbinata EO is the best antioxidant agent as compared with the other two oils, due to its high ability to scavenge FR and ROS as well as its capacity of inhibiting lipid peroxidation. Additionally, the AA of C. gilliesii oil was higher than that of L. integrifolia.
The major compounds of L. turbinata and C. gilliesii oils are oxygenated monoterpenes while the major compounds of L. integrifolia EO are sesquiterpenes, which could explain the differences in the AA between them. In addition, the oxygenated monoterpenes found in these EOs may act as antioxidant agents.
The antioxidant properties of monoterpene in EOs have been referred to by several authors. Martins et al. (Citation2014) suggested that the observed β-carotene antioxidant capacity in the Schinus molle L. (Anacardiaceae) EO can be justified by the presence of the monoterpenes. Edziri et al. (Citation2010) reported that Retama raetam (Forssk.) Webb (Fabaceae) EO has a good AA (measured through the DPPH method), and they consider that this activity could be attributed to the relatively high percentage of monoterpenes present in the oil. Moreover, there are some reports that show that oxygenated monoterpenes, such as α-terpineol, linalool, carvacrol, piperitenone, and piperitenone oxide, were mainly responsible for the antioxidant potential of plant oils (Bicas et al., Citation2011; Maestri et al., Citation2006; Miguel, Citation2010). In this sense, Iqbal et al. (Citation2013) reported that the most abundant constituents in Mentha longifolia L. (Lamiaceae) EO were piperitenone oxide (28.3%) and piperitenone (24.9%), and they showed that the AA of its EO was similar to the piperitenone activity.
Civitelli et al. (Citation2014) reported that piperitenone oxide exerted a strong inhibitory effect against herpes simplex virus type 1 (HSV-1). HSV-1 infection is known to induce a pro-oxidative state, and this redox change in the cell is important for viral replication. These authors proposed that piperitenone oxide could interfere with some redox-sensitive cellular pathways exploited for viral replication. Considering the results reported in the literature, as well as our results, we can suggest that the observed AA in the oils studied can be mainly justified by the presence of oxygenated monoterpenes and piperitenone oxide.
According to our best knowledge, there is only one report on the antioxidant potential of L. turbinata EO (Quiroga et al., 2013). The authors have reported a good AA for this oil, finding α-limonene (76.80%) and 1,8-cineole (4.95%) as major components, while in our study, piperitenone oxide (63.0%) and limonene (7.2%) are identified as the principal components. This difference in oil composition is probably due to the existence of different chemotypes. Overall, the EO composition proved to exhibit considerable chemodiversity depending on the method of extraction and geographical origin (Lima et al., Citation2011), thus, the importance of analyzing plants from different locations.
In addition, comparing our results with those reported by Cabana et al. (Citation2013), the antiradical activity (DPPH test) of C. gilliesii EO observed herein was weaker than that found by these authors. They described piperitenone oxide as the major compound of C. gilliesii EO (68.8%), while in our study, this compound only represented 14.7% of the oil. Taking this into account, the difference in the piperitenone oxide amounts between these two C. gilliesii oils could explain their different AA.
Conclusion
The AA of EOs is a biological property of great interest because it may be used to preserve foods from the toxic effects of oxidants. Moreover, the oils that have the ability to scavenge FR may play an important role in the prevention of some diseases such as cancer, brain dysfunction, and inflammatory diseases. We found that L. turbinata oil was significantly more effective as antioxidant than the other oils studied. This oil is predominantly constituted by piperitenone oxide. These findings support the view that certain aromatic plants are potential sources of antioxidants. In this sense, the L. turbinata EO collected in the La Rioja province may be a good and safe source of natural antioxidants to be used as preservative, cosmeceutical, or nutraceutical.
Acknowledgements
The authors thank Dr. Guillermo Darbyshire for his help with the design and editing of the manuscript.
Declaration of interest
This work was supported by the Universidad Nacional de Chilecito under Grant FyCT 2012. The authors report that they have no conflicts of interest.
References
- Adams RP. (2001). Identification of Essential Oils Components by Gas Chromatography/Quadrupole Mass Spectroscopy. Illinois, USA: Allured Publishing Corporation
- Afoulous S, Ferhout H, Raoelison EG, et al. (2013). Chemical composition and anticancer, antiinflammatory, antioxidant and antimalarial activities of leaves essential oil of Cedrelopsis grevei. Food Chem Toxicol 56:352–62
- Alonso J, Desmarchelier C. (2006). Plantas medicinales autóctonas de la Argentina. Bases científicas para su aplicación en atención primaria de la salud. Buenos Aires: Fitociencia
- Bayala B, Bassole IH, Gnoula C, et al. (2014). Chemical composition, antioxidant, anti-inflammatory and anti-proliferative activities of essential oils of plants from Burkina Faso. PLos One 9:e92122
- Bicas JL, Neri-Numa IA, Ruiz AL, et al. (2011). Evaluation of the antioxidant and antiproliferative potential of bioflavors. Food Chem Toxicol 49:1610–15
- Bluma RV, Etcheverry MG. (2008). Application of essential oils in maize grain: Impact on Aspergillus section Flavi growth parameters and aflatoxin accumulation. Food Microbiol 25:324–34
- Bustos DA, Tapia AA, Feresin GE, Ariza-Espinar L. (1996). Ethnopharmacobotanical survey of Bauchazeta district, San Juan province Argentina. Fitoterapia 5:411–15
- Cabana R, Silva LR, Valentão P, et al. (2013). Effect of different extraction methodologies on the recovery of bioactive metabolites from Satureja parvifolia (Phil.) Epling (Lamiaceae). Ind Crops Prod 48:49–56
- Civitelli L, Panella S, Marcocci ME, et al. (2014). In vitro inhibition of herpes simplex virus type 1 replication by Mentha suaveolens essential oil and its main component piperitenone oxide. Phytomedicine 21:857–65
- Ebrahimabadi AH, Mazoochi A, Kashi FJ, et al. (2010). Essential oil composition and antioxidant and antimicrobial properties of the aerial parts of Salvia eremophila Boiss from Iran. Food Chem Toxicol 48:1371–6
- Edziri H, Mastouri M, Chéraif I, Aouini M. (2010). Chemical composition and antibacterial, antifungal and antioxidant activities of the flower oil of Retama raetam (Forssk.) Webb from Tunisia. Nat Prod Res 24:789–96
- Farasat M, Khavari-Nejad RA, Nabavi SM, Namjooyan F. (2013). Antioxidant properties of two edible green seaweeds from northern coasts of the Persian Gulf. Jundishapur J Nat Pharm Prod 8:47–52
- García CC, Talarico L, Almeida N, et al. (2003). Virucidal activity of essential oils from aromatic plants of San Luis, Argentina. Phytother Res 17:1073–5
- Gleiser RM, Zygadlo JA. (2007). Insecticidal properties of essential oils from Lippia turbinata and Lippia polystachya (Verbenaceae) against Culex quinquefasciatus (Diptera: Culicidae). Parasitol Res 101:1349–54
- Gleiser RM, Bonino MA, Zygadlo JA. (2011). Repellence of essential oils of aromatic plants growing in Argentina against Aedes aegypti (Diptera: Culicidae). Parasitol Res 108:69–78
- González MJ, Marioli JM. (2010). Antibacterial activity of water extracts and essential oils of various aromatic plants against Paenibacillus larvae, the causative agent of American Foulbrood. J Invertebr Pathol 104:209–13
- Iqbal T, Hussain AI, Chatha SA, et al. (2013). Antioxidant activity and volatile and phenolic profiles of essential oil and different extracts of wild mint (Mentha longifolia) from the Pakistani flora. J Anal Methods Chem 2013:536490
- Lima B, López S, Luna L, et al. (2011). Essential oils of medicinal plants from the Central Andes of Argentina: Chemical composition, and antifungal, antibacterial and insect-repellent activities. Chem Biodivers 8:924–36
- Maestri DM, Nepote V, Lamarque AL, Zygadlo JA. (2006). Natural products as antioxidants. In: Imperato F, ed. Phytochemistry: Advances in Research. Kerala: Research Signpost, 105–35
- Martins M do R, Arantes S, Candeias F, et al. (2014). Antioxidant, antimicrobial and toxicological properties of Schinus molle L. essential oils. J Ethnopharmacol 151:485–92
- Miguel MG. (2010). Antioxidant and anti-inflammatory activities of essential oils: A short review. Molecules 15:9252–87
- Mohamed AA, Ali SI, El-Baz FK. (2013). Antioxidant and antibacterial activities of crude extracts and essential oils of Syzygium cumini leaves. Plos One 8:e60269
- Ordoñez AA, Gomez D, Vattuone MA, Isla MI. (2006). Antioxidant activity of Sechium edule (Jacq) Swartz. Food Chem 97:452–8
- Pascual ME, Slowing K, Carretero E, et al. (2001). Lippia: Traditional uses, chemistry and pharmacology: A review. J Ethnopharmacol 76:201–14
- Passone MA, Etcheverry M. (2014). Antifungal impact of volatile fractions of Peumus boldus and Lippia turbinata on Aspergillus section Flavi and residual levels of these oils in irradiated peanut. Int J Food Microbiol 3:168–9
- Peschel W, Sanchez-Rabaneda F, Dieckmann W, et al. (2006). An industrial approach in the search of natural antioxidants from vegetable and fruits wastes. Food Chem 97:137–50
- Pham-Huy LA, He H, Pham-Huy C. (2008). Free radicals, antioxidants in disease and health. Int J Biomed Sci 4:89–96
- Quiroga PR, Grosso NR, Lante A, et al. (2013). Chemical composition, antioxidant activity and anti-lipase activity of Origanum vulgare and Lippia turbinata essential oils. Int J Food Sci Technol 48:642–9
- Quiroga PR, Riveros CG, Zygadlo JA, et al. (2011). Antioxidant activity of essential oil of oregano species from Argentina in relation to their chemical composition. Int J Food Sci Technol 46:2648–55
- Re R, Pellegrini N, Proteggente A, et al. (1999). Antioxidant activity applying an improve ABTS radical cation decoloration assay. Free Radic Biol Med 26:1231–7
- Ruch RJ, Cheng SJ, Klaunig JE. (1989). Prevention of cytotoxicity and inhibition of intracellular communication by antioxidant catechins isolated from Chinese green tea. Carcinogenesis 10:1003–8
- Sahin Basak S, Candan F. (2013). Effect of Laurus nobilis L. essential oil and its main components on α-glucosidase and reactive oxygen species scavenging activity. Iran J Pharm Res 12:367–79
- Sen S, Chakraborty R, Sridhar C, et al. (2010). Free radicals, antioxidants, diseases and phytomedicines: Current status and future prospect. Int J Pharm Sci Rev Res 3:91–100
- Viturro CI, Molina A, Guy I, et al. (2000). Essential oils of Satureja boliviana and S. parvifolia growing in the region of Jujuy, Argentina. Flavour Fragr J 15:377–82