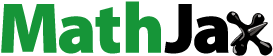
Abstract
Context Pomegranate peel (PP) has health benefits including antibacterial, antioxidant, anti-inflammatory, and antimutagenic properties.
Objective This study investigated the biochemical composition and protective effects of PP against hematotoxicity and genotoxicity induced by barium chloride (BaCl2) in adult rats.
Materials and methods Adult Wistar rats were divided into four groups of six each: control, barium (67 ppm via drinking water), PP (5% via diet), and their combination during 21 d. Oxidative stress was determined by MDA, AOPP, and antioxidant status: CAT, GPx, GSH, Vit C. Osmotic fragility (OF), chromosomal aberrations (CAs), and micronucleus (MN) assays were also studied.
Results PP showed a rich composition of antioxidant compounds. DPPH test found IC50 value= 5.3 μg/mL and a high polysaccharides content (315 ± 5 mg/g of extract). In vivo study showed a decrease in red blood cells (70%) and platelet counts (46%), hemoglobin content (8%), hematocrit percent (7%), and an 80% increase of white blood cells in Ba-treated rats. A reduction in antioxidant status: catalase, glutathione peroxidase activities, glutathione, and vitamin C levels by 31, 21, 28, and 29%, respectively, and an increase in MDA (46%) and AOPP levels (72%) were also observed compared with controls. BaCl2-treatment showed a significant increase in the frequencies of total chromosomal aberrations with abnormal metaphases and micronucleus in bone-marrow cells. Oxidative stress induced by BaCl2 might be the major cause for chromosomal abnormalities leading to DNA damage.
Discussion and conclusion A decrease in hematotoxic and genotoxic effects induced by PP is due to its powerful antioxidant capacity.
Introduction
The erythrocytes, due to their role as O2 and CO2 transporters, are under constant exposure to reactive oxygen species and oxidative stress. Their exposure to ROS leads to membrane injury, lipid peroxidation, changes in cellular morphology, osmotic fragility, protein cross-linking, and antioxidant enzymes depletion (Kolanjiappana et al. Citation2002; Okamoto et al. Citation2004). They may be considered as the sensitive and intermediate cells in oxidative reactions due to their propensity to generate radical species (Cimen Citation2008). Additionally, their membranes are highly susceptible to lipid peroxidation due to their richness in (i) polyunsaturated fatty acids, (ii) transition metal catalyst (heme–iron), and (iii) hydrogen peroxide (Ertabak et al. Citation2004).
Previous studies have demonstrated that heavy metals increase the generation of ROS causing damage to macromolecules, membrane ion transport systems, enzymes, lipid peroxidation in hematological system leading probably to genotoxic effects on blood (Kumar et al. Citation2013; Ashour & El-Shemi Citation2014) in rats treated by mercury chloride and cadmium, respectively. Barium, an alkaline earth metal, alters hematological parameters including the decrease in blood hemoglobin content and thrombocytes count in industrial workers (Tarasenko et al. Citation1977), while its genotoxicity has not been previously characterised in experimental models. Therefore, a micronucleus and chromosome aberrations tests, assayed in our study, were used for the assessment of barium genotoxicity. These assays are now recognised as the most successful and reliable assays for genotoxic carcinogens and mutagens causing genetic damages. Micronuclei are the cytoplasmic bodies having a portion of acentric chromosome or a whole chromosome not carried to the opposite poles during the anaphase (Boffetta & Islami Citation2013).
To overcome cytotoxicity and genotoxicity, herbal medicines, having antioxidant properties in reducing free radical-induced tissue injury, are increasingly used.
In this context, Punica granatum L. (Punicaceae), commonly known as pomegranate, was used in our experiment as a plant candidate providing natural sources of antioxidants. In fact, its peel has pharmacological functions. It is used for its antioxidant capacity (Thring et al. Citation2009; Panichayupakarananta et al. Citation2010; Abdel Moneim et al. Citation2013), hepatoprotective (Murthy et al. Citation2002), and hypoglycemic effects (Hontecillas et al. Citation2009).
To our knowledge, pomegranate peel alleviating genotoxicity induced by BaCl2 has not been previously examined. Thus the present study aimed, first to evaluate (i) the pro-oxidant effects of BaCl2 administered by oral way at 67 ppm on blood oxidative damage and genotoxicity, (ii) the potential protective role of pomegranate peel against BaCl2-induced toxicity, (iii) the hematological parameters and genotoxic effects in bone marrow cells.
Materials and methods
Chemicals
Barium chloride was obtained from Loba Chemie (Mumbai, India). Some chemical products used in this study were purchased from Sigma Chemical Co. (St. Louis, MO). The other ones, of analytical grade, were purchased from standard commercial suppliers.
Plant material
Biological pomegranate fruits were collected in September 2014 from Jebeniana (suburb of Sfax City located in the South of Tunisia) and identified by Doctor Ines Ksentini. A voucher specimen was deposited in herbarium at the Faculty of Pharmacy (Monastir, Tunisia). They were washed by tap water, peeled, and their edible portions were carefully separated. The peels were air dried and ground to a fine powder with a traditional stone blender.
Pomegranate peel extract (PPE) preparation
Pomegranate peel powder (250 g) was extracted with 1 L of ethanol/water (4V/1V) with refluxing for 5 h. The ethanolic extract was filtered with No. 1 Whatmann Millipore filter paper (0.45 μm Ref HAWP04700, Millipore Inc., Bedford, MA). Then it was concentrated to dryness with a rotary evaporator at 50 ± 1 °C to give a solid residue and stored at 4 °C until use.
Total phenolic content (TPC) in PP extract
The total phenolic content of pomegranate peel extract was determined according to the method of Singleton et al. (Citation1999). The reaction mixture contained 0.5 mL of Folin–Ciocalteu reagent and 0.5 mL of pomegranate peel ethanolic extract. Afterwards, 0.5 mL of 7.5% Na2CO3 solution was added to the mixture followed by incubation in the dark at ambient temperature for 30 min. Absorbance against blank was measured at 760 nm. The results were expressed as mg gallic acid equivalents (GAE) per gram of PP extract.
Total flavonoid contents (TFC) in PP extract
Flavonoid contents were determined according to the method of Zhishen et al. (Citation1999). A 500 μL aliquot of PP extract or of quercetin solution was mixed with 1.25 mL of distilled water and 75 μL of 5% NaNO2 solution. After 6 min, 150 μL of 10% aluminum chloride solution was added. Five minutes later, 0.5 mL of 1 M NaOH was added and the total volume was adjusted to 2.5 mL with distilled water and mixed. Absorbance against blank was determined at 510 nm. Quercetin was used for standard curve (0.003–0.1 mg/mL; y = 25.63x; R2 = 0.9993), where Y is the absorbance and X represents the concentrations of quercetin. The results were expressed as milligrams of quercetin equivalents (QE)/g of peel extract.
Total anthocyanin content
Anthocyanin was determined by the Giusti and Wrolstad (Citation2001) method. To determine anthocyanin content, the PP extract was diluted with Tampon solution [potassium chloride buffer (pH 1), sodium acetate buffer (pH 4.5)]. The mixture was then incubated for 15 min at room temperature and the absorbance was measured at 510 and 700 nm. The results were expressed as mg of cyanidin-3-glucoside equivalents/g of peel extract using a molar extinction coefficient of cyanidin-3-glucoside of 26.900 L/mol/cm and a molar weight of 449.2 g/mol.
Polysaccharides content in pomegranate peel
According to the method of Dubois et al. (Citation1956), the content of sugar was determined using 1 mL phenol 5% and 5 mL sulfuric acid 98% in 1 mL of sample. With the presence of these two reactants, saccharides were colored in yellow-orange. Color intensity was proportional to the concentration of carbohydrates in the solution. The optical density was then measured at 490 nm.
Antioxidant activity of PP extract (DPPH assay)
DPPH radical (DPPH•) was estimated using the method of Liyana-Pathiranan and Shahidi (Citation2005) with slight modifications. Samples of initial plant extract (0.1 mg/mL) and of Vitamin E and/or BHT used as standards, were diluted in methanol. Final concentrations of standard solutions or plant extracts (6.25, 12, 25, 50, and 100 μg/mL) were obtained. An aliquot of 1 mL of DPPH methanol solution (0.135 mM) was added to 1 mL of each sample allowing them to react in a dark place at room temperature. After 30 min, the absorbance values were measured at 517 nm and expressed in % using the following equation:
where Abscontrol is the absorbance of the DPPH•+methanol, Abssample is the absorbance of DPPH•+sample extract/standard.
The antioxidant activity of pomegranate peel extract or of vitamin E or BHT, used as standards, was expressed as IC50 value, defined by the concentration of the test extract or vitamin E or BHT required to cause a 50% decrease in initial DPPH concentration.
Animal housing and experimental design
Adult female rats of Wistar strain weighing about 168 ± 10 g were purchased from the breeding center of the Central Pharmacy (SIPHAT, Tunisia). They were maintained under standard conditions of temperature (22 ± 3 °C) and humidity (50 ± 10%) with 10 h/14 h light/dark cycle. A standard pellet diet (SNA, Sfax) and water were provided ad libitum. After acclimatisation to the laboratory conditions, 24 rats were divided into four groups of six rats each and were treated as follows: Group I (serving as a control group) was given distilled water; group II (Ba) was given 67 ppm of barium chloride via drinking water; group III (Ba + PP) received both Barium via drinking water and 5% of pomegranate peel via diet; group IV (PP) received diet containing 5% of pomegranate peel.
All animal procedures were conducted in strict conformity according to the General Guidelines on the use of living Animals in Scientific Investigation (Council of European Communities Citation1986) and approved by the ethical Committee of Sfax Faculty of Sciences. At the end of the experimental period (21 d), control and treated groups were euthanised by cervical decapitation to avoid stress conditions.
Blood sample collection
Some blood samples were collected into evacuated tubes containing EDTA solution as anticoagulant for the determination of hematological parameters. The other ones were collected into heparined tubes and centrifuged at 2200 g for 15 min. Plasma samples were then removed and the sediments containing erythrocytes were suspended in phosphate buffer saline solution (0.9% NaCl in 0.01 M phosphate buffer, pH 7.4) and centrifuged. This process was repeated twice. After removing cells’ debris by centrifugation at 3000 g for 15 min, the hemolysats were obtained and stored at −20 °C until biochemical analysis.
Extraction of bone marrow
Animals were yeast stimulated overnight and injected with an antimitotic solution (Vinblastin Sulfate) 4 min before euthanasia. After the sacrifice, two femurs were immediately dissected out and were cut at both ends with bone snips for bone marrow extraction (Valette et al. Citation2002) in order to study chromosomal aberrations and micronucleus assay.
Hematological parameters
Red blood cell (RBC), total white blood cell (WBC) and platelet counts, % hematocrit (Ht), hemoglobin (Hb) content, mean corpuscular volume (MCV), mean corpuscular hemoglobin (MCH), and mean corpuscular hemoglobin concentration (MCHC) were analyzed by electronic automate coulter MAXM (Beckman Coulter, Inc., Fullerton, CA).
Evaluation of erythrocytes osmotic fragility
Erythrocytes hemolysis was determined by osmotic fragility using NaCl solutions at different concentrations. An aliquot of 100 μL of blood samples from all studied groups was added to tubes containing saline solutions at graded concentrations (0.1–0.9% of NaCl). After a gentle mixing and standing for 30 min at room temperature, the erythrocyte suspensions were centrifuged at 1270 g for 10 min. The absorbance of released Hb into the supernatant was measured at 540 nm according to the method of Mineo and Hara (Citation2005). The amount of lysis in each tube was expressed as percent of hemolysis and was compared with a 0.1% of NaCl tube corresponding to 100% of lysis.
Protein content
Protein was determined by the Lowry et al. (Citation1951) method, using bovine serum albumin as standard.
MDA levels in erythrocytes
Lipid peroxidation (LPO) was measured by the method of Draper and Hadley (Citation1990) as thiobarbituric acid reactive substances (TBARS), since malondialdehyde (MDA) was a degradation product of peroxidised lipids. The development of pink color with the absorption at 532 nm of TBA-MDA chromophore was taken as an index of LPO. TBARS values were expressed as nanomoles of MDA per mg of protein.
Determination of AOPP levels
Advanced oxidation of protein products (AOPP) were determined according to the method of Witko et al. (Citation1992). The concentration of AOPP for each sample was calculated using the extinction coefficient of 261 cm−1 mM−1 and the results were expressed as μmoles/mg protein.
Catalase (CAT) activities
CAT was performed by measuring H2O2 concentration using the UV absorbance at 240 nm as previously described by Aebi's (Citation1984) method. CAT activity was expressed as micromoles of H2O2 consumed per minute per milligram of protein.
Glutathione peroxidase (GPx)
GPx was quantified with the continuous photometric monitoring of oxidised glutathione at 37 °C according to the method of Flohe and Gunzler (Citation1984). The conversion of NADPH to NADP was evaluated using the UV absorbance at 340 nm. GPx activity was expressed as nanomoles of GSH oxidised per minute per milligram of protein.
Glutathione (GSH) content
GSH contents were determined by Ellman’s method (Citation1959), modified by Jollow et al. (Citation1974) based on the development of a yellow color when 5,5-dithiobtis-2-nitro benzoic acid (DTNB) was added to compounds containing sulfhydryl groups. Briefly, 3 mL of 4% sulfosalicylic acid was added to 500 μl of tissue homogenate in phosphate buffer. The mixture was centrifuged at 1600 g for 15 min. Then Ellman’s reagent was added to 500 μl of supernatant. After 10 min, the absorbance was measured at 412 nm. Total GSH content was expressed as μg/mg protein.
Ascorbic acid content
Ascorbic acid determination was performed as described by Jacques-Silva et al. (Citation2001). Protein in the hemolysates was precipitated in 10 V of a cold 4% TCA solution. An aliquot of 300 μL was adjusted to a final volume of 1 mL and incubated at 38 °C for 3 h, then 1 mL of H2SO4 65% (v/v) was added to the medium. The reaction product was determined using color reagent containing 4.5 mg/mL dinitrophenylhydrazine and CuSO4 (0.075 mg/mL). The data were expressed as μmoles ascorbic acid/g of tissue.
Chromosomal aberration assays
Preparation of bone marrow cells and the assessment of chromosomal aberrations were carried out as described previously (Boujbiha et al. Citation2012; Bouaziz-Ketata et al. Citation2015). Bone marrow was extracted and incubated for 18 min at 37 °C in 8 mL of KCl 0.075 M, then centrifuged and fixed twice with fresh methanol:glacial acetic acid (3:1). The cell suspensions were dropped on cold glass slides air-dried and then stained with 5% Giemsa solution (pH 6.8) for 15 min. All slides were coded before being scored. Sixty metaphases from each point were analyzed (the total of 100 metaphases per duplicate point). Only unstable aberrations were recorded, namely double-strand breaks (DSB), exchange (EXC), dicentric (DIC), and polyploidy (PLP).
In vivo micronucleus (MN) assay
After aspiration of bone marrow with fetal calf serum, the cell suspension was centrifuged and a small drop (3 mL) of the resuspended cell pellet was spread on a microscope slide and stained with Giemsa (D’Souza & Narayana Citation2002). Three slides per animal were stained with acridine orange (AO) and washed twice with phosphate buffer (pH 6.8) as described by Hayashi et al. (Citation1994). Micronuclei were scored with a Zeiss Axioskop microscope cat 100 × (Zeiss Inc., Rochester, NY) following previously proposed criteria (Heddle et al. Citation2011). Approximately 2000 erythrocytes per animal were scored to estimate the frequency of micronucleated erythrocytes.
Statistical analysis
Statistical tests used in chromosomal rearrangements and biochemical analysis were the following: Mann–Whitney U test used for the total chromosomal aberrations (TCA) and micronucleus frequencies; Chi-square test (χ2) was used for the aberrant metaphases percentage; one-way analysis of variance (ANOVA), followed by Fisher’s Protected Least Significant Difference (PLSD) test as a post-hoc test for comparison between groups, was performed for the statistical analysis of oxidative stress parameters (All values were expressed as means ± SD. Differences were considered significant if p< 0.05).
Results
Total phenolic, flavonoid, anthocyanin, and polysaccharide contents in PP extract
Total phenolic, flavonoid, and anthocyanins contents of ethanolic pomegranate peel extract are shown in . The extraction yield was 10%. The total phenolic content of the ethanolic extract was 110 ± 0.1 mg of gallic acid equivalent (GAE)/g of extract. The total flavonoids content was 26.8 ± 0.1 mg of quercetin equivalent (QE)/g of extract. Anthocyanin and polysaccharide contents in pomegranate peel extract were, respectively, 0.25 ± 0.05 mg cyanidin-3-glucoside equivalents/g of extract and 315 ± 5 mg/g extract.
Table 1. Extraction yield (%), total phenolic (GAE/g extract), flavonoids (QE/g extract), anthocyanin (CGE/g extract), and polysaccharide (mg/g of extract) content in pomegranate peel extract.
DPPH radical-scavenging activity
As shown in , the results indicated that ethanolic pomegranate peel extract, at a concentration of 5.3 μg/mL, inhibited the formation of DPPH by 50% owing to its electron-donating ability. The DPPH• scavenging activity was enhanced with the increase of concentrations. The radical scavenging capacity of pomegranate peel extract (5.3 μg/mL) was considerably lower (p < 0.05) than that of vitamin E (IC50 value = 4 μg/mL) and higher than that of BHT (19 μg/mL), used as standards.
Hematological parameters
Data of hematological parameters are shown in . Compared with controls, a significant decrease in RBC and platelet counts, Hb level, and Ht % in the barium treated rats was observed, while no significant changes were shown in MCV, MCH, and MCHC values. Total WBC counts were significantly increased in the barium-treated group compared with those of controls. Pomegranate peel, added in the diet of barium treated rats, ameliorated the hematological parameters.
Table 2. Erythrocyte parameters in control, barium (67 ppm Ba), with both barium and pomegranate peel (Ba + PP) or pomegranate peel alone (PP 5%) during 21 d.
Erythrocyte osmotic fragility
Changes in RBCs hemolytic pattern after exposition to different concentrations of NaCl solutions were measured to determine osmotic fragility of erythrocytes in control and treated rats. As shown in , the osmotic fragility of RBCs in the barium-treated group was significantly higher than those of (Ba + PP) and control rats.
Evaluation of antioxidant and oxidative stress status in erythrocytes
MDA (an index of lipid peroxidation) and AOPP (marker of protein oxidative damage) increased by 46% and 72%, respectively, in barium-treated rats than those of controls (). Pomegranate peel, added in the diet of barium treated rats, decreased these parameters.
Table 3. Malondialdehyde (MDA), advanced oxidation protein products (AOPP), (CAT, GPx) activities, (GSH, Vitamin C) levels in erythrocytes of control and treated rats with 67 ppm of barium, PP along with Ba or PP during 21 d.
Antioxidant status was also altered by barium chloride. In fact, GPx and CAT activities were significantly decreased by 21% and 31%, respectively. A decline of GSH and vitamin C by 28% and 29% was observed in barium-treated rats. A significant recovery in GPx, CAT activities, GSH, and vitamin C content was observed in rats co-exposed to barium and pomegranate peel ().
Genotoxicity parameters
Chromosomal aberration frequencies and the percentages of aberrant metaphases are shown in . shows the chromosomal aberrations observed in bone marrow cells of control and barium treated rats. Our study showed a significant increase in total chromosomal aberrations frequencies (TCA) and in the percentage of aberrant metaphases of the barium-treated group compared with those of the control group (). Thus, we observed multiple types of chromosomal aberrations, mainly in the form of exchanges, dicentrics, breaks, and polyploidy metaphasis (see for more details).
Figure 3. Chromosomal aberrations analysis of bone marrow cells in treated rats compared to controls showing: (a) normal chromosome; (b) chromatid exchanges (EXC); (c) dicentric chromosome (DIC); (d) a double strand break (DSB); (e) polyploidy metaphasis (PLP).

Table 4. The frequencies of chromosomal aberrations and micronucleus in bone marrow cells of control and treated rats with Ba, PP, and their combination.
shows erythrocytes with or without micronucleus in bone marrow cells of control, rats treated with barium, rats co-treated with barium and pomegranate peel and treated with pomegranate peel alone.
Figure 4. Micronucleus analysis of bone marrow cells in control and treated rats with Ba (b), (Ba + PP) (c), and PP alone (d). A photomicrograph showed normal erythrocytes (solid arrow) and erythrocytes with one or more micronuclei (empty arrow).

Regarding MN assay, similar to TCA data, a significant increase was found in MN frequency in the bone marrow cells of the barium-treated group compared with controls (). Administration of pomegranate peel in the diet of barium treated rats reduced TCA and aberrant metaphase frequencies without reaching control values.
Discussion
Under natural conditions, internal antioxidant enzymes such as superoxide dismutase, catalase, and glutathione peroxidase eliminate reactive oxygen species (ROS), affording protection for the cells. Enzyme injuries could significantly affect the defensive mechanisms. In case of unbalance between free radicals and antioxidants, an oxidative stress could be induced causing chronic permanent damage (Chipman et al. Citation2006). Nowadays, erythrocytes are used to study xenobiotics effects as they are sensitive to the peroxidation process (Ghorbel et al. Citation2015). Their membranes are also susceptible to covalent damage, including cross-linking and aggregation by free radical-induced peroxidation products like superoxides resulting from Hb autooxidation.
In the present study, abnormalities in some blood cell parameters in BaCl2-treated rats were noted. A significant decrease in the erythrocytes count, Hb level, and Ht % was observed in the treated group. There was also a reduction in the platelet count which could be due to the DNA strand breakage in these cells probably induced by oxidative stress associated with BaCl2. Additionally, in our experiment, barium significantly increased the total leukocyte counts in rats, which could be due, according to Rawat et al. (Citation2013), to the compensatory increase in the production of blood cellular components by the bone marrow. Pomegranate peel in barium-treated rats decreased total leukocyte counts probably via its richness in polysaccharides content, confirming its anti-inflammatory property, as reported by us and by Lee et al. (Citation2008) and Romier et al. (Citation2008).
Impairment of hematological parameters might be due to oxidative stress probably generated by BaCl2. In fact, in the present study, the ability of barium to increase erythrocyte fragility and oxidative damage has been noted. This might be due to the increased lipoperoxidative damage in the erythrocyte membrane. The increased MDA concentration and erythrocyte fragility as a result of free radicals' induction altered lipid composition and the integrity of erythrocyte membranes. Peroxidation of membrane lipids can result in the inactivation of enzymes and cross-linking of membrane lipids and proteins (Repetto et al. Citation2012). The occurrence of protein oxidative stress in the erythrocyte of experimental rats was also confirmed by AOPP marker which reflected an excess of free radical generation and protein oxidative damages. Pomegranate peel has been shown, in the present study, to alleviate erythrocyte fragility and lipoperoxidation in barium treated rats. In fact, the significant decrease in MDA level in the co-treated group with pomegranate was in accordance with a previous study of Abdou et al. (Citation2012) after carbon tetrachloride treatment, indicating that pomegranate possesses protective effects against CCl4-induced genotoxicity and hepatotoxicity in rats. This protective effect might be attributed to its antioxidant activities (polyphenol, flavonoid, anthocyanin, and polysaccharide contents) and free radical scavenger confirmed by DPPH test. According to Qi et al. (Citation2006), sulfated polysaccharides have been reported to possess an antioxidant activity. Their activity depends on several factors, such as the sulfation degree, the molecular weight, and the sulfation positions. Additionally, anthocyanins are one of the major groups of hydrosoluble pigments belonging to the flavonoid class (Schwinn & Davies Citation2004). These compounds have an anti-inflammatory and an anticarcinogenic action to prevent cardiovascular diseases, to reduce the action of diabetes and to exhibit antioxidant capacity (He & Giusti Citation2010).
Erythrocytes are well equipped with several biological mechanisms to defend themselves against intracellular oxidative stress, including many antioxidant enzymes such as SOD, GPx, CAT, and glutathione transferase (Prasanthi et al. Citation2005) and GSH levels. Up to now, there are no studies reporting the hematotoxic effects of barium in rats. In the present study, a decrease in GPx and CAT activities as well as GSH and vitamin C levels was found after barium treatment. The reduction in CAT activity might reflect the inability of erythrocytes to eliminate H2O2 produced by barium. The reduced activity of GPx could be explained on the basis of the observed lack of the GSH substrate. Moreover, low rates of non-enzymatic antioxidants like GSH and vitamin C in the same group might be attributed to the direct conjugation of barium and/or its metabolite with these antioxidants, leading to the increased susceptibility of the blood tissue to free radical damage. Pomegranate peel, added via diet, had a potent protective effect against erythrocytes oxidative stress induced by barium, as revealed by a significant elevation of antioxidant enzyme activities and GSH and vitamin C contents. The reversal of altered antioxidant status and peroxidative damage in erythrocytes by pomegranate peel confirmed its antioxidant, anti-peroxidative properties, and its main role in the defense against free radicals, which could be attributed to its antioxidant potential.
Up to now, the detailed mechanism of barium-mediated toxicity is still unknown. Our data showed that this metal-induced oxidative stress could cause free radical reactions to produce deleterious modifications in membranes, proteins, enzymes, and genotoxicity. In fact, the mutagenic effects of barium were examined with the chromosomal aberrations and micronucleus assays in bone marrow cells. Genetic damage at the chromosomal level entails alterations in either chromosomal number or chromosome structure, and such alterations can be measured as chromosomal aberrations or micronucleus frequency. The study of DNA damage at the chromosomal level is an essential part of genotoxicity because CA is an important event in the development of mutations. The MN assay has emerged as one of the preferred methods in order to quantify and to assess genetic damage (Krishna & Hayashi Citation2000). In our study, the mutagenic effects of barium were confirmed via the significant increase in chromosomal aberrations and micronuclei in bone marrow cells. The DNA damage could be attributed to oxidative stress. In fact, it has been reported that oxidative stress, and especially ROS, may affect biological molecules such as proteins and phospholipids, causing protein modification and lipid peroxidation that could affect the deoxyribonucleosides which produced the DNA adducts (Blair Citation2008). Lines of evidence in favor of this hypothesis were reflected by the increase of MDA levels and the variations in antioxidant enzyme activities in the erythrocytes of barium-treated rats. The protective effects of pomegranate peel against BaCl2 genotoxicity have not been reported earlier and this is the first report on the mammalian system. Our data showed an eventual scavenging of the physiologically relevant radicals by dietary pomegranate peel. According to previous studies, pomegranate peel is a source of a natural substance called ellagic acid which inhibits cancer mutation by latching onto DNA-masking sensitive sites on the genetic material that might be occupied by harmful chemicals (Abdou et al. Citation2012). Also, previous findings have noted that pomegranate containing polyphenols suppresses the growth of cancer cells by interfering with its genetic factors leading to death (Abdou et al. Citation2012). Phenylpropanoids, hydrobenzoic asides, flavones, and conjugated fatty acids which are present in the pomegranate juice can inhibit a specific protein found in bone marrow, responsible for bone cancer (Zeweil et al. Citation2013).
In conclusion, our data indicated chromosomal aberrations and micronuclei formation in adult rats and revealed genotoxicity induced by barium treatment. In addition, hematological parameters and erythrocyte osmotic fragility were affected. Barium cytotoxic potential was objectified by an increase in AOPP and MDA levels. Pomegranate peel alleviated toxicity by scavenging free radicals and preventing DNA damage. It seemed to be a potential preventive disease due to its antioxidant and antimutagenic capacities.
Funding information
The present work was supported by the Ministry of Higher Education and Scientific Research in Tunisia (UR/11ES-70).
Disclosure statement
The authors report that they have no conflicts of interest.
References
- Abdel Moneim AE, Othman MS, Mohmoud SM, El-Deib KM. 2013. Pomegranate peel attenuates aluminum-induced hepatorenal toxicity. Toxicol Mech Methods. 23:624–633.
- Abdou HS, Salah SH, Hoda FB, Abdel Rahim EA. 2012. Effect of pomegranate pretreatment on genotoxicity and hepatotoxicity induced by carbon tetrachloride CCl4 in male rats. J Med Plants Res. 6:3370–3380.
- Aebi H. 1984. Catalase in vitro. Methods Enzymol. 105:121–126.
- Ashour TH, El-Shemi AG. 2014. Caffeic acid phenyl ester prevents cadmium intoxication induced disturbances in erythrocyte indices and blood coagulability, hepatorenal dysfunction and oxidative stress in rats. Acta Haematol Pol. 45:272–278.
- Blair IA. 2008. DNA adducts with lipid peroxidation products. J Biol Chem. 283:15545–15549.
- Boffetta P, Islami F. 2013. The contribution of molecular epidemiology to the identification of human carcinogens: current status and future perspectives. Ann Oncol. 24:901–908.
- Bouaziz-Ketata H, Ben Salah G, Mahjoubi A, Aidi Z, Kallel C, Kammoun H, Fakhfakh F, Zeghal N. 2015. Hyparrheniahirta: a potential protective agent against hematotoxicity and genotoxicity of sodium nitrate in adult rats. Environ Toxicol. 30:1275–1284.
- Boujbiha MA, Ben Salah G, Ben Feleh A, Saoudi M, Kamoun H, Bousslema A, Ommezzine A, Said K, Fakhfakh F, El Feki A. 2012. Hematotoxicity and genotoxicity of mercuric chloride following subchronic exposure through drinking water in male rats. Biol Trace Elem Res. 148:76–82.
- Chipman JK, Parsons JL, Beddowes EJ. 2006. The multiple influences of glutathione on bromate genotoxicity: implications for the dose–response relationship. Toxicology. 221:187–189.
- Cimen MY 2008. Free radical metabolism in human erythrocytes. Clin Chim Acta. 390:1–11.
- Council of European Communities. 1986. Council instructions about the protection of living animals used in scientific investigations. Off J Eur Commun JO86/609/CEEL358.1-18.
- Draper HH, Hadley M. 1990. Malondialdehyde determination as index of lipid peroxidation. Method Enzymol. 86:421–431.
- DuBois M, Gilles KA, Hamilton JK, Rebers PA, Smith F. 1956. Colorimetric method for determination of sugars and related substances. Anal Chem. 28:350–356.
- D'Souza UJ, Narayana K. 2002. Mechanism of cytotoxicity of ribavirin in the rat bone marrow and testis. Ind J Physiol Pharmacol. 46:468–474.
- Ellman GL. 1959. Tissue sulfhydryl groups. Arch Biochem Biophys. 82:70–77.
- Ertabak A, Kutluay T, Unlu A, Türközkan N, Cimen B, Yaman H. 2004. The effect of desferrioxamine on peroxynitrite-induced oxidative damage in erythrocytes. Cell Biochem Funct. 22:149–152.
- Flohe L, Gunzler WA. 1984. Assays of glutathione peroxidase. Methods Enzymol. 105:114–121.
- Ghorbel I, Maktouf S, Kallel C, Ellouze Chaabouni S, Boudawara T, Zeghal N. 2015. Disruption of erythrocyte antioxidant defense system, hematological parameters, induction of pro-inflammatory cytokines and DNA damage in liver of co-exposed rats to aluminium and acrylamide. Chem Biol Interact. 236:31–40.
- Giusti MM, Wrolstad RE. 2001. Anthocyanins. Characterization and measurement with UV–visible spectroscopy. In: Wrolstad RE, editor. Current protocols in food analytical chemistry. New York: John Wiley & Sons. F1.2.1–2.13.
- Hayashi M, Tice RR, MacGregor JT, Anderson D, Blakey DH, Kirsh-Volders M, Oleson FB Jr, Pacchierotti F, Romagna F, Shimada H, et al. 1994. In vivo rodent erythrocyte micronucleus assay. Mutat Res. 312:293–304.
- He J, Giusti MM. 2010. Biosynthesis of anthocyanins and their regulation in colored grapes. Molecules. 15:9057–9091.
- Heddle JA, Fenech M, Hayashi M, MacGregor JT. 2011. Reflections on the development of micronucleus assays. Mutagenesis. 26:3–10.
- Hontecillas R, O'Shea M, Einerhand A, Diguardo M, Bassaganya-Riera J. 2009. Activation of PPAR gamma and alpha by punicic acid ameliorates glucose tolerance and suppresses obesity-related inflammation. J Am Coll Nutr. 28:184–195.
- Jacques-Silva MC, Nogueira CW, Broch LC. 2001. Diphenyl diselenide and ascorbic acid changes deposition of selenium and ascorbic acid in liver and brain of mice. Pharmacol Toxicol. 88:119–125.
- Jollow DJ, Mitchell JR, Zampaglione N, Gillette JR. 1974. Bromobenzene-induced liver necrosis. Protective role of glutathione and evidence for 3,4-bromobenzene oxide as the hepatotoxic metabolite. Pharmacology. 11:151–169.
- Kolanjiappana K, Manoharana S, Kayalvizhib M. 2002. Measurement of erythrocyte lipids, lipid peroxidation, antioxidants and osmotic fragility in cervical cancer patients. Clin Chim Acta. 326:143–149.
- Krishna G, Hayashi M. 2000. In vivo rodent micronucleus assay: protocol, conduct and data interpretation. Mutat Res. 455:155–166.
- Kumar D, Singh S, Singh AK, Rizvi SI. 2013. Pomegranate (Punica granatum) peel extract provides protection against mercuric chloride-induced oxidative stress in Wistar strain rats. Pharm Biol. 51:441–446.
- Lee SI, Kim BS, Kim KS, Lee S, Shin KS, Lim JS. 2008. Immune-suppressive activity of punicalagin via inhibition of NFAT activation. Biochem Biophys Res Commun. 371:799–803.
- Liyana-Pathiranan CM, Shahidi F. 2005. Antioxidant activity of commercial soft and hard wheat Triticum aestivum L. as affected by gastric pH conditions. J Agric Food Chem. 53:2433–2440.
- Lowry OH, Rosebrough NJ, Farr AL, Randall RJ. 1951. Protein measurement with the Folin phenol reagent. J Biol Chem. 193:265–275.
- Mineo H, Hara H. 2005. Structure-dependent and receptor-independent increase in osmotic fragility of rat erythrocytes by short-chain fatty acids. Biochim Biophys Acta. 1713:113–117.
- Murthy KN, Jayaprakasha GK, Singh RP. 2002. Studies on antioxidant activity of pomegranate Punica granatum peel extract using in vivo models of peel using vivo. J Agric Food Chem. 50:4791–4795.
- Okamoto K, Maruyama T, Kaji Y, Harada M, Mawatari S, Fujino T, Uyesaka N. 2004. Verapamil prevents impairment in filterability of human erythrocytes exposed to oxidative stress. Jpn J Physiol. 54:39–46.
- Panichayupakarananta P, Issuriya A, Sirikatitham A, Wang W. 2010. Antioxidant assay-guided purification and LC determination of ellagic acid in pomegranate peel. J Chromatogr Sci. 48:456–459.
- Prasanthi K, Marulidhara R, Rajini PS. 2005. Morphological and biochemical perturbations in rat erythrocytes following in vitro exposure to fenvalerate and its metabolite. Toxicol In Vitro. 19:449–456.
- Qi H, Zhang Q, Zhao T, Hu R, Zhang K, Li Z. 2006. In vitro antioxidant activity of acetylated and benzoylated derivatives of polysaccharide extracted from Ulva pertusa Chlorophyta. Bioorg Med Chem Lett. 16:2441–2445.
- Rawat SK, Singh RK, Bansode FW, Singh P, Singh RP. 2013. Nitrate induced toxicity on some haematological parameters of Charles Foster rats. JRAAS. 28:35–38.
- Repetto M, Semprine J, Boveris A. 2012. Lipid peroxidation: chemical mechanism, biological implications and analytical determination. In: Catala A, editor. Lipid peroxidation. Argentina: InTech. Doi:10.5772/45943.
- Romier B, Van DeWalle J, During A, Larondelle Y, Schneider YJ. 2008. Modulation of signalling nuclear factor-kappaB activation pathway by polyphenols in human intestinal Caco-2 cells. Br J Nutr. 100:542–551.
- Schwinn KE, Davies KM. 2004. Flavonoids. In: Davies KM, editor. Plant pigments and their manipulation. Vol. 14. Oxford, UK: Blackwell. p. 92–149.
- Singleton VL, Orthofor R, Lamuela-Raventos RM. 1999. Analysis of total phenols and other oxidation substrates and antioxidants by means of Folin–Ciocaltau reagent. Methods Enzymol. 299:152–178.
- Tarasenko NY, Pronin OA, Silayev AA. 1977. Barium compounds as industrial poisons an experimental study. J Hyg Epidemiol Microbiol Immunol. 21:361–373.
- Thring TS, Hili P, Naughton DP. 2009. Anti-collagenase, anti-elastase and anti-oxidant activities of extracts from 21 plants. BMC Complement Alternat Med. 9:27.
- Valette H, Dolle F, Bottlaender M, Hinnen F, Marzin D. 2002. Fluoro-A-85380 demonstrated no mutagenic properties in in vivo rat micronucleus and Ames tests. Nucl Med Biol. 29:849–853.
- Witko V, Nguyen AT, Descamps-Latscha B. 1992. Microtiter plate assay for phagocyte-derived taurine-chloramines. J Clin Lab Anal. 6:47–53.
- Zeweil HS, ElNagar S, Zahran SM, Ahmed MH, El-Gindy Y. 2013. Pomegranate peel as a natural antioxidant boosts bucks' fertility under Egyptian summer conditions. World Rabbit Sci. 21:33–39.
- Zhishen J, Mengcheng T, Jianming W. 1999. The determination of flavonoid contents in mulberry and their scavenging effects on superoxide radicals. Food Chem. 64:555–559.