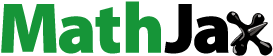
Abstract
Context: The main barrier for transdermal delivery is the obstacle property of the stratum corneum. Many types of chemical penetration enhancers have been used to breach the skin barrier; among the penetration enhancers, terpenes are found as the most highly advanced, safe, and proven category.
Objective: In the present investigation, the terpenes anethole, menthone, and eugenol were used to enhance the permeation of valsartan through rat skin in vitro and their enhancement mechanism was investigated.
Materials and methods: Skin permeation studies of valsartan across rat skin in the absence and the presence of terpenes at 1% w/v, 3% w/v, and 5% w/v in vehicle were carried out using the transdermal diffusion cell sampling system across rat skin and samples were withdrawn from the receptor compartment at 1, 2, 3, 4, 6, 8, 10, 12, and 24 h and analysed for drug content by the HPLC method. The mechanism of skin permeation enhancement of valsartan by terpenes treatment was evaluated by Fourier transform infrared spectroscopy (FTIR) analysis and differential scanning calorimetry (DSC).
Results: All the investigated terpenes provided a significant (p < 0.01) enhancement in the valsartan flux at a concentration of 1%, and less so at 3% and 5%. The effectiveness of terpenes at 1% concentration was in the following order: anethole > menthone > eugenol with 4.4-, 4.0-, and 3.0-fold enhancement ratio over control, respectively. DSC study showed that the treatment of stratum corneum with anethole shifted endotherm down to lower melting point while FTIR studies revealed that anethole produced maximum decrease in peak height and area than other two terpenes.
Conclusion: The investigated terpenes can be successfully used as potential enhancers for the enhancement of skin permeation of lipophilic drug.
Keywords:
Introduction
Transdermal delivery of drugs promises many benefits over an oral or intravenous administration. Major advantages provided by transdermal delivery includes improved bioavailability, more uniform plasma levels, longer duration of action resulting in less dosing frequency, reduced side effects, and improved therapy due to the maintenance of plasma levels (Ahad et al. Citation2013a, Citation2014a, Citation2015).
Human skin provides an effective obstacle to the penetration of most actives in the form of stratum corneum (Ahad et al. Citation2011a,Citationb). The success of all transdermal systems depends on the ability of the drug to permeate the skin in sufficient quantities to achieve its desired therapeutic effect (Roberts Citation1997). Many overtures have been employed to enhance the skin permeation rate of active moieties. However, the most convenient and widely implemented approach is the use of chemical penetration enhancers (Ahad et al. Citation2009; Hassan et al. Citation2010) such as dimethyl sulfoxide, dimethylformamide, azone, ionic surfactants, but their use is also associated with unpleasant and toxic side effects. In recent years, there has been a search for natural permeation enhancers to improve drug permeation that also exhibits low toxicity while maintaining their enhancing activity. The natural absorption promoters documented so far include terpenes, essential oils, terpenoids, fatty acid esters, fatty acid glycols, and herbal extracts (Vashistha et al. Citation2014).
Terpenes are an effective class of permeation enhancers obtained from natural sources (Akbari et al. Citation2015). Further, quite a few terpenes are included in the list of Generally Recognized As Safe (GRAS) agents issued by the US Food and Drug Administration (US FDA) (Aqil et al. Citation2007; Sapra et al. Citation2008). They cause no skin toxicity or if any, only mild irritation. Even terpenes, which are considered to be skin irritants, do not cause lasting erythema (Ahad et al. Citation2009). Terpenes have been used for permeation enhancement of both hydrophilic and lipophilic drugs. The activity of terpenes as penetration enhancers is primarily related to their chemical structure as well as the physico-chemical properties (Aqil et al. Citation2007; Ahad et al. Citation2011b).
Valsartan, orally active and specific angiotensin II receptor blocker acting on the AT1 receptor subtype, is a lipophilic antihypertensive drug that has a low oral bioavailability of about 25%. It has low molecular weight (435.5 Da) and melting point (116–117 °C) with a log partition coefficient of 4.5, pKa (4.73), and mean biological half-life of 7.5 h. There are no reports of skin irritation attributed to valsartan and it has been previously identified as a promising candidate for transdermal drug delivery (Rizwan et al. Citation2008; Nishida et al. Citation2010; Ahad et al. Citation2011b, Citation2012, Citation2013b, Citation2014b). All the above characteristics make valsartan a good candidate for transdermal delivery (Ahad et al. Citation2014b,Citationc).
In view of the renewed interest in herbal components, in the present study, we tried to improve the penetration of valsartan by using natural permeation enhancer such as anethole, eugenol, and menthone and to elucidate the mechanism of skin permeation enhancement by differential scanning calorimetry (DSC) and Fourier transform infrared spectroscopy (FTIR) studies. Both methods FTIR and DSC provide independent but complementary data about the interaction of terpenes with stratum corneum (Tenjarla et al. Citation1999). Some important properties of investigated terpenes are mentioned in .
Table 1. Some important physical parameters of investigated terpenes.
Materials and methods
Chemicals and materials
Terpenes such as anethole, eugenol, and menthone were purchased from (Sigma-Aldrich Chemicals Private Limited, New Delhi, India). Valsartan was received as gratis sample from Ranbaxy Research Laboratories Ltd, Gurgaon, India. Sodium hydroxide and potassium dihydrogen orthophosphate were purchased from Merck India Ltd., Mumbai, India. Sodium azide, sodium chloride, and potassium sulphate were purchased from S.D. Fine Chemicals, Mumbai, India. Water for HPLC was purchased from Thomas Baker (Chemicals) Ltd, Mumbai, India. Absolute ethanol was purchased from Changshuyangyuan Chemical, Mainland, China. Acetonitrile (HPLC grade) was purchased from Spectrochem Pvt Ltd, Mumbai, India. All other chemicals used were of analytical grade. All materials were used as received. Double distilled water was used for all experiments.
Animals
Wistar albino rats (120–125 g) were supplied by Central Animal House of Hamdard University and inhabited under standard laboratory conditions in 12 h light/dark cycle at 25 ± 2 °C. Animals were nourished with pellet diet (Lipton, Darjeeling, India) and water ad libitum. The animals were received after the study was duly approved by the Jamia Hamdard University Animal Ethics Committee, and CPSCEA (Committee for the purpose of control and supervision on experiments on animals), Government of India.
Preparation of rat skin
Wistar rat was sacrificed with prolonged ether anaesthesia; the abdominal skin of each rat was excised. The hairs on the skin of animals were removed with electric clipper; subcutaneous tissues were surgically removed, and dermis side was wiped with isopropyl alcohol to remove residual adhering fat. The skin was washed with isotonic phosphate buffer, wrapped in aluminium foil and stored in a deep freezer at −20 °C until further use (used within 2 weeks of preparation). On the day of experiment, skin was brought to room temperature and skin samples were mounted over the diffusion cells in such a way that stratum corneum side faced the donor compartment whereas the dermis faced the receiver compartment (Jain et al. Citation2002; Narishetty and Panchagnula Citation2004a).
In vitro skin permeation studies
Following the skin preparation procedure, the skin samples were cut and trimmed to the appropriate size and were mounted over the diffusion cells in such a way that stratum corneum side faced the donor compartment whereas dermis faced the receiver compartment (Amin et al. Citation2008; Manosroi et al. Citation2011a,Citationb). In vitro skin permeation studies were carried out through rat skin using transdermal diffusion cell sampling system (SFDC 6, LOGAN Instruments, Somerset, NJ). The system consisted of three side by side cells with a diffusion area of 0.636 cm2 and 4 ml of receptor cell volume. The water was warmed by the in-built heater thermostated set at 37 ± 1 °C throughout the experiments to provide a skin surface temperature of approximately (i.e., 32 ± 1 °C) (Moghimi et al. Citation2009; Mura et al. Citation2009). A Teflon-coated mini magnetic bead was kept in the receiver compartment for agitating the contained vehicle (ethanol:isotonic phosphate buffer, pH 7.4) (40:60) at 600 rpm. Following the stabilisation of the skin, the donor chamber was filled with 4 ml solution of valsartan (20 mg, calculated transdermal dose of valsartan) in vehicle with or without terpenes (1–5% w/v) (Narishetty and Panchagnula Citation2004a,Citationb; Ahad et al. Citation2011a). The donor and receiver compartment was covered with a Teflon plug to prevent evaporation of the vehicle. After application of drug solution, 0.5 ml samples were withdrawn from the receptor compartment at different time intervals and analysed for drug content by the HPLC method (Tatar and Saglik Citation2002; Ahad et al. Citation2012, Citation2013b, Citation2014b).
HPLC analysis of samples from receiver solutions
Each sample was injected into a HPLC system, consisted of a series LL-10AT VP Shimadzu pump, and SPD-10A Shimadzu UV/Vis detector (Shimadzu Corporation, Kyoto, Japan). The data were acquired and processed by Shimadzu Class-VP series software (Shimadzu Corporation, Kyoto, Japan). The Shiseido C-18 column (5 μm, 250 mm × 4.6 mm i.d) was used (Shimadzu Corporation, Kyoto, Japan). The mobile phase consisted of 45% acetonitrile and 55% phosphate buffer solution (pH 3), filtered through a 0.45 μm Millipore filter (Waters Corporation, Milford, MA), and degassed prior to use. The flow rate was 1.3 ml/min. Valsartan was analysed at a wavelength of 265 nm. Receiver volume was immediately replenished with the same amount of fresh vehicle.
Calculation of the in vitro data
Permeation parameters like flux, permeability coefficient, lag time (tL), and enhancement ratio (ER) were calculated. The cumulative amount of drug permeated was plotted as a function of time. Skin permeation rate (flux) of the drug at a steady state was calculated from the slope of the linear portion of the cumulative amount permeated through the rat skin per unit area versus time plot (Yamane et al. Citation1995; Narishetty and Panchagnula Citation2004a). The permeability coefficient was calculated as the quotient of flux and drug concentration in the donor compartment. The tL was determined by extrapolating the linear portion of the plot to the abscissa. In order to determine the extent of enhancement, the enhancement ratio (ER) was calculated as follows:
Statistical data were analysed using one-way analysis of variance. A Dunette test using GRAPHPAD INSTAT 3 software (GraphPad Software Inc, San Diego, CA) was used for statistical analysis, and the level of significance was taken as p < 0.05.
DSC studies
Approximately 20 mg of freshly prepared rat skin was taken and hydrated over saturated potassium sulfate solution for 3 d. Percent hydration was calculated using the following formula:
(2)
(2)
Hydrated rat skin samples were dipped into respective terpene solutions (1% w/v) in 4 ml vehicle for 24 h (equivalent to the duration of permeation studies). After the terpene treatment, sample was removed and blotted to attain hydration of 20–25%, cut (5 mg) and sealed in aluminum hermatic pans, and equilibrated for 1 h before the DSC run (Jain et al. Citation2002; Vaddi et al. Citation2002a,Citationb). Then, the skin samples were scanned on a DSC6 differential scanning calorimeter (Perkin Elmer, Überlingen, Germany). Scanning rate was 5 °C/min over the temperature range of 30–200 °C (Krishnaiah et al. Citation2002b; Vaddi et al. Citation2002a; Shakeel et al. Citation2008).
FTIR studies
Rat skins were prepared as mentioned earlier and the skin was cut into small circular disc with an approximate diameter of 1.5 cm. Equal volumes of sodium chloride (0.9% w/v) solution were placed in a different conical flask and skin samples of approximate diameter 1.5 cm was floated over for 3 d. After 3 d of hydration, these discs were thoroughly blotted over filter paper and FTIR (Perkin Elmar, Überlingen, Germany) spectrum was recorded before terpenes treatment (Control) in the frequency range of 400–4000 cm−1, with a resolution of 2 cm−1. Each spectrum was an average of 60 scans. In terpenes-treated skin samples, skin samples were dipped into a respective terpenes solution (1% w/v) in 4 ml vehicle for 24 h (equivalent to the duration of permeation studies). Each sample disc after treatment was washed, blotted dry, and then air dried for 2 h. Samples were kept under vacuum in desiccators for 15 min to remove traces of solvent and enhancer completely (Krishnaiah et al. Citation2003). FTIR spectra of all sample discs treated with terpenes were recorded again for comparison.
Results
In vitro skin permeation studies
The results of the in vitro skin permeation studies are presented in ). The passive diffusion (without enhancer) of valsartan through rat skin produced a flux of 39.3 μg/cm2/h (). All the investigated terpenes used in the study provided a significant (p < 0.01) increase in the flux at a concentration of 1% w/v. The effectiveness of terpenes at 1% concentration was in the following order: anethole > menthone > eugenol. The flux value dipped when the terpene concentration was increased to 3% w/v in case of all enhancers (and ). When the concentration was increased to 5% w/v, valsartan flux value further decreased (and ). This implies that 1% w/v is the optimised concentration for anethole, menthone, and eugenol, which produced a 4.4 ± 1.7-, 4.0 ± 1.1-, and 3.0 ± 0.6-fold enhancement ratio over control, respectively ().
Figure 1. Permeation profile of valsartan across rat skin in the absence and presence of various terpenes at (a) 1% w/v, (b) 3% w/v, and (c) 5% w/v in vehicle (mean ± SD).
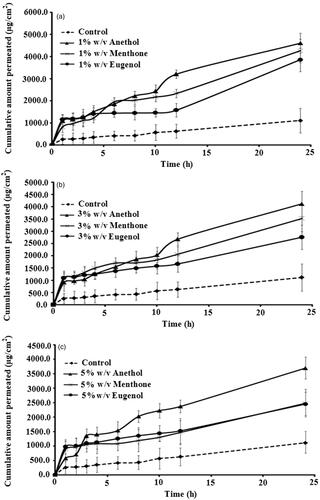
Table 2. Effect of different terpenes on permeation of valsartan across rat skin (mean ± SD).
DSC studies
In DSC study of skin treated with investigated terpenes, it was observed that both T2 and T3 endotherms completely disappeared or shifted to lower melting points in thermogram of stratum corneum treated with the terpenes (anethole, menthone, and eugenol). Treatment with anethole and eugenol decreases the protein endotherm T4 to lower melting points. However, in case of menthone treatment, there was no substantial change in T4. Another observation was that treatment of stratum corneum with anethole shifted T4 down to 106 °C with broadening of the peak (). Hence, there was no surprise that anethole produced highest flux value of valsartan through rat skin as compared to other two terpenes.
FTIR studies
The lipid extraction resulting from the terpenes treatment (1% w/v) was evaluated by comparing the intensities of the asymmetric and symmetric C–H stretching absorbance after terpenes treatment to the corresponding peaks with control treatment. shows the peak heights under the asymmetric and symmetric C–H stretching. FTIR spectra of stratum corneum treated with terpenes exhibited decreased height and area of asymmetric and symmetric CH– stretching suggesting the extraction of lipids from stratum corneum by the above terpenes (). The maximum extraction of lipids was observed with anethole as the decrease in peak height and area was the highest. The above observations are in conformity to the in vitro skin permeation results which indicated highest skin permeation rate (flux) values when anethole was added as an enhancer in the vehicle. The peak shift to higher wave number was not observed, it is clear that study terpenes did not fluidise the stratum corneum lipids (Gumming and Winfield Citation1994; Zhao and Singh Citation1998; Vaddi et al. Citation2002a; Krishnaiah et al. Citation2006). There was a substantial change in the pattern of amide I peak when anethole was used as an enhancer (
Figure 4. FTIR spectra of rat skin. Change in amide I (1640 cm−1) and amide II (1550 cm−1) vibrations after 24 h treatment with (a) control, (b) anethole, (c) menthone, and (d) eugenol.
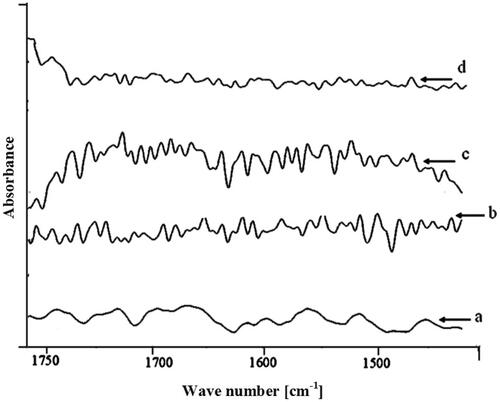
Figure 3. FTIR spectra of rat skin. Change in lipid C–H stretching (2920 cm−1) vibrations after 24 h treatment with (a) control, (b) anethole, (c) menthone, and (d) eugenol.
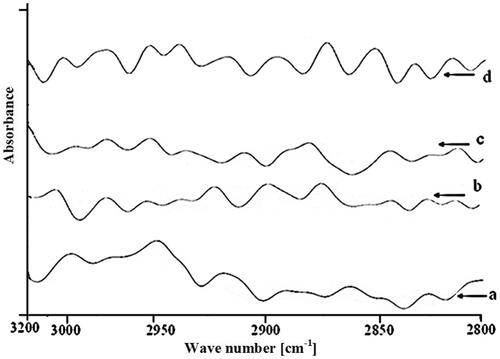
Table 3. Peak height of asymmetric CH and symmetric CH stretching absorbance before and after treatment of rat stratum corneum with control or 1% terpenes solutions for 24 h and their percentage decrease.
Discussion
The solubility of valsartan in selected vehicle was previously found to be 47.08 mg/ml (Rizwan et al. Citation2008). Ethanol is used as a co-solvent as well as skin penetration enhancer in many transdermal therapeutic systems (Krishnaiah et al. Citation2002a; Narishetty and Panchagnula Citation2004a; Krishnaiah et al. Citation2005; Fang et al. Citation2008; Rhee et al. Citation2008). Hence, we have selected vehicle (ethanol:isotonic phosphate buffer, 7.4, 40:60) as a receiver medium for in vitro skin permeation studies.
At 1% terpene concentration (), the transdermal flux of valsartan was more than at 3% and 5% of terpene. A similar conclusion was drawn in the case of a zidovudine transport study (Kararli et al. Citation1995) and propranolol (Kunta et al. Citation1997); the permeation of these drugs did not increase by increasing the concentration beyond 5%, with all terpenes studied (t-anethole, carvacrol, thymol, linalool, and menthol).
There was no explanation available in these reports as to why there was a slight decrease in penetration enhancement at higher concentrations. One possible reason in our study could be the limited solubility of terpenes in 40% v/v of ethanol solution used in the experiments. It is reported that in the penetration of indomethacin, as high as 70% v/v ethanol solution was used to completely solubilise the terpenes (Okabe et al. Citation1989; Shirakura et al. Citation1995).
It is anticipated that lipophilic terpenes would provide a better permeation enhancement for a lipophilic drug whereas hydrophilic enhancers are more active towards promoting the permeation of hydrophilic permeants (Yamane et al. Citation1995; Williams and Barry Citation2004; Aqil et al. Citation2007). The log P value of terpenes () appears as a predictor in many QSAR models (Yamashita and Hashida Citation2003; Geinoz et al. Citation2004; Sapra et al. Citation2008). Kang et al. (Citation2007) determined permeability coefficient of different terpenes experimentally using human skin and employed non-linear regression model. Their results suggested that (a) liquid terpenes have a tendency to produce ameliorate enhancing effects than solid terpenes; (b) terpenes with higher Log P values were more effective enhancers than those with smaller Log P as it was easier for lipophilic terpenes to get mixed with stratum corneum intercellular lipids (Ghafourian et al. Citation2004; Narishetty and Panchagnula Citation2004a).
The results of this study are in agreement with the above hypothesis as anethole [Log P = 3.39 ()] being lipophilic compound yielded higher flux (p < 0.05) for valsartan (a lipophilic drug). Furthermore, eugenol being the least lipophilic terpene produced the least penetration of valsartan. This finding agrees with the study that hydrophobic terpenes such as limonene were effective in enhancing the transport of lipophilic molecules such as indomethacin and ketoprofen (Kunta et al. Citation1997; Levison et al. Citation1994; El-Kattan et al. Citation2000). The lipophilic structure of valsartan makes it more permeable to lipophilic layers for the skin, and this result is consistent with the fact that lipophilicity of a drug is an important parameter for its percutaneous absorption (Thomas and Panchagnula Citation2003). At the end of 24 h period, anethole enhanced the percutaneous absorption of valsartan significantly (p < 0.05). Our studies have shown clearly () that incorporation of anethole increased the rate of permeation across rat skin by up to 4.4 times when compared with control.
In DSC, thermogram of control sample of rat epidermis presented four endotherms (). The first three endotherms were obtained at 34 °C (T1), 83.13 °C (T2), and 104.56 °C (T3), respectively. Whereas, the fourth endotherm (T4) produced a very sharp and prominent peak at 114 °C which is attributed to stratum corneum proteins (Yamane et al. Citation1995; Vaddi et al. Citation2002b; Narishetty and Panchagnula Citation2004a). The first endotherm (having the lowest enthalpy) is attributed to sebaceous secretions (Lee et al. Citation2005) or surface contamination and to minor structural rearrangement of lipid bilayer and is some time completely removed by lipid extracting solvents (Yamane et al. Citation1995). The second and the third endotherms T2 and T3 appear due to the melting of stratum corneum lipids and the fourth endotherm T4 has been assigned to intracellular keratin denaturation. Fading or shifting of T2 and T3 endotherms to lower melting points in thermogram of stratum corneum treated with the terpenes (anethole, menthone, and eugenol) indicates that the terpenes present in the vehicle enhance skin permeation of drug through extraction of stratum corneum lipids (Yamane et al. Citation1995; Jain et al. Citation2002; Krishnaiah et al. Citation2002a,Citationb, Citation2003). Anethole and eugenol treatments decrease the protein endotherm T4 to lower melting points, suggesting keratin denaturation and possible intracellular permeation mechanism in addition to the extraction of lipid bilayers by terpenes (). While no significant change in T4 was observed when skin was treated with menthone, thus precluding the intracellular transport as a probable mechanism of permeation enhancement, thus, leading to conclusion that extraction of stratum corneum lipids is the only mechanism of penetration enhancement for menthone.
The mechanism of skin permeation enhancement by the investigated terpenes was further elucidated by FTIR studies. The FTIR spectrum of control sample shows various peaks due to molecular vibration of lipids and proteins in the stratum corneum. The absorption bands from 3000 to 2800 cm−1 are due to the C–H stretching motions of the alkyl groups present in both proteins and lipids. The signal linked to proteins is a broad band, rather weak compared with the lipids absorption which exhibits four peaks at around 2850 cm−1, 2920 cm−1, 2870 cm−1 and 2955 cm−1 (Gumming and Winfield Citation1994; Zhao and Singh Citation1998; Panchagnula et al. Citation2001; Krishnaiah et al. Citation2006). The bands at 2920 cm−1 and 2850 cm−1 are due to the asymmetric –CH2 and symmetric –CH2 vibrations of long-chain hydrocarbons of lipids. These narrow bands are attributable to the long alkyl chains of ceramides, cholesterol, and fatty acids, which are the major components of the stratum corneum lipids (Amnuaikit et al. Citation2005). The two strong bands at 1650 cm−1 and 1550 cm−1 are due to the amide I and amide II stretching vibrations of stratum corneum proteins. The amide I bands arises from C = O stretching vibration and amide II bands from C–N bending vibration. The amide I band consisting of component bands, represents various secondary structures of keratin. It is evident from the literature that the presence of ethanol (66.6%) in the vehicle with or without terpenes does not produce significant changes in the peak height and peak area with respect to different components of skin (proteins and lipids) (Narishetty and Panchagnula Citation2004a, Citationb). Hence, selected vehicle-treated skin specimen served as the control in order to compare the changes in the FTIR spectra caused by different investigated terpenes.
The rate limiting step or main barrier to transdermal drug delivery is the lipophilic part of stratum corneum in which lipids (ceramides) are tightly packed as bilayers due to high degree of hydrogen bonding. The amide I group of ceramide is hydrogen bounded to amide I group of another ceramide forming a tight network of hydrogen bonding at the head of ceramide. This hydrogen bonding lends strength and stability to lipids bilayers and thus imparts barrier property to stratum corneum (Jain et al. Citation2002). When skin is treated with terpenes, ceramides may get loosened because of competitive hydrogen bonding leading to breaking of hydrogen bond network at the head of ceramides due to entry of terpenes into the lipid bilayers of stratum corneum. Peaks at 1650 and 1550 cm −1 are sensitive to shift from their position depending on the changes in protein upon application of enhancers as shown in the control skin spectrum ().
Treatment with terpenes resulted shifting of peak to lower wave number () which suggests breaking of hydrogen bonds by terpenes. In our study, anethole treatment caused breaking the hydrogen bonds, which is further confirmed by the hypothesis that breaking or loosening of hydrogen bond network between ceramides head groups is caused by oxygen containing terpenes such as anethole, menthone, and eugenol (Jain et al. Citation2002).
Conclusions
In this study, we demonstrated that valsartan was penetrated into rat skin quickly. Anethole enhanced the permeation of valsartan significantly (p < 0.05). Anethole was the most effective terpene enhancer for the permeation of the drug followed by menthone. Eugenol was the least effective terpene enhancer. The lipophilicity of the enhancers seems an important factor in promoting penetration of valsartan which is a highly lipophilic drug, through the skin. Taken together, these results indicate that anethole is effective enhancer of the flux of valsartan, and the transdermal system containing anethole has potential application as a new transdermal therapeutic system for the management of hypertension.
Declaration of interest
All authors have approved the final manuscript and the authors declare that they have no conflicts of interest to disclose.
References
- Ahad A, Al-Jenoobi FI, Al-Mohizea AM, Aqil M, Kohli K. 2013a. Transdermal delivery of calcium channel blockers for hypertension. Expert Opin Drug Deliv. 10:1137–1153.
- Ahad A, Al-Mohizea AM, Al-Jenoobi FI, Aqil M. 2014a. Transdermal delivery of angiotensin II receptor blockers (ARBs), angiotensin-converting enzyme inhibitors (ACEIs) and others for management of hypertension. Drug Deliv. [Epub ahead of print]. doi: 10.3109/10717544.2014.942444.
- Ahad A, Al-Saleh AA, Akhtar N, Al-Mohizea AM, Al-Jenoobi FI. 2015. Transdermal delivery of antidiabetic drugs: formulation and delivery strategies. Drug Discov Today. [Epub ahead of print]. doi: 10.1016/j.drudis.2015.06.002.
- Ahad A, Aqil M, Ali A. 2014b. Investigation of antihypertensive activity of carbopol valsartan transdermal gel containing 1,8-cineole. Int J Biol Macromol. 64:144–149.
- Ahad A, Aqil M, Kohli K, Chaudhary H, Sultana Y, Mujeeb M, Talegaonkar S. 2009. Chemical penetration enhancers: a patent review. Expert Opin Ther Pat. 19:969–988.
- Ahad A, Aqil M, Kohli K, Sultana Y, Mujeeb M, Ali A. 2011a. Interactions between novel terpenes and main components of rat and human skin: mechanistic view for transdermal delivery of propranolol hydrochloride. Curr Drug Deliv. 8:213–224.
- Ahad A, Aqil M, Kohli K, Sultana Y, Mujeeb M, Ali A. 2011b. Role of novel terpenes in transcutaneous permeation of valsartan: effectiveness and mechanism of action. Drug Dev Ind Pharm. 37:583–596.
- Ahad A, Aqil M, Kohli K, Sultana Y, Mujeeb M, Ali A. 2012. Formulation and optimization of nanotransfersomes using experimental design technique for accentuated transdermal delivery of valsartan. Nanomedicine. 8:237–249.
- Ahad A, Aqil M, Kohli K, Sultana Y, Mujeeb M. 2013b. Enhanced transdermal delivery of an anti-hypertensive agent via nanoethosomes: statistical optimization, characterization and pharmacokinetic assessment. Int J Pharm. 443:26–38.
- Ahad A, Aqil M, Kohli K, Sultana Y, Mujeeb M. 2014c. Design, formulation and optimization of valsartan transdermal gel containing iso-eucalyptol as novel permeation enhancer: preclinical assessment of pharmacokinetics in Wistar albino rats. Expert Opin Drug Deliv. 11:1149–1162.
- Akbari J, Saeedi M, Farzin D, Morteza-Semnani K, Esmaili Z. 2015. Transdermal absorption enhancing effect of the essential oil of Rosmarinus officinalis on percutaneous absorption of Na diclofenac from topical gel. Pharm Biol. 53:1442–1447.
- Amin S, Kohli K, Khar RK, Mir SR, Pillai KK. 2008. Mechanism of in vitro percutaneous absorption enhancement of carvedilol by penetration enhancers. Pharm Dev Technol. 13:533–539.
- Amnuaikit C, Ikeuchi I, Ogawara K, Higaki K, Kimura T. 2005. Skin permeation of propranolol from polymeric film containing terpene enhancers for transdermal use. Int J Pharm. 289:167–178.
- Aqil M, Ahad A, Sultana Y, Ali A. 2007. Status of terpenes as skin penetration enhancers. Drug Discov Today. 12:1061–1067.
- El-Kattan AF, Asbill CS, Michniak BB. 2000. The effect of terpene enhancer lipophilicity on the percutaneous permeation of hydrocortisone formulated in HPMC gel systems. Int J Pharm. 198:179–189.
- Fang C, Liu Y, Ye X, Rong ZX, Feng XM, Jiang CB, Chen HZ. 2008. Synergistically enhanced transdermal permeation and topical analgesia of tetracaine gel containing menthol and ethanol in experimental and clinical studies. Eur J Pharm Biopharm. 68:735–740.
- Geinoz S, Guy RH, Testa B, Carrupt PA. 2004. Quantitative structure-permeation relationships (QSPeRs) to predict skin permeation: a critical evaluation. Pharm Res. 21:83–92.
- Ghafourian T, Zandasrar P, Hamishekar H, Nokhodchi A. 2004. The effect of penetration enhancers on drug delivery through skin: a QSAR study. J Control Release. 99:113–125.
- Gumming KI, Winfield AJ. 1994. In vitro evaluation of a series of sodium carboxylates as dermal penetration enhancers. Int J Pharmaceut. 108:141–148.
- Hassan N, Ahad A, Ali M, Ali J. 2010. Chemical permeation enhancers for transbuccal drug delivery. Expert Opin Drug Deliv. 7:97–112.
- Jain AK, Thomas NS, Panchagnula R. 2002. Transdermal drug delivery of imipramine hydrochloride. I. Effect of terpenes. J Control Release. 79:93–101.
- Kang L, Yap CW, Lim PF, Chen YZ, Ho PC, Chan YW, Wong GP, Chan SY. 2007. Formulation development of transdermal dosage forms: quantitative structure–activity relationship model for predicting activities of terpenes that enhance drug penetration through human skin. J Control Release. 120:211–219.
- Kararli TT, Kirchhoff CF, Penzotti SC. 1995. Enhancement of transdermal transport of azidothymidine (AZT) with novel terpene and terpene-like enhancers: in vivo–in vitro correlations. J Control Release. 34:43–51.
- Krishnaiah YS, Al-Saidan SM, Chandrasekhar DV, Satyanarayana V. 2005. Bioavailability of nerodilol-based transdermal therapeutic system of nicorandil in human volunteers. J Control Release. 106:111–122.
- Krishnaiah YS, Al-Saidan SM, Jayaram B. 2006. Effect of nerodilol, carvone and anethole on the in vitro transdermal delivery of selegiline hydrochloride. Pharmazie. 61:46–53.
- Krishnaiah YS, Satyanarayana V, Bhaskar P. 2002a. Influence of limonene on the bioavailability of nicardipine hydrochloride from membrane-moderated transdermal therapeutic systems in human volunteers. Int J Pharm. 247:91–102.
- Krishnaiah YS, Satyanarayana V, Bhaskar P. 2003. Influence of menthol and pressure-sensitive adhesives on the in vivo performance of membrane-moderated transdermal therapeutic system of nicardipine hydrochloride in human volunteers. Eur J Pharm Biopharm. 55:329–337.
- Krishnaiah YS, Satyanarayana V, Karthikeyan RS. 2002b. Effect of the solvent system on the in vitro permeability of nicardipine hydrochloride through excised rat epidermis. J Pharm Pharm Sci. 5:123–130.
- Kunta JR, Goskonda VR, Brotherton HO, Khan MA, Reddy IK. 1997. Effect of menthol and related terpenes on the percutaneous absorption of propranolol across excised hairless mouse skin. J Pharm Sci. 86:1369–1373.
- Lee PJ, Langer R, Shastri VP. 2005. Role of n-methyl pyrrolidone in the enhancement of aqueous phase transdermal transport. J Pharm Sci. 94:912–917.
- Levison KK, Takayama K, Isowa K, Okabe K, Nagai T. 1994. Formulation optimization of indomethacin gels containing a combination of three kinds of cyclic monoterpenes as percutaneous penetration enhancers. J Pharm Sci. 83:1367–1372.
- Manosroi A, Jantrawut P, Akazawa H, Akihisa T, Manosroi W, Manosroi J. 2011a. Transdermal absorption enhancement of gel containing elastic niosomes loaded with gallic acid from Terminalia chebula galls. Pharm Biol. 49:553–562.
- Manosroi A, Jantrawut P, Akihisa T, Manosroi W, Manosroi J. 2011b. In vitro and in vivo skin anti-aging evaluation of gel containing niosomes loaded with a semi-purified fraction containing gallic acid from Terminalia chebula galls. Pharm Biol. 49:1190–1203.
- Moghimi HR, Makhmalzadeh BS, Manafi A. 2009. Enhancement effect of terpenes on silver sulphadiazine permeation through third-degree burn eschar. Burns. 35:1165–1170.
- Mura S, Manconi M, Sinico C, Valenti D, Fadda AM. 2009. Penetration enhancer-containing vesicles (PEVs) as carriers for cutaneous delivery of minoxidil. Int J Pharm. 380:72–79.
- Narishetty ST, Panchagnula R. 2004a. Transdermal delivery of zidovudine: effect of terpenes and their mechanism of action. J Control Release. 95:367–379.
- Narishetty ST, Panchagnula R. 2004b. Transdermal delivery system for zidovudine: in vitro, ex vivo and in vivo evaluation. Biopharm Drug Dispos. 25:9–20.
- Nishida N, Taniyama K, Sawabe T, Manome Y. 2010. Development and evaluation of a monolithic drug-in-adhesive patch for valsartan. Int J Pharm. 402:103–109.
- Okabe H, Takayama K, Ogura A, Nagai T. 1989. Effect of limonene and related compounds on the percutaneous absorption of indomethacin. Drug Des Deliv. 4:313–321.
- Panchagnula R, Salve PS, Thomas NS, Jain AK, Ramarao P. 2001. Transdermal delivery of naloxone: effect of water, propylene glycol, ethanol and their binary combinations on permeation through rat skin. Int J Pharm. 219:95–105.
- Rhee YS, Chang SY, Park CW, Chi SC, Park ES. 2008. Optimization of ibuprofen gel formulations using experimental design technique for enhanced transdermal penetration. Int J Pharm. 364:14–20.
- Rizwan M, Aqil M, Ahad A, Sultana Y, Ali MM. 2008. Transdermal delivery of valsartan: I. Effect of various terpenes. Drug Dev Ind Pharm. 34:618–626.
- Roberts MS. 1997. Targeted drug delivery to the skin and deeper tissues: role of physiology, solute structure and disease. Clin Exp Pharmacol Physiol. 24:874–879.
- Sapra B, Jain S, Tiwary AK. 2008. Percutaneous permeation enhancement by terpenes: mechanistic view. AAPS J. 10:120–132.
- Shakeel F, Baboota S, Ahuja A, Ali J, Shafiq S. 2008. Skin permeation mechanism and bioavailability enhancement of celecoxib from transdermally applied nanoemulsion. J Nanobiotechnol. 6:8.
- Shirakura O, Ohshima A, Tsunemi S, Ishimaru S, Sakaguchi T, Takayama K, Nagai T. 1995. Synergistic effect of d-limonene and ethanol on the transdermal permeation of NB-818. Drug Dev Ind Pharm. 21:411–425.
- Tatar S, Saglik S. 2002. Comparison of UV- and second derivative-spectrophotometric and LC methods for the determination of valsartan in pharmaceutical formulation. J Pharm Biomed Anal. 30:371–375.
- Tenjarla SN, Kasina R, Puranajoti P, Omar MS, Harris WT. 1999. Synthesis and evaluation of N-acetylprolinate esters – novel skin penetration enhancers. Int J Pharm. 192:147–158.
- Thomas NS, Panchagnula R. 2003. Combination strategies to enhance transdermal permeation of zidovudine (AZT). Pharmazie. 58:895–898.
- Vaddi HK, Ho PC, Chan SY. 2002a. Terpenes in propylene glycol as skin-penetration enhancers: permeation and partition of haloperidol, Fourier transform infrared spectroscopy, and differential scanning calorimetry. J Pharm Sci. 91:1639–1651.
- Vaddi HK, Ho PC, Chan YW, Chan SY. 2002b. Terpenes in ethanol: haloperidol permeation and partition through human skin and stratum corneum changes. J Control Release. 81:121–133.
- Vashistha I, Ahad A, Aqil M, Agarwala SP. 2014. Investigating the potential of essential oils as penetration enhancer for transdermal losartan delivery: effectiveness and mechanism of action. Asian J Pharm Sci. 9:260–267.
- Williams AC, Barry BW. 2004. Penetration enhancers. Adv Drug Deliv Rev. 56:603–618.
- Yamane MA, Williams AC, Barry BW. 1995. Terpene penetration enhancers in propylene glycol/water co-solvent systems: effectiveness and mechanism of action. J Pharm Pharmacol. 47:978–989.
- Yamashita F, Hashida M. 2003. Mechanistic and empirical modeling of skin permeation of drugs. Adv Drug Deliv Rev. 55:1185–1199.
- Zhao K, Singh J. 1998. Mechanisms of percutaneous absorption of tamoxifen by terpenes: eugenol, d-limonene and menthone. J Control Release. 55:253–260.