Abstract
Context: Overdose of acetaminophen (APAP) is common in humans and is often associated with hepatic damage. Withania somnifera (L.) Dunal (Solanaceae) shows multiple pharmacological activities including antioxidant and anti-inflammatory potential.
Objective: To evaluate the possible mechanism of hepatoprotective activity of withanolide-rich fraction (WRF) isolated from a methanolic extract of Withania somnifera roots.
Materials and methods: Hepatotoxicity was induced by oral administration of APAP (750 mg/kg, p.o.) for 14 d. The control group received the vehicle. APAP-treated animals were given either silymarin (25 mg/kg) or graded doses of WRF (50, 100 and 200mg/kg) 2 h prior to APAP administration. Animals were killed on 15th day and blood and liver tissue samples were collected for the further analysis.
Results: In WRF-treated group, there was significant and dose-dependent (p < 0.01 and p < 0.001) decrease in serum bilirubin, ALP, AST and ALT levels with significant and dose-dependent (p < 0.01 and p < 0.001) increase in hepatic SOD, GSH and total antioxidant capacity. The level of MDA and NO decreased significantly (p < 0.01) by WRF treatment. Up-regulated mRNA expression of TNF-α, IL-1β, COX-II and iNOS was significantly down-regulated (p < 0.001) by WRF. Histological alternations induced by APAP in liver were restored to near normality by WRF pretreatment.
Conclusion: WRF may exert its hepatoprotective action by alleviating inflammatory and oxido-nitrosative stress via inhibition of TNF-α, IL-1β, COX-II and iNOS.
Introduction
Use of acetaminophen (APAP) for the therapeutic purpose was approved by the U.S. Food and Drug Administration in 1955 (Ahmad et al. Citation2012). APAP, also commonly known as paracetamol is a commonly used analgesic and an antipyretic drug which is regarded as safe and efficacious compared to aspirin especially for pediatric treatment (Anderson et al. Citation1999). However, an overdose of APAP causes severe hepatotoxicity and necrosis in both humans and experimental animals (Katyare & Satav Citation1991; Larson et al. Citation2005). The action of APAP as an analgesic drug is either through inhibition of prostaglandin synthesis or active metabolite p-aminophenol. p-Aminophenol is conjugated to arachidonic acid by an amide linkage to form the active archidonic acid metabolite: AM404; AM404 exerts therapeutic effect through cannabinoid receptors (Ottani et al. Citation2006). APAP is primarily metabolized in the liver by glucuronidation and sulfation. However, a small proportion undergoes cytochrome P450 (CYP450)-mediated bioactivation to form highly toxic metabolite N-acetyl-p-benzoquinoimine (NAPQI), which is rapidly quenched by glutathione (GSH) (James et al. Citation2003). After an overdose of APAP, elevated levels of NAPQI are generated which cause the depletion of GSH. Subsequent to covalent adduct formation with of NAPQI, oxidative stress, lipid peroxidation and inhibition of plasma membrane Ca2+-ATPase activity which disrupts Ca2+ homeostasis lead to necrosis (Moore et al. Citation1985). Although the precise biochemical mechanism of cell necrosis is not fully understood, it is believed that there is simultaneous involvement of GSH depletion, lipid peroxidation and oxidative stress (Randle et al. Citation2008; Honmore et al. Citation2015). It has been reported that 48% of acetaminophen-related cases were associated with overdose of APAP which leads to hepatic damage and compromised antioxidant metabolism in the kidneys (Ghosh & Sil Citation2007).
An overdose of APAP can cause acute liver failure in humans as well as in experimental animals (Ghosh & Sil Citation2007). Animal model played a vital role in the development of new chemical entities for the treatment of hepatotoxicity (Kandhare et al. Citation2011b). Silymarin, a known hepatoprotective agent, is a flavonoid obtained from the plant Silybum marianum (L.) Gaertn. (Asteraceae), also known as milk thistle. Silymarin comprises three isomers: silybinin, silydianin and silychristin, of which silybinin is quantitatively the most important (Kshirsagar et al. Citation2009). Silybinin has been shown to inhibit the function of Kupffer cells which are well recognized for their fibrogenic mediator activity (Jeong et al. Citation2005). In addition, silymarin stabilizes the lipid structures in the hepatocellular membranes which may apply in general to all cell membranes (Jeong et al. Citation2005).
The liver is a vital organ which regulates several important metabolic functions and is also responsible for maintaining homeostasis of the body (Kandhare et al. Citation2015a). A number of chemical agents and drugs which are used routinely produce cellular as well as metabolic liver damage (Gosavi et al. Citation2012a; Adil et al. Citation2014). The traditional system of medicine has a major role in the treatment of liver ailments (Mohan Citation2002). In the recent years, importance is being given to Ayurvedic polyherbal formulations due to their effective therapeutic action and minimal side effects. Withania somnifera (L.) Dunal (Solanaceae), commonly known as Ashwagandha, winter cherry and Indian ginseng, is considered to be one of the most important medicinal plant of the medicinal system in Indian Ayurveda for over 3000 years (Mishra et al. Citation2000; Kulkarni et al. Citation2008). W. somnifera has several pharmacological activities, viz., antioxidant, antimicrobial, antifungal, adaptogenic, cardioprotective, anticancer, neuroprotective, anticonvulsant, immunomodulatory, apoptic, diuretic, hepatoprotective and anti-inflammatory. These medicinal properties are mainly attributed to withanolides present in the roots of W. somnifera (Kulkarni et al. Citation2008). Withanolides are steroidal lactones and about 35 withanolides have been isolated from the roots of W. somnifera and quantified (Mishra et al. Citation2000; Kulkarni et al. Citation2008; Devkar et al. Citation2012, Citation2013). The multi-component extracts show better medicinal efficacy than purified components, i.e., the strength of herbal medicine increased with the mixture of secondary metabolites instead of a single component (Patwardhan & Mashelkar Citation2009).
Therefore, the aim of present investigation was to evaluate the hepatoprotective potential of withanolide-rich fraction (WRF) extracted from roots of W. somnifera against APAP-induced toxicity in laboratory animals by using various biochemical, molecular and histological parameters.
Materials and methods
Chemicals
1,1′,3,3′-Tetraethoxypropane, crystalline beef liver catalase, reduced glutathione, 5,5′-dithiobis 2-nitrobenzoic acid (DTNB), Folin-phenol reagent and silymarin were purchased from S.D. Fine Chemicals, Mumbai, India. Sulfanilamide, naphthylamine diamine and other analytical grade reagents and solvents were obtained from Loba Chemi Pvt. Ltd, Mumbai, India. RT-PCR was performed according to instructions provided by the manufacturer (Biotools B and M Labs, Spain).
Preparation of drug
Isolation and characterization of WRF was carried out according to the method described earlier (Devkar et al. Citation2012, Citation2013). Briefly, dried and pulverized roots of W. somnifera were subjected to Soxhlet extraction using methanol as the solvent. The solvent was evaporated, and the residue was hydrolyzed with 50% (v/v) methanol containing 1.2 M HCl. The hydrolysate was filtered using Whatman filter paper No. 1. The filtrate was extracted by vigorously shaking with freshly prepared chloroform-methanol (3:1 v/v) mixture. The mixture was allowed to settle down; chloroform layer is completely separated and evaporates to dryness to obtain the WRF. WRF is subjected to HPTLC using dichloromethane:toluene:methanol:acetone:diethyl ether (6.5:7:4:1.5:1 v/v) solvent system. Quantification of major withanolides was carried out by comparing with authentic samples. Antioxidant characterization of individual components was derived using TLC-DPPH based assay (Devkar et al. Citation2013).
Animals
Adult male albino rats of Wistar strain (8–10 week old) were obtained from the National Institute of Biosciences, Pune (India). The animals were maintained at 24 ± 1 °C with relative humidity of 45–55% and 12:12 h dark/light cycle. The animals had free access to standard pellet chow (Pranav Agro Industries Ltd., Sangli, India) and water throughout the experimental period. The experimental protocol was approved by the Institutional Animal Ethics Committee (IAEC) of Poona College of Pharmacy, Bharati Vidyapeeth Deemed University, Pune and performed in accordance with the guidelines of Committee for Control and Supervision of Experimentation on Animals (CPCSEA/103/12).
Acute oral toxicity tests for WRF
Acute oral toxicity test was performed for the WRF in male Swiss albino mice according to the OECD guidelines using AOT 425 software.
Experimental design
The animals were randomly divided into six groups of six rats each as given below:
Group I: Normal: The animals were administered a single daily dose of 10 mg/kg of 1% aqueous solution of gum acacia p.o. for 14d.
Group II: APAP-treated: The animals were administered a single daily dose of 10 mg/kg of 1% aqueous solution of gum acacia p.o. for 14 d 2 h before oral administration of APAP suspension (750 mg/kg) for 14 d.
Group III: Silymarin-treated: The animals were administered a single daily dose of 25 mg/kg of 1% aqueous solution of silymarin in distilled water p.o. 2 h before oral administration of APAP suspension (750 mg/kg) for 14 d.
Group IV: WRF (50mg/kg): The animals were administered a single daily dose of 50 mg/kg of 1% aqueous solution of WRF in distilled water p.o. 2 h before oral administration of APAP suspension (750 mg/kg) for 14d.
Group V: WRF (100 mg/kg): The animals were administered a single daily dose of 100 mg/kg of 1% aqueous solution of WRF in distilled water p.o. 2 h before oral administration of APAP suspension (750 mg/kg) for 14d.
Group VI: WRF (200 mg/kg): The animals were administered a single daily dose of 200 mg/kg of 1% aqueous solution of WRF in distilled water p.o. 2 h before oral administration of APAP suspension (750 mg/kg) for 14 d.
The selection of the dose regimen of APAP was according to the previously reported method (Ahmad et al. Citation2012). At the end of the experimental period, i.e. on the 15th day the animals were anesthetized using anesthetic ether and blood was withdrawn by retroorbital puncture and collected in EDTA tubes. After collecting the blood the animals were sacrificed by cervical dislocation, the liver was excised and divided into two portions which were stored at −80 °C. These portions were then used for biochemical estimations, RT-PCR studies and histopathological examination.
Serum analysis
The EDTA tubes were subjected to centrifugation at 7000 rpm using Eppendorf cryocentrifuge (model No. 5810, Germany), at 4 °C to obtain clear serum samples. The levels of albumin, direct bilirubin, total bilirubin, alkaline phosphatase (ALP), aspartate transaminase (AST), alanine transaminase (ALT), lactate dehydrogenase (LDH), triglyceride (TG), cholesterol, low-density lipoprotein (LDL) and high-density lipoprotein (HDL) were measured by using commercially available reagent kits according to the procedures provided by the manufacturer (Accurex Biomedical Pvt. Ltd., Mumbai, India).
Estimation of oxidative stress
20% w/v liver homogenates were prepared in 0.1 M Tris-HCl buffer (pH 7.4) and used for determination of malondialdehyde (MDA), nitric oxide (NO), content of reduced GSH, superoxide dismutase activity (SOD) and total protein according to methods reported previously (Gosavi et al. Citation2012a, Citation2012b; Kandhare et al. Citation2012a, Citation2012b, Citation2013b).
Estimation of total antioxidant capacity (TAC)
Liver homogenates (20% w/v) were prepared using phosphate buffered saline (pH 7.4), and the total antioxidant capacity was determined by using Autolab, PGSTA 101 cyclic voltammeter (Metrohm, Switzerland). A three-electrode system was used for the study. The working electrode: glassy carbon (Autolab GC) 8 mm in diameter was polished before each measurement; platinum wire served as an auxiliary electrode and saturated calomel electrode as the reference electrode. The cell contained 2 mL of phosphate buffered liver homogenate. All cyclic voltammogram measurements were performed in the range −0.2–1.3 V at a scan rate of 400 mV/s. Each sample was analyzed thrice (Momen-Beitollahi et al. Citation2010; Kandhare et al. Citation2015b).
Reverse transcriptase-polymerase chain reaction (RT-PCR) analysis
Total RNA was extracted from liver tissues according to earlier reported methods (Kandhare et al. Citation2012e; Visnagri et al. Citation2014). The polymerase chain reaction mixture was amplified in a DNA thermal cycler (Eppendorf India Ltd, Chennai) by using gene specific primers. The primer sequences for TNF-α, IL-1β, iNOs and COX-II were selected according to the previously reported method (Supplementary file) (Kandhare et al. Citation2014a, Citation2015d; Visnagri et al. Citation2015). PCR products were run on 1% agarose gels, stained with ethidium bromide. The expression of all the genes was assessed by generating densitometry data for band intensities in different sets of experiments and was generated by analyzing the gel images in the Image J program Version 1.33 (Wayne Rasband, National Institutes of Health (NIH), Bethesda, MD). The band intensities were compared with constitutively expressed β-actin which served as a control for sample loading and integrity. The intensity of mRNAs was standardized against that of the β-actin mRNA from each sample and the results are expressed as PCR-product/β-actin mRNA ratio.
Histological examination
The liver tissue was kept in 10% formalin for 24 h at room temperature and dehydrated by passing through grades of ethyl alcohol (70, 90 and 100%). Following this, the samples were placed in xylene for 1 h with the solvent being replaced three times. The tissue was then fixed in paraffin wax, and sections of 3–5 μm thickness were using a microtone. The samples were stained with hematoxylin and eosin. The specimens were mounted on slides using Distrene Phthalate Xylene (DPX) as mounting medium. Sections were examined under a light microscope for inspection of the histopathological features and infiltration of cells. The changes in histological features were graded according to the previously reported method (Honmore et al. Citation2015) as Grade 0 (not present or very slight); Grade 1 (mild); Grade 2 (moderate); and Grade 3 (severe).
Statistical analysis
The data were analyzed by one-way analysis of variance test (ANOVA) using GraphPad Prism 5.0 software (GraphPad, San Diego, CA). Dunnett’s test was applied for post hoc analysis. The results are given as mean ± SEM. Values of p < 0.05 were considered to be statistically significant.
Results
Characterization of WRF
WRF was prepared essentially by following our previously described method and subjected an analysis by HPTLC and TLC-DPPH methods. The analysis was consistent with our previously reported values (Devkar et al. Citation2013). The contents of withanoside V, withaferin A, 1, 2 deoxywithastramonolide, withanone, withanolide A and withanolide B were 0.86, 12.9, 1.92, 1.52, 5.24 and 4.52 mg/g, respectively. TLC-DPPH analysis showed that withaferin A, withanolide A, withanoside V, withanone and 1, 2 deoxywithastramonolide had antioxidant activity. Additionally unidentified peak 17 showed highest antioxidant activity while peak 16 showed lowest antioxidant activity. Peaks nine and 13 did not possess antioxidant activity (Devkar et al. Citation2013).
Acute toxicity studies
Acute toxicity studies showed no toxic signs and symptoms such as restlessness, respiratory distress, diarrhea, convulsions and coma; WRF was safe up to 2000 mg/kg.
Effects of WRF on serum parameters
APAP treatment resulted in significant decrease (p < 0.01) in serum albumin level with a significant increase (p < 0.001) in the direct bilirubin, total bilirubin, ALP, AST, ALT, and LDH. Treatment with WRF (50, 100 and 200 mg/kg) did not show any significant change in the serum albumin level. However, direct bilirubin and total bilirubin levels decreased significantly (p < 0.01) in the WRF (200 mg/kg)-treated the group as compared to APAP-treated rats. There was significant and dose-dependent (p < 0.01 and p < 0.001) decrease in the ALP, AST, ALT and LDH in the WRF (100 and 200 mg/kg)-treated rats as compared to the APAP-treated group. Treatment with silymarin significantly lowered (p < 0.001) the elevated levels of direct bilirubin, total bilirubin, ALP, AST, ALT and LDH as compared to the APAP-treated rats ().
Table 1. Effect of treatment with withanolide-rich fraction (WRF) on APAP-induced alteration of serum levels of albumin, direct bilirubin, total bilirubin, ALP, AST and ALT in rats.
Effects of WRF on plasma lipid parameters
In APAP-treated rats, TG, cholesterol and LDL levels were significantly elevated (p < 0.001) whereas HDL level decreased significantly (p < 0.001) as compared to normal rats. WRF treatment (100 and 200 mg/kg) significantly and dose-dependently restored (p< 0.01 and p< 0.001) the altered levels of TG, cholesterol, LDL and HDL in a dose-dependent manner. Silymarin-treated rats also showed a significant reduction (p < 0.001) in elevated levels of TG, cholesterol, LDL and restored the altered levels of HDL ().
Table 2. Effect of treatment with withanolide-rich fraction (WRF) on APAP-induced alteration in serum cholesterol, LDH, HDL, LDL and TG in rats.
Effect of WRF on antioxidant parameters
The MDA and NO levels increased significantly (p < 0.01 and p < 0.001) in the APAP-treated rats; the elevated level of MDA and NO decreased significantly (p < 0.05 and (p < 0.01) and dose-dependently following WRF (100 and 200 mg/kg) treatment. Silymarin-treated rats also showed significant (p < 0.05 and p < 0.01) decreased the level of MDA and NO (). There was significant decrease (p < 0.001) in the TAC in APAP-treated rats as compared to normal controls. The decreased levels of TAC showed dose-dependent (p < 0.05 and p < 0.01) increase by WRF treatment () ().
Figure 1. Cyclic voltammograms analysis of rat liver homogenate in phosphate buffer saline. (A) The background (phosphate buffer alone) has been subtracted to estimate the peak anodic current (ip) i.e. total antioxidant potential. (B) Rat liver tissue, the superimposed voltammograms of (1) normal, (2) Silymarin (25 mg/kg)-treated, (3) WRF (200 mg/kg)-treated, (4) WRF (10 mg/kg)-treated, (5) WRF (50 mg/kg)-treated, (6) APAP-treated and (7) blank (phosphate buffer alone).
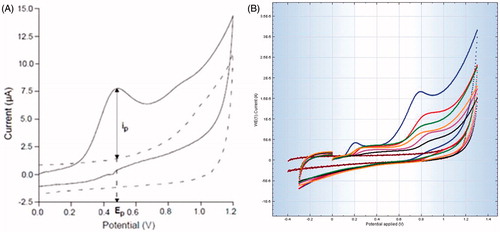
Table 3. Effect of withanolide-rich fraction (WRF) treatment on APAP-induced alteration on antioxidant parameters in rat liver.
There was significant decrease (p < 0.001) in the GSH and SOD levels in the APAP-treated rats as compared to normal controls. The APAP-induced decreased levels of GSH and SOD was significantly (p < 0.01 and p < 0.001) and dose-dependently increased by WRF (100 and 200 mg/kg) treatment. Silymarin-treated rats also showed significantly (p < 0.001) elevated GSH and SOD levels ().
Effect of WRF on TNF-α, IL-1β, iNOS and COX-II mRNA expression
There was significant (p < 0.001) up-regulation in the TNF-α, IL-1β, iNOS and COX-II mRNA expression in APAP-treated rats as compared to normal controls. WRF (100 and 200 mg/kg) treatment significantly and dose-dependently (p < 0.01 and p < 0.001) down-regulated TNF-α and IL-1β mRNA expression in a dose-dependent manner. Up-regulated iNOS and COX-II mRNA expression was significantly (p < 0.001) reduced only after treatment WRS (200 mg/kg). Silymarin treatment showed significantly (p < 0.001) down-regulation in TNF-α, iNOS and COX-II mRNA expression but failed to produce any significant alteration in IL-1β mRNA expression ().
Figure 2. Effect of WRF treatment on APAP-induced alteration in reverse transcriptase analysis of mRNA expression of TNF-α (A), IL-1β (B), iNOs (C) and COX-II (D).
Data are expressed as mean ± SEM and analyzed by one-way ANOVA followed by Dunnett’s test. *p < 0.05, **p < 0.01 and ***p < 0.001 as compared to control group and #p < 0.05, ##p < 0.01, ###p < 0.001 as compared to normal group.
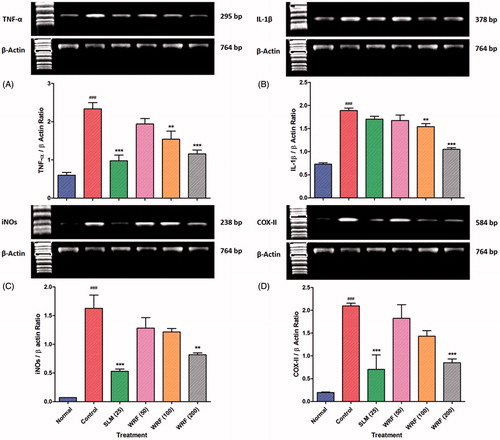
Histopathological observations
The presence of the normal central vein with portal triads without any evidence of necrosis (grade 0) and inflammatory infiltration (grade 0) reflected the normal architecture of liver tissue (). There was no evidence of vesicular or fatty changes (grade 0). However, the presence of a mild degree of congestion (grade 1) was noted. Histopathological studies of the liver tissue from APAP-treated rats showed the presence of centrilobular necrosis (grade 3) with inflammatory infiltration (grade 3) around the centrilobular veins with periportal degeneration. It showed the focal hyaline degeneration of hepatocytes along with macrovesicular fatty changes (grade 4) (). Liver from silymarin-treated rats showed mild necrosis (grade 2) and a scant number of inflammatory cells mainly microphages (grade 1) were seen around centrilobular veins. There was no evidence of periportal degeneration. However; it showed vacuolization (grade 1) with macrovesicular fatty changes (grade 1) (). Liver from WRF (50 and 100 mg/kg)-treated rats showed necrosis (grade 3 and grade 2, respectively) and congestion around central vein (grade 2). Inflammatory cells mainly macrophages and plasma cells (grade 3) were seen along with macrovesicular fatty changes (grade 3 and grade 1, respectively). It also showed focal hepatic hyaline degeneration (). Histology of liver tissue from WRF (200 mg/kg)-treated rats showed normal architecture. Hepatocytes were unremarkable; central veins and portal triads were normal. However, it showed mild necrosis (grade 1) without any infiltration of inflammatory cells. There was no evidence of macrovesicular fatty changes or congestion () ().
Figure 3. Effect of WRF treatment on APAP-induced pathological alteration in rat liver. Photomicrograph of sections of liver of normal (A), APAP-treated (B), Silymarin (25 mg/kg)-treated (C), WRF (50 mg/kg)-treated (D), WRF (100 mg/kg)-treated (E) and WRF (200 mg/kg)-treated (E) rats. Inflammatory infiltration (red arrow), congestion (yellow arrow), pyknosis (green arrow) and necrosis (black arrow). H&E staining at 40 X and 100 X (inset).
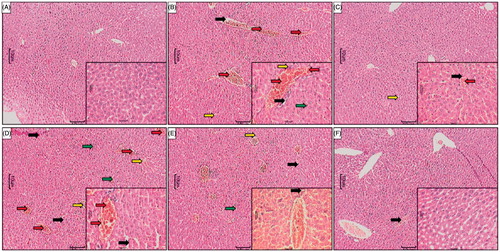
Table 4. Effect of withanolide-rich fraction (WRF) on APAP-induced histology alterations in rat liver.
Discussion
There are more than 28 billion doses of products containing acetaminophen that were purchased consumers over the last decade (Kaufman et al. Citation2002). It has been reported that 48% of acetaminophen-related cases were associated with overdose of APAP which leads to hepatic damage (Katyare & Satav Citation1991; Larson et al. Citation2005). An array of pharmacological activities of W. somnifera have been reported by researcher including hepatoprotective activity (Malik et al. Citation2013). However, they failed to reveal its exact mechanism of action. Therefore, present investigations were undertaken to evaluate the possible mechanism of action behind hepatoprotective properties of WRF in APAP-induced hepatotoxicity in a rat model. In WRF, concentration of WF, A is higher than other withanolides which are followed by WN A while WN B (Devkar et al. Citation2015). It also reported that WF A possesses highest antioxidant activity followed by WN B, WN A, WS-V, 1, 2 DWM and WNN respectively (Devkar et al. Citation2013).
The estimation of enzymes in the serum is a useful quantitative marker of the extent and type of hepatocellular damage. ALT and AST are important endogenous diagnostic biomarkers for hepatic toxicity. The increase in the serum AST and ALT levels has been attributed to the damaged structural integrity of the liver (Ahmad et al. Citation2012). Whereas, LDH is present in the cell cytoplasm and insult to cell caused significant leakage of LDH from cell cytoplasm into the serum (Visnagri et al. Citation2012; Kumar et al. Citation2014; Badole et al. Citation2015). Moreover, cholestasis with increased biliary pressure resulted in the release of ALP into bile canaliculi cell lining. In this study, a significant elevation in the marker enzymes viz. AST, ALT, ALP and LDH, and levels of total bilirubin and direct bilirubin in serum were noted following APAP administration. The increased levels of these serum biomarkers in APAP-intoxicated rats indicate a deterioration of the hepatic structure/function due to liver membrane damage (Jaeschke et al. Citation2003). It has been reported that aqueous extract of roots of W. somnifera (500 mg/kg) attenuated the increased activities of liver markers enzymes (Malik et al. Citation2013). It has been suggested that the prevention of the leakage of intracellular enzymes in serum of APAP-intoxicated rats might be due to the membrane stabilizing activity of WRF of W. somnifera (Ganguly et al. Citation2009).
Reduced serum albumin is a hallmark of liver diseases. It has been well reported that decreased serum albumin is associated with elevated level of β- and γ-globulins via production of IgG and IgM. Furthermore, increased levels of serum bilirubin indicate impaired capacity of the liver to store bilirubin (Jaeschke et al. Citation2003; Ganguly et al. Citation2009). Bilirubin transported by liver parenchyma cells to form conjugates with glucoronic acid in the presence of enzyme glucuronyl-transferase which further excreted into bile via the process of glucuronidation. Hepatic parenchymal cells damage leads to elevated serum bilirubin (total as well as direct) level in APAP-induced toxicity. In these studies administration of WRF significantly inhibited APAP-induced alterations in serum albumin and bilirubin levels.
It has been well documented that significant alterations occurred in lipoprotein and cholesterol metabolism after APAP intoxication (Kaushal et al. Citation1999; Malik et al. Citation2013). With increased availability of free fatty acids, the triglycerides levels were significantly increased in APAP-induced toxicity which leads to decreased hepatic release of lipoprotein and increased esterification of free fatty acids (Malik et al. Citation2013). Histological examination of the liver tissue from APAP-treated rats showed macro-vesicular fatty changes (grade 4) () this also supports the above findings. Treatment with WRF significantly reduced cholesterol and triglyceride levels which correlated with histopathological findings in which liver tissue from WRF-treated rats showed a reduction in macro-vesicular fatty changes ().
It has been reported that the highly reactive intermediate of APAP metabolism NAPQI has a great affinity for GSH which causes depletion of cellular GSH leading to damage to the liver cells due increased of reactive oxygen species (ROS) (Mitchell et al. Citation1973). SOD plays an important role in eliminating the superoxide radicals (Ghule et al. Citation2015; Kandhare et al. Citation2012b, Citation2012c, Citation2016). GSH is a non-enzymatic biological antioxidant which plays an important role in quenching of free radical species such as hydrogen peroxide, superoxide and alkoxy radicals (Kandhare et al. Citation2014b; Visnagri et al. Citation2014; Ketkar et al. Citation2015). Decreased levels of GSH and SOD correlates with the toxic effects of ROS produced by APAP, which is consistent with previously reported observations, treatment with WRF caused elevation of GSH and SOD level indicating protection from APAP-induced liver damage (Olaleye & Rocha Citation2008).
Membrane lipid peroxidation (in terms of MDA) reflects the damage to the cellular membranes structure via destruction of the double bonds in the unsaturated fatty acids and is considered to be a reliable marker of oxidative stress (Kandhare et al. Citation2012d Raygude et al. Citation2012; Patil et al. Citation2015; Sarkar et al. Citation2015). It is evident from the reduction of antioxidant status of APAP intoxicated rats (Malik et al. Citation2013). In this investigation increase in the MDA level was noted in the APAP-treated rats. Administration of WRF significantly lowered the elevated level of MDA, which corroborates the results of earlier reports for W. somnifera root powder and aqueous extracts suggesting its protective role in APAP-mediated liver injury (Malik et al. Citation2013).
Elevated oxidative stress caused the release of pro-inflammatory mediators such as NO via inducible nitric oxide synthase in APAP intoxicated liver which results in cellular dysfunction (Ahmad et al. Citation2012). Results of the present investigations are consistent with the finding of the previous study where administration of APAP significantly increased NO level in liver (Ahmad et al. Citation2012). An effort has been made to improve hemodynamic properties of the liver by inhibiting the hepatic microvascular constriction by administration of compounds with NO scavenging or nitric oxide synthase (NOS) inhibitors (Malik et al. Citation2013). Treatment with WRF significantly reduced the elevated level of NO suggesting its protective role in APAP-induced hepatotoxicity.
The anodic peak potential (Ep) of the anodic peak current wave (ip) characterizes the reducing power of antioxidants and provides information on the ability of antioxidants to donate electrons ip corresponds to the concentration of antioxidants in terms of total antioxidant capacity (TAC). Cyclic voltammeter (CV) use to determine the TAC in plasma or other biological fluids or tissue homogenates (Momen-Beitollahi et al. Citation2010). In this investigation, we used CV for the first time to measure the TAC of liver in APAP-treated and WRF-treated rats. The potential at the maximum of anodic wave reflects the reducing ability of antioxidants present in the liver tissue. We noted a decrease in antioxidant levels in APAP-intoxicated rats as compared to treated rats; the CV analysis also showed that APAP caused a significant decrease in liver TAC. The elevated level of oxidative stress in APAP-treated rats correlates well with the results of CV where TAC of liver tissue decreased significantly.
It has been reported that NF-κB (nuclear transcription factor-κB) plays a decisive role in the regulation of various gene expressions such as IL-1β, TNF-α, and COX-II (Sarkate et al. Citation2015; Kandhare et al. Citation2015c; Honmore et al. Citation2016). These genes play a critical role in tumorigenesis, various autoimmune diseases, and inflammation (Visnagri et al. Citation2013). In vitro studies carried out in murine J774.2 monocytes and macrophages showed that APAP caused significant activation of NF-κB, caused the release of pro-inflammatory mediators TNF-α and IL-1β and thus elevated oxidative stress (Al-Belooshi et al. Citation2010). It has been reported that APAP-induced hepatotoxicity is associated with elevated levels of pro-inflammatory cytokines such as TNF-α and IL-1β (Tilg et al. Citation1992). Cytokines are associated with mediation of various inflammatory conditions. In an array of diseases, TNF-α is pro-inflammatory cytokine which in turn triggers a cascade of interleukins like IL-1 β, IL-6 and IL-8 (Kandhare et al. Citation2011a, Citation2013a; Aswar et al. Citation2015). IL-1 β which is synthesized as 31 kDa precursor peptides and converted into mature cytokine of 17 kDa triggers inflammatory response resulting in tissue necrosis (Ahmad et al. Citation2012). Results of this investigations are in accordance with the findings of previous investigators (Ahmad et al. Citation2012). Treatment with WRF significantly lowered the elevated oxidative stress and thus, in turn, down-regulated expression of pro-inflammatory cytokines (TNF-α and IL-1β). The results of these studies are in agreement with findings of previous investigators where administration of an alcoholic extract of W. somnifera showed inhibition of the cytokines activity in mice via down-regulation of NF-κB (Grover et al. Citation2010).
NF-kB has been reported to regulate the gene expression and subsequent mRNA translation of iNOS. Various studies have demonstrated that APAP intoxication leads to up-regulation of iNOS protein and nitric oxide production in hepatocytes (Ahmad et al. Citation2012). In this investigation, we noted upregulation of NF-kB, iNOS and nitric oxide production in the APAP-treated group. WRF administration inhibited iNOS expression and nitric oxide production, thereby alleviating hepatic inflammation and damage. Inflammatory insult by the APAP caused overproduction of prostaglandins controlled by the release of rate-limiting enzyme, cyclooxygenase-2 (COX-II) (Ahmad et al. Citation2012). Our data show over expression of COX-II synthesis, which is up-regulated by inflammatory stimuli or lipid hyperoxidation induced synthesis of PG, which causes liver injury. Treatment with WRF significantly down-regulated COX-II expression and thus reduced the inflammation, confirming the anti-inflammatory activity of WRF. Earlier reports state that leaf extract W. somnifera and withaferine A inhibited the expression of inflammation markers TNF- α, NF-κB, COX-II and IKKβ (Grover et al. Citation2010). We observed similar results in our present studies. Based on the results of our present studies we suggest that WRF down-regulates NF-κB-regulated gene products such as COX-II and iNOS induced by TNF-α and IL-1β activation.
Conclusion
These studies we conclude that the WRF exercises its hepatoprotective effect in APAP-treated rats through its antioxidant potential. The results also suggest that cyclic voltammeter may be an alternative rapid method for determining loss of antioxidant as a measure of toxicity. The findings of these studies also suggest that the anti-inflammatory and anti-oxidant activities of WRF of W. somnifera are possibly mediated through suppression of the TNF-α, IL-1β, COX-II and iNOS to exert its hepatoprotective effect ().
Figure 4. Scheme showing the proposed mechanisms of hepatoprotective activity of WRF. Toxic exposure could induce oxidative stress in the liver due to its metabolization into highly reactive free radicals. Oxidative stress triggers the IL-1β and TNF-α release from Kupffer cells and injured hepatocytes, which further activates NF-kB, allowing its nuclear translocation. Consequently, NF-kB stimulates the expression of iNOS and COX-II at the level of transcription, translation, and the enzyme level. The final products of iNOS and COX-II, NO- and prostaglandins, respectively, contributes to nitrosative stress and, on the other hand, initiate the cascade of the inflammatory response in the injured liver. Inflammation, in turn, is associated with the release of highly reactive oxygen and nitrogen species from inflammatory cells, further exacerbating oxidative and nitrosative stress. WRF prevents oxidative damage, as indicated by the decrease in lipid peroxidation, and improves the antioxidant status. Furthermore, WRF suppresses the inflammatory response by down-regulating the pro-inflammatory cascade initiated by TNF-α and IL-1β and attenuates nitrosative stress by the iNOS inhibition.
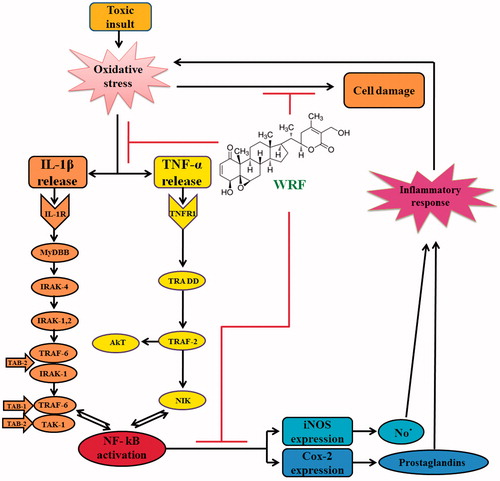
Acknowledgements
We thank Dr. Shivajirao Kadam, Vice Chancellor, Bharati Vidyapeeth Deemed University and Dr. K. R. Mahadik, Principal, Poona College of Pharmacy for the keen interest and providing the necessary facilities to carry out the study. Dr. M. V. Hegde thankfully acknowledges the award of Research Grant by Indian Council of Agriculture Research (ICAR) under the National Agriculture Innovation Project (NAIP).
Disclosure statement
The authors report no declarations of interest.
References
- Adil M, Visnagri A, Kumar VS, Kandhare AD, Ghosh P, Bodhankar SL. 2014. Protective effect of naringin on sodium arsenite induced testicular toxicity via modulation of biochernical perturbations in experimental rats. Pharmacologia. 5:222–234.
- Ahmad ST, Arjumand W, Nafees S, Seth A, Ali N, Rashid S, Sultana S. 2012. Hesperidin alleviates acetaminophen induced toxicity in Wistar rats by abrogation of oxidative stress, apoptosis and inflammation. Toxicol Lett. 208:149–161.
- Al-Belooshi T, John A, Al-Otaiba A, Raza H. 2010. Acetaminophen-induced mitochondrial oxidative stress in murine J774. 2 monocyte macrophages. Am J Biomed Sci. 2:142–154.
- Anderson BJ, Holford NH, Armishaw JC, Aicken R. 1999. Predicting concentrations in children presenting with acetaminophen overdose. J Pediatr. 135:290–295.
- Aswar UM, Kandhare AD, Mohan V, Thakurdesai PA. 2015. Anti-allergic effect of intranasal administration of type-A procyanidin polyphenols based standardized extract of cinnamon bark in ovalbumin sensitized BALB/c mice. Phytother Res. 29:423–433.
- Badole SL, Chaudhari SM, Jangam GB, Kandhare AD, Bodhankar SL. 2015. Cardioprotective activity of Pongamia pinnata in streptozotocin-nicotinamide induced diabetic rats. Biomed Res Int. 2015:403291.
- Devkar ST, Badhe YS, Jagtap SD, Hedge MV. 2012. Quantification of major bioactive withanolides in Withania somnifera (Ashwagandha) roots by HPTLC for rapid validation of Ayurvedic products. J Planar Chromatogr Mod TLC. 25:290–294.
- Devkar ST, Jagtap SD, Katyare SS, Hedge MV. 2013. Estimation of antioxidant potential of individual components present in complex mixture of Withania sominfera (Ashwagnadha) root fraction by TLC-DPPH method. J Planar Chromatogr Mod TLC. 27:157:161. JPC433R1
- Devkar ST, Kandhare AD, Sloley BD, Jagtap SD, Lin J, Tam YK, Katyare SS, Bodhankar SL, Hegde MV. 2015. Evaluation of the bioavailability of major withanolides of using an absorption model system. J Adv Pharm Technol Res. 6:159–164.
- Ganguly P, Gupta AK, Majumder UK, Ghosal S. 2009. Hepatoprotective and antioxidant effects of naturally occurring withasteroid metal ion conjugates of Withania somnifera in paracetamol induced hepatotoxicity in rats. Pharmacologyonline. 1:1044–1056.
- Ghosh A, Sil PC. 2007. Anti-oxidative effect of a protein from Cajanus indicus L against acetaminophen-induced hepato-nephro toxicity. J Biochem Mol Biol. 40:1039–1049.
- Ghule AE, Kandhare AD, Jadhav SS, Zanwar AA, Bodhankar SL. 2015. Omega-3-fatty acid adds to the protective effect of flax lignan concentrate in pressure overload-induced myocardial hypertrophy in rats via modulation of oxidative stress and apoptosis. Int Immunopharmacol. 28:751–763.
- Gosavi TP, Ghosh P, Kandhare AD, kumar VS, Adil M, Rajmane AR, Bodhankar SL. 2012a. Therapeutic effect of H. pylori nosode, a homeopathic preparation in healing of chronic H. pylori infected ulcers in laboratory animals. Asian Pac J Trop Dis. 2:S603–S611.
- Gosavi TP, Kandhare AD, Ghosh P, Bodhankar SL. 2012b. Anticonvulsant activity of Argentum metallicum, a homeopathic preparation. Der Pharmacia Lettre. 4:626–637.
- Grover A, Shandilya A, Punetha A, Bisaria VS, Sundar D. 2010. Inhibition of the NEMO/IKKbeta association complex formation, a novel mechanism associated with the NF-kappaB activation suppression by Withania somnifera's key metabolite withaferin A. BMC Genomics. 11:S25.
- Honmore V, Kandhare A, Zanwar AA, Rojatkar S, Bodhankar S, Natu A. 2015. Artemisia pallens alleviates acetaminophen induced toxicity via modulation of endogenous biomarkers. Pharm Biol. 53:571–581.
- Honmore VS, Kandhare AD, Kadam PP, Khedkar VM, Sarkar D, Bodhankar SL, Zanwar AA, Rojatkar SR, Natu AD. 2016. Isolates of Alpinia officinarum Hance as COX-2 inhibitors: Evidence from anti-inflammatory, antioxidant and molecular docking studies. Int Immunopharmacol. 33:8–17.
- Jaeschke H, Knight TR, Bajt ML. 2003. The role of oxidant stress and reactive nitrogen species in acetaminophen hepatotoxicity. Toxicol Lett. 144:279–288.
- James LP, Mayeux PR, Hinson JA. 2003. Acetaminophen-induced hepatotoxicity. Drug Metab Dispos. 31:1499–1506.
- Jeong DH, Lee GP, Jeong WI, Do SH, Yang HJ, Yuan DW, Park HY, Kim KJ, Jeong KS. 2005. Alterations of mast cells and TGF-beta1 on the silymarin treatment for CCl4-induced hepatic fibrosis. World J Gastroenterol. 11:1141–1148.
- Kandhare AD, Alam J, Patil MV, Sinha A, Bodhankar SL. 2016. Wound healing potential of naringin ointment formulation via regulating the expression of inflammatory, apoptotic and growth mediators in experimental rats. Pharm Biol. 54:419–432.
- Kandhare AD, Bodhankar SL, Mohan V, Thakurdesai PA. 2015a. Acute and repeated doses (28 days) oral toxicity study of glycosides based standardized fenugreek seed extract in laboratory mice. Regul Toxicol Pharmacol. 72:323–334.
- Kandhare AD, Bodhankar SL, Mohan V, Thakurdesai PA. 2015b. Effect of glycosides based standardized fenugreek seed extract in bleomycin-induced pulmonary fibrosis in rats: Decisive role of Bax, Nrf2, NF-κB, Muc5ac, TNF-α and IL-1β. Chem Biol Interact. 237:151–165.
- Kandhare AD, Bodhankar SL, Mohan V, Thakurdesai PA. 2015c. Prophylactic efficacy and possible mechanisms of oligosaccharides based standardized fenugreek seed extract on high-fat diet-induced insulin resistance in C57BL/6 mice. J App Pharma Sci. 5:035–045.
- Kandhare AD, Bodhankar SL, Singh V, Thakurdesai PA, Mohan V. 2013a. Anti-asthmatic effects of type-A procyanidine polyphenols from cinnamon bark in ovalbumin-induced airway hyperresponsiveness in laboratory animals. Biomed Aging Pathol. 3:23–30.
- Kandhare AD, Ghosh P, Bodhankar SL. 2014a. Naringin, a flavanone glycoside, promotes angiogenesis and inhibits endothelial apoptosis through modulation of inflammatory and growth factor expression in diabetic foot ulcer in rats. Chem Biol Interact. 219:101–112.
- Kandhare AD, Ghosh P, Ghule AE, Zambare GN, Bodhankar SL. 2013b. Protective effect of Phyllanthus amarus by modulation of endogenous biomarkers and DNA damage in acetic acid induced ulcerative colitis: Role of phyllanthin and hypophyllanthin. Apollo Med. 10:87–97.
- Kandhare AD, Kumar VS, Adil M, Rajmane AR, Ghosh P, Bodhankar SL. 2012a. Investigation of gastro protective activity of Xanthium strumarium L. by modulation of cellular and biochemical marker. Orient Pharm Exper Med. 12:287–299.
- Kandhare AD, Patil MV, Bodhankar SL. 2015d. L-Arginine attenuates the ethylene glycol induced urolithiasis in ininephrectomized hypertensive rats: role of KIM-1, NGAL, and NOs. Ren Fail. 37:709–721.
- Kandhare AD, Raygude KS, Ghosh P, Bodhankar SL. 2011a. The ameliorative effect of fisetin, a bioflavonoid, on ethanol-induced and pylorus ligation-induced gastric ulcer in rats. Inter J Green Pharm. 5:236–243.
- Kandhare AD, Raygude KS, Ghosh P, Ghule AE, Bodhankar SL. 2012b. Neuroprotective effect of naringin by modulation of endogenous biomarkers in streptozotocin induced painful diabetic neuropathy. Fitoterapia. 83:650–659.
- Kandhare AD, Raygude KS, Ghosh P, Ghule AE, Bodhankar SL. 2012c. Therapeutic role of curcumin in prevention of biochemical and behavioral aberration induced by alcoholic neuropathy in laboratory animals. Neurosci Lett. 511:18–22.
- Kandhare AD, Raygude KS, Ghosh P, Ghule AE, Gosavi TP, Badole SL, Bodhankar SL. 2012d. Effect of hydroalcoholic extract of Hibiscus rosa sinensis Linn. leaves in experimental colitis in rats. Asian Pac J Trop Biomed. 337–344.
- Kandhare AD, Raygude KS, Ghosh P, Gosavi TP, Bodhankar SL. 2011b. Patentability of animal models: India and the globe. Inter J Pharm Bio Arc. 2:1024–1032.
- Kandhare AD, Raygude KS, Shiva Kumar V, Rajmane AR, Visnagri A, Ghule AE, Ghosh P, Badole SL, Bodhankar SL. 2012e. Ameliorative effects quercetin against impaired motor nerve function, inflammatory mediators and apoptosis in neonatal streptozotocin-induced diabetic neuropathy in rats. Biomed Aging Pathol. 2:173–186.
- Kandhare AD, Shivakumar V, Rajmane A, Ghosh P, Bodhankar SL. 2014b. Evaluation of the neuroprotective effect of chrysin via modulation of endogenous biomarkers in a rat model of spinal cord injury. J Nat Med. 68:586–603.
- Katyare S, Satav J. 1991. Altered kinetic properties of liver mitochondrial membrane-bound enzyme activities following paracetamol hepatotoxicity in the rat. J Biosci. 16:71–79.
- Kaufman DW, Kelly JP, Rosenberg L, Anderson TE, Mitchell AA. 2002. Recent patterns of medication use in the ambulatory adult population of the United States: the Slone survey. JAMA. 287:337–344.
- Kaushal R, Dave KR, Katyare SS. 1999. Paracetamol hepatotoxicity and microsomal function. Environ Toxicol Pharmacol. 7:67–74.
- Ketkar S, Rathore A, Kandhare AD, Lohidasan S, Bodhankar SL, Paradkar A, Mahadik K. 2015. Alleviating exercise induced muscular stress using neat and processed bee pollen: oxidative markers, mitochondrial enzymes and myostatin expression in rats. Integr Med Res. 4:147–160.
- Kshirsagar A, Ingawale D, Ashok P, Vyawahare N. 2009. Silymarin: a comprehensive review. Pharmacog Rev. 3:116–124.
- Kulkarni S, Akula KK, Dhir A. 2008. Effects of Withania sonmifera Dunal root extract against pentylenetetrazol seizure threshold in mice: possible involvement of GABAergic system. Indian J Exper Biol. 46:465–469.
- Kumar VS, Rajmane AR, Adil M, Kandhare AD, Ghosh P, Bodhankar SL. 2014. Naringin ameliorates acetic acid induced colitis through modulation of endogenous oxido-nitrosative balance and DNA damage in rats. J Biomed Res. 28:132–145.
- Larson AM, Polson J, Fontana RJ, Davern TJ, Lalani E, Hynan LS, Reisch JS, Schiødt FV, Ostapowicz G, Shakil AO, Lee WM. 2005. Acetaminophen-induced acute liver failure: results of a United States multicenter, prospective study. Hepatology. 42:1364–1372.
- Malik T, Pandey DK, Dogra N. 2013. Ameliorative potential of aqueous root extract of Withania somnifera against paracetamol induced liver damage in mice. Pharmacologia. 4:89–94.
- Mishra L-C, Singh BB, Dagenais S. 2000. Scientific basis for the therapeutic use of Withania somnifera (ashwagandha): a review. Altern Med Rev. 5:334–346.
- Mitchell JR, Jollow DJ, Potter WZ, Gillette JR, Brodie BB. 1973. Acetaminophen-induced hepatic necrosis. IV. Protective role of glutathione. J Pharmacol Exp Ther. 187:211–217.
- Mohan H. 2002. The liver, biliary tract and exocrine pancreas. Text Book of Pathology, New Delhi: Jaypee Brothers Medical Publishers (P) Ltd.
- Momen-Beitollahi J, Mansourian A, Momen-Heravi F, Amanlou M, Obradov S, Sahebjamee M. 2010. Assessment of salivary and serum antioxidant status in patients with recurrent aphthous stomatitis. Med Oral Patol Oral Cir Bucal. 15:e557–e561.
- Moore M, Thor H, Moore G, Nelson S, Moldéus P, Orrenius S. 1985. The toxicity of acetaminophen and N-acetyl-p-benzoquinone imine in isolated hepatocytes is associated with thiol depletion and increased cytosolic Ca2+. J Biol Chem. 260:13035–13040.
- Olaleye MT, Rocha B. 2008. Acetaminophen-induced liver damage in mice: effects of some medicinal plants on the oxidative defense system. Exp Toxicol Pathol. 59:319–327.
- Ottani A, Leone S, Sandrini M, Ferrari A, Bertolini A. 2006. The analgesic activity of paracetamol is prevented by the blockade of cannabinoid CB1 receptors. Eur J Pharmacol. 531:280–281.
- Patil A, Guru A, Mukhrjee A, Sengupta A, Sarkar S, Parmar HM, Kandhare AD, Muthal AP, Bodhankar SL. 2015. Elucidation of gastro-protective activity of morin in pylorus ligation induced gastric ulcer via modulation of oxidative stress. Der Pharmacia Lettre. 7:131–139.
- Patwardhan B, Mashelkar RA. 2009. Traditional medicine-inspired approaches to drug discovery: can Ayurveda show the way forward? Drug Discov Today. 14:804–811.
- Randle L, Sathish J, Kitteringham N, Macdonald I, Williams D, Park B. 2008. α1-Adrenoceptor antagonists prevent paracetamol-induced hepatotoxicity in mice. Br J Pharmacol. 153:820–830.
- Raygude KS, Kandhare AD, Ghosh P, Ghule AE, Bodhankar SL. 2012. Evaluation of ameliorative effect of quercetin in experimental model of alcoholic neuropathy in rats. Inflammopharmacology. 20:331–341.
- Sarkar A, Sengupta A, Mukhrjee A, Guru A, Patil A Kandhare AD, Bodhankar SL. 2015. Antiulcer potential of morin in acetic acid-induced gastric ulcer via modulation of endogenous biomarkers in laboratory animals. Pharmacologia. 6:273–281.
- Sarkate AP, Murumkar PR, Lokwani DK, Kandhare AD, Bodhankar SL, Shinde DB, Bothara KG. 2015. Design of selective TACE inhibitors using molecular docking studies: Synthesis and preliminary evaluation of anti-inflammatory and TACE inhibitory activity. SAR QSAR Environ Res. 26:905–923.
- Tilg H, Wilmer A, Vogel W, Herold M, Nölchen B, Judmaier G, Huber C. 1992. Serum levels of cytokines in chronic liver diseases. Gastroenterology. 103:264–274.
- Visnagri A, Kandhare AD, Bodhankar SL. 2015. Renoprotective effect of berberine via intonation on apoptosis and mitochondrial-dependent pathway in renal ischemia reperfusion-induced mutilation. Ren Fail. 37:482–493.
- Visnagri A, Kandhare AD, Chakravarty S, Ghosh P, Bodhankar SL. 2014. Hesperidin, a flavanoglycone attenuates experimental diabetic neuropathy via modulation of cellular and biochemical marker to improve nerve functions. Pharm Biol. 52:814–828.
- Visnagri A, Kandhare AD, Ghosh P, Bodhankar SL. 2013. Endothelin receptor blocker bosentan inhibits hypertensive cardiac fibrosis in pressure overload-induced cardiac hypertrophy in rats. Cardiovasc Endocrinol. 2:85–97.
- Visnagri A, Kandhare AD, Shiva Kumar V, Rajmane AR, Mohammad A, Ghosh P, Ghule AE, Bodhankar SL. 2012. Elucidation of ameliorative effect of co-enzyme Q10 in streptozotocin-induced diabetic neuropathic perturbation by modulation of electrophysiological, biochemical and behavioral markers. Biomed Aging Pathol. 2:157–172.