Abstract
Objectives. Previous studies have suggested that gastrointestinal integrity is compromised after cardiopulmonary bypass (CPB). We compared the effects of prolonged minimized (MCPB) and conventional CPB (CCPB) on intestinal mucosal integrity by determining mucosal damage, epithelial cell proliferation rate and distribution of tight junction proteins in a porcine model. Design. Fourteen animals were randomly assigned to undergo 240 minutes of mild hypothermic MCPB or CCPB. Ileal and colonic biopsies were obtained prior and at the end of CPB. Mucosal damage was determined under light microscopic evaluation. Immunohistochemistry was used to investigate epithelial expression of Ki-67 as a measure of cell proliferation rate and claudin-1, 2, 3, 4, 5, and 7 as elements of tight junctions. Results. In colonic biopsies, independent of the circuit type used, moderate mucosal damage was observed as indicated by focal epithelial damage, increased epithelial cell proliferation and decreased expression of tight junction protein claudin-4. Conclusions. Colonic mucosal damage was observed similarly in MCPB and CCPB. Based on these results, the effects of MCPB on intestinal mucosal stability are similar to those of CCPB.
The interest in splanchnic compatibility during cardiopulmonary bypass (CPB) is beyond preventing abdominal complications after cardiac surgery, as current understanding of gastrointestinal physiology implicates the gastrointestinal tract in the pathogenesis of the systemic inflammatory response syndrome (SIRS) and multiple organ failure (MOF) (Citation1). Previous studies have shown that while global splanchnic perfusion is reasonably well-maintained during CPB (Citation2,Citation4), splanchnic oxygen consumption is enhanced (Citation5) and intestinal permeability increases (Citation6,Citation7). However, the data on mucosal tissue perfusion and oxygenation during CPB are controversial (Citation2,Citation3,Citation8–11).
In an attempt to attenuate the adverse effects of CPB, the use of minimized cardiopulmonary bypass (MCPB) systems has recently increased. As these circuits lack a venous reservoir, intravascular filling may be hypothesized to maintain more physiologically compared to conventional cardiopulmonary bypass (CCPB). This, along with a well-maintained hematocrit level (Citation12), provides a theoretical basis for better-preserved tissue perfusion. To date, the effects of MCPB on splanchnic function have not been reported.
The present study was designed to investigate the effects of prolonged mild hypothermic MCPB and CCPB on intestinal epithelial integrity in terms of mucosal histological damage, epithelial cell proliferation rate and distribution of tight junction proteins. In addition, the effects of the two different circuit models on splanchnic blood flow and splanchnic oxygen delivery (DO2) and consumption (VO2) ratio were evaluated. It was hypothesized that MCPB would cause less alterations in intestinal mucosal integrity than CCPB.
Material and methods
Experimental setting
Fourteen female (6–7 weeks, weight range 28–39 kg) piglets from a native stock were randomly assigned to undergo mild hypothermic CPB using either a MCPB or CCPB circuit system. In addition, two animals served as sham controls and underwent the experimental protocol excluding the CPB. All experiments were performed in compliance with the guidelines of the European Convention for the Protection of Vertebrate Animals used for Experimental and Other Scientific Purposes (1986) and in compliance with the European Union Directive 86/609/EEC (1997). The research protocol was approved by the Animal Care and Use Committee of the University of Oulu, Finland.
Anesthesia
Animals were deprived of food 24 hours before the experiment. After sedation with intramuscular ketamine hydrochloride (350 mg) and midazolam (45 mg), anesthesia was induced with intravenous thiopental (5–10 mg/kg). Following endotracheal intubation, the animals were mechanically ventilated with a volume-controlled ventilator (Servo 900C, Siemens, Solna, Sweden). Tidal volume was kept at 10 ml/kg while the respiratory rate and inspired O2-concentration were adjusted to maintain PaCO2 levels between 4.5 kPa and 5.5 kPa, and PaO2 levels between 13 kPa and 20 kPa. Anesthesia was maintained with continuous infusions of fentanyl 25 μg/kg/hour and midazolam 0.25 mg/kg/hour combined with isoflurane (0.5%). Muscle relaxation was achieved with a pancuronium 0.2 mg/kg/hour. In addition, 1.5 g of cefuroxime was administered intravenously.
In all animals, an arterial catheter was inserted via left femoral artery and a pulmonary artery thermodilution catheter (CritiCath 7F; Ohmeda GmbH & Co, Erlangen, Germany) was introduced via left femoral vein. An orogastric tube and a urinary catheter were inserted. The animals received 0.9% saline at a constant rate of 10 ml/kg/hour. Ringer's lactate and hydroxyethyl starch (Voluven™) at a ratio of 2:1 was additionally administered to keep the pulmonary artery occlusion pressure between 5 mmHg and 8 mmHg. Blood glucose level was kept between 5–7 mmol/l by intravenous infusion of 30% glucose if necessary. The normal porcine body temperature of 39°C ± 0.5 was maintained until the induction of CPB.
Cardiopulmonary bypass
In the CCPB group, cardiopulmonary bypass circuit consisted of biocompatible material coated (phosphorylcholine) lines, a hard shell venous reservoir, a membrane oxygenator, (D905 Eos; Dideco SpA, Mirandola, Italy) and a roller pump. The MCPB circuit consisted of biocompatible material coated (phosphorylcholine) minimized circuit with a soft-shell reservoir bag, a venous bubble trap and a membrane oxygenator (ECC.O, Dideco SpA, Mirandola, Italy) with an integrated centrifugal pump (Stöckert SCP, Sorin Group Deutschland GMBH, München, Germany). The circuits were primed with a crystalloid solution containing 50 mg of heparin. In the MCPB group, retrograde autologous priming was performed at the initiation of the CPB to displace about 150 ml of the crystalloid prime. No blood transfusions were given. Pump flow was initiated at a flow rate of 90 to 110 ml/kg/min and adjusted to maintain an arterial pressure of 50 to 70 mmHg and a mixed venous saturation (SvO2) above 70%. No vasoactive drugs were used. The animals were cooled down to 34°C but rewarmed to 36°C during the last 30 minutes of CPB. The aorta was not clamped, but the animals were disconnected from the ventilator.
Surgical preparations
All surgical preparations were performed by full trained surgeons. The abdominal cavity was exposed through a midline laparotomy. The superior mesenteric artery and celiac trunk were exposed and transit time ultrasonic flow probes (Transonic Systems, Ithaca, NY, USA) were secured around the vessels. A catheter was inserted into the portal vein. Baseline intestinal biopsies (PRECPB) representing all layers of the intestinal wall were obtained from terminal ileum and ascending colon (one each), and intestinal walls and the biopsy sites were carefully sutured. Thereafter, the abdominal wall was reapproximated but the fascia was left open. A right anterolateral thoracotomy was performed in the fourth intercostal space. The right thoracic vessels were ligated and cut, and the pericardium was opened. The animals received heparin (5 mg/kg) with additional boluses if necessary to maintain activated clotting time of more than 400 seconds. Thereafter, a 12-F arterial cannula and a 24-F venous cannula were inserted into the ascending aorta and the right atrial appendage, respectively.
Experimental protocol
The animals were allowed to stabilize for 60 minutes before the baseline measurements were obtained. Then, mild hypothermic CPB was initiated and maintained for four hours (240 minutes). After post procedural biopsies (CPB240min) from terminal ileum and ascending colon (one each) were obtained, the animals were killed with intravenous pentobarbital (60 mg/kg).
Hemodynamic measurements
Arterial, pulmonary and central venous pressures were continuously monitored with quartz pressure transducers and a Datex monitor (Datex-Ohmeda). All pressure transducers were zeroed at the level of the heart. At baseline, cardiac output was measured using the thermodilution catheter and indexed to weight (CI). During CPB, CI was calculated from pump flow. Regional blood flows of superior mesenteric artery and celiac trunk (Qspl) were measured continuously with the transit time flow probes attached to a flow meter.
Blood samples
Arterial blood samples were drawn every 30 minutes to analyze blood gas values, pH, electrolytes, glucose- and hemoglobin concentrations with an i-STAT Analyzer (i-STAT Corporation, East Windsor, NJ, USA). Pulmonary and portal vein samples were taken every 60 minutes to measure SvO2 and portal vein saturation (SpO2). In addition, systemic arterial and portal venous lactate concentrations were analyzed every 120 minutes.
Oxygen delivery and consumption
Systemic oxygen delivery index (DO2i) was calculated as [CI × Hb × SaO2 × 0.0139] and systemic oxygen consumption index (VO2i) as [CI × Hb × (SaO2–SvO2) × 0.0139]. Qspl was indexed to body weight (Qspli). Gut oxygen delivery index (GutDO2i) was calculated as [Qspli × Hb × SaO2 × 0.0139], and splanchnic oxygen consumption index (VO2i) as [Qspli × Hb × (SaO2–Portal vein O2 saturation) × 0.0139].
Histopathologic evaluation and immunohistochemistry
Biopsies were fixed in neutral buffered 10% formalin for three days, embedded in paraffin and cut into 5 μm thick sections that were stained in hematoxylin and eosin. Histopathologic evaluation under light microscope at 40X and 100X included measurements of the villi and crypt height with a calibrated ocular micrometer, quantitation of the amount of inflammatory cells and estimation of the microvascular filling. These features were separately assessed in mucosal, submucosal, muscular and serosal layers. Mucosal injury was assessed by an unbiased experienced pathologist. Histologic criteria for epithelial damage were increased eosinophilic staining intensity of epithelial cells, karyopyknosis, karyolysis, and karyorrhexis, cell disruption, subepithelial edema and detachment of cells from the basal lamina. Morphologic damage was expressed as mucosal damage score developed to grade morphologic damage ().
Table I. Mucosal epithelial damage score and combined score for the assessment of immunohistochemical staining in claudin proteins.
Immunohistochemistry was used to investigate epithelial expression of Ki-67 as a measure of proliferation as well as claudin-1, 2, 3, 4, 5, and 7 as elements of tight junctions. The sections were dewaxed in xylene and rehydrated in graded alcohol series to PBS, pre-treated with TRIS/EDTA and heated in a microwave oven for 17 minutes. The endogenous peroxidase activity was quenched by incubating the specimen for 15 minutes with DAKO Peroxidase Block. Primary antibodies were purchased from Zymed Laboratories Inc. (San Francisco, CA, USA) for detection of claudins and from Immunotech (Marseille, France) for detection of cell proliferation (Ki-67). Polyclonal rabbit anti-claudin 1 (clone JAY.8), monoclonal mouse anti-claudin 2 (clone 12H12), polyclonal rabbit anti-claudin 3 (clone Z23.JM), monoclonal mouse anti-claudin 4 (clone 3E2C1), monoclonal mouse anti-claudin 5 (clone 4C3C2) and polyclonal rabbit anti-claudin 7 (clone ZMD.241) and monoclonal mouse anti-MIB-1 (Ki-67, clone MIB-1) were used. After a 30 minute incubation with a primary antibody (dilution 1:50 for anti-claudin 1, 2, 3, 4, 5, and 7 and 1:25 for anti-MIB-1), staining was completed with the EnVision Detection Systems Peroxidase/DAB (rabbit/mouse) for automated systems (Dako, Glostrup, Denmark) with Dako Autostainer (Dako, Glostrup, Denmark). The sections were lightly counterstained with haematoxylin and mounted with Eukitt (Kindler, Freiburg, Germany). Negative control stains were carried out by substituting phosphate buffered saline for the primary antibodies. The immunoreactivity was assessed quantitatively. In each section, multiple readings of immunoreactive staining were obtained at 500 μm intervals and the mean of these 15–20 readings for each section provided a staining intensity for that section. Staining was categorized into five classes (0 = absent, 1 = weak, 2 = moderate, 3 = strong, 4 = very strong) in terms of intensity and quantity (). For epithelial cell proliferation rate, approximately 300 epithelial cells in each sample were evaluated and the Ki-67 index was calculated as the percentage of cells showing nuclear Ki-67-expression. All assessments were performed blinded to the group and sampling time point by two investigators.
Statistical analysis
Statistical analysis was performed with SPSS (version 17.0; SPSS Inc, Chicago, Ill, USA) and SAS (version 9.1.3; SAS Institute, Inc, Cary, NC, USA) statistical software packages. Either the Student t-test or Mann-Whitney U-test was used to assess the distribution of variables between the study groups. Differences between two related measurements, such as histopathologic measurements, were analyzed using Paired samples t-test or Wilcoxon signed rank test as appropriate. The SAS procedure Mixed was used for repeated measurements. Complete independence was assumed across animals (by random statement). Reported p-values are as follows: For repeated measurements, p between groups (pg) indicates a level of difference between the groups, p over time (pt) indicates changes of measurements over time, p for time by group (p t*g), indicates behavior between the groups with time. For histopathologic measurements, pgroup indicates the distribution of variables between the study groups and ptime indicates the level of difference between two measurements. Two-tailed significance levels are reported. Data are presented as the median with 25th and 75th percentiles.
Results
The protocol was carried out in 20 animals. Three animals in both study groups were excluded from the analyses due to surgical difficulties. The median weight of the remaining 14 animals were 32.8 (31.1–33.8 kg) in the MCPB group and 31.9 (31.1–36.3 kg) in the CPB group (p = 0.57).
Hemodynamic and metabolic data
Descriptive data are summarized in . At baseline, hemodynamic and metabolic variables showed no significant differences between the two groups. Characteristic for the native pig stock used, baseline Hb levels were reasonably low. Hemodilution occurred during CPB, with a tendency (pg = 0.08) for higher Hb values in the MCPB group. The volume of intravenous fluids infused was similar in both groups. Pump flow during CPB was lower in the MCPB group than in the CCPB group. Larger portion of systemic blood flow was directed to the splanchnic region in the MCPB group, and Qspli decreased comparably in both groups. Systemic and gut DO2i values declined similarly in both groups. Gut VO2i was stable in both groups, and portal venous O2 (SpO2) saturation declined in line with the systemic mixed venous O2 saturation. Due to the surgical preparations, baseline systemic and portal venous lactate concentrations were somewhat elevated in both groups as well as in sham animals. After baseline, lactate values normalized in sham animals, with no significant changes from baseline in the study groups. However, systemic lactate concentrations were consistently higher in the CCPB than MCPB group.
Table II. Descriptive data of the study groups and the two sham animals.
Histopathologic evaluation and immunohistochemistry
In all groups, light microscopic examination of baseline biopsies showed intact ileal mucosal structure with minor focal epithelial degeneration in colon samples. There were no changes relative to the baseline ileal or colonic biopsies in sham animals at the end of the experiment. In both study groups, the height of villi and crypts, the amount of inflammatory cells as well as microvascular filling were comparable to baseline. However, mild focal degeneration of mucosal epithelium was detected in ileal CPB240 biopsies in both groups. The focal epithelial alterations were more evident in colonic CPB240 biopsies in both CPB groups (). In sites of the damage, colonic epithelial cells were shrunken and nuclei showed pyknosis and karyolysis and there were foci showing detachment of the epithelial cells from the basal lamina (). These changes were present only on the luminal epithelium while the crypts remained intact. Ileal epithelial cell proliferation rate (Ki67 index) remained constant despite of CPB in both groups, while in colonic specimens epithelial cell proliferation rate showed a slight increase after CPB without an intergroup difference (data not shown, ptime < 0.05, pgroup = 0.51). In sham animals, the proliferation rate stayed constant in both ileal and colonic biopsies.
Figure 1. Mucosal damage scores (median, 25th, 75th percentiles) of ileal (ileal) and colon (right) biopsies at baseline and at 240 minutes of cardiopulmonary bypass (CPB) in the study groups and in the two sham animals.
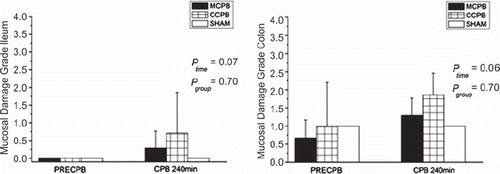
There were differences in mucosal epithelial cell immunostaining of various claudins (CL). CL1 was clearly cytosolic-bound and expressed abundantly in both ileal and colonic epithelium. CL2 was more membrane-bound, present in ileal villi, excluding the villus tips, and in the deeper layer of the colonic mucosa. CL3 and CL7 were constantly expressed both in cytoplasm and membranes of ileal and colonic epithelial cells. CL4 staining was slightly more membrane bound and expression was stronger on the apical parts of both ileal and colonic samples. CL5 was evenly stained in cytoplasms and membranes while staining was fainted towards the basal parts of the epithelium. Immunostaining of CL1, 2, 3, 5 and 7 revealed no differences in relation of group or time (). Ileal membrane-bound CL4 decreased slightly during CPB in the two groups combined, with no statistically significant differences between the groups (). In colon samples this decrease was found in both membrane- and cytoplasmic-bound CL 4 without any significant differences between groups ().
Figure 2. Median (25th, 75th percentiles) combined scores of cells staining positively for claudins in ileum and colon in the study groups and in the two sham animals. CL1, claudin-1; CL2, claudin-2; CL3, claudin-3; CL4, claudin-4; CL5, claudin-5; CL7, claudin-7.
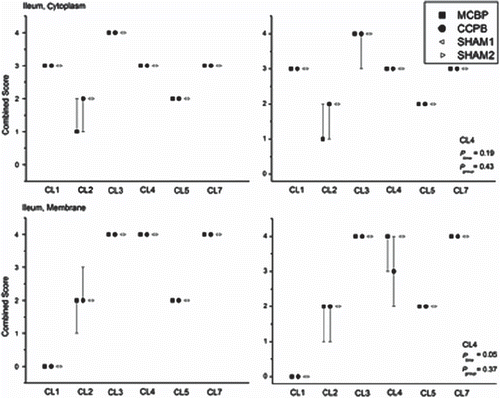
Figure 3. Micrographs of hematoxylin/eosin (HE-)-stained sections of colon biopsies of animals in the MCPB (first row), CCPB (second row) groups and in a sham animal (third row) at baseline and at 240 minutes on CPB. Bar = 250 μm.
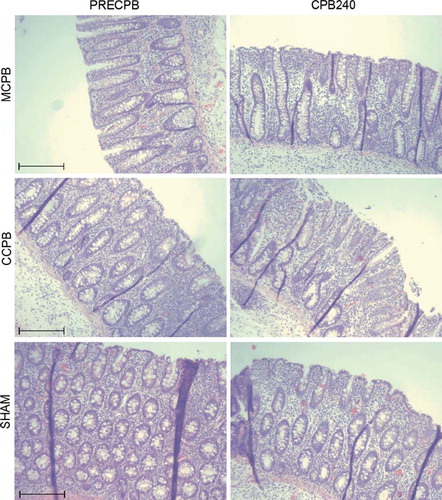
Figure 4. Micrographs of sections of colon biopsies showing immunohistochemical staining for claudin-4 (CL4) in the minimized cardiopulmonary bypass (MCPB) (first row), conventional cardiopulmonary bypass (CCPB) (second row) groups and in a sham animal (third row) at baseline and at 240 minutes on cardiopulmonary bypass (CPB). Bar = 250 μm.
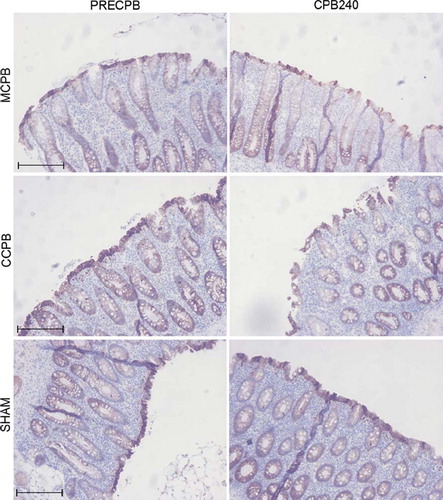
Discussion
The major finding of the present study is that during prolonged CPB, gut mucosal integrity was affected similarly by MCPB and CCPB. McIlwain and collegues (Citation13) reported similar, although more severe, alterations of porcine jejunal biopsies following eight hours of extracorporeal membrane oxygenation (ECMO). The pathophysiologic mechanisms causing CPB-related gastrointestinal dysfunction are largely unresolved and multifaceted, and their attenuation may require more than minimized circuits are able to provide. Our results support previous concerns of CPB related gastrointestinal dysfunction. There is some evidence that patients with over 120 minutes of CPB are at a greater risk of developing postoperative gastrointestinal complications (Citation14), suggesting that splanchnic integrity may be more threatened by prolonged duration of CPB. After 240 minutes of CPB we found intestinal mucosal damage in all animals except one in the MCPB group. However, the abnormalities were only moderate and given the intestinal epithelial restitution capacity even after severe damage following hemorrhagic shock (Citation15), it may be assumed that injuries of this degree would not have caused evident intestinal ischemia. Still, it is possible that epithelial barrier function was affected.
Intestinal mucosal integrity depends on the result of a dynamic equilibrium between tissue injury and repair mechanisms such as epithelial restitution and proliferation. Factors that may affect gut barrier function include enterocyte apoptosis and necrosis, as well as disruption of tight junctions that play a key role in maintaining epithelial monolayer integrity (Citation16). Intercellular tight junctions regulate the paracellular permeability of ions, macromolecules and cells and serve as a barrier against penetration of pathogenic bacteria and toxic luminal antigens including endotoxins (Citation17,Citation18). Intramembranous tight junction strands consist of transmembrane proteins, such as occludin (Citation19), tricellulin (Citation20) and claudins (Citation21). The claudin family membrane proteins determine the barrier properties of tight junctions (Citation17,Citation18). Previously, CPB has been shown to decrease the expression of tight junction proteins occludin and ZO–1 with a simultaneous increase in intestinal permeability (Citation7). To our knowledge, there are no previous studies concerning the intestinal expression of claudins after CPB. We investigated the expression and localizaton of claudins 1, 2, 3, 4, 5 and 7 and found that the immunostaining of intestinal apical CL4 protein was decreased after prolonged CPB. The role of CL4 is strongly related to barrier function as overexpression of CL4 has been shown to increase the paracellular barrier (Citation26). Previously, decreased and redistributed expression of CL4 protein has been reported in human active ulcerative colitis (Citation22), norovirus infection (Citation23) and in murine polymicrobial sepsis (Citation24,Citation25). On the other hand, there were no changes in expression of CL1, 3, 5 and 7 in jejunal biopsies of septic mice (Citation25). Similarly, we did not detect any changes in the expression of CL1, 2, 3, 5 and 7 in response to CPB. Since our study had no follow-up time, the distribution of claudins after CPB remains to be elucidated. The loss of expression of occludin and ZO-1 has been shown to further decrease in two hours after CPB (Citation7). Colonic Ki-67 expression rate, which is a measure of cell proliferation, was slightly increased as a result to prolonged CPB. This is in line with other observed alterations of the mucosal structure and tight junction proteins in colon samples and further indicates that CPB can induce significant epithelial destruction necessitating prompt regeneration.
The specific pathophysiologic mechanisms endangering the gastrointestinal integrity during CPB were not addressed in this study. There have been numerous attempts to clarify peripheral mucosal tissue perfusion and oxygenation during CPB with conflicting results (Citation2,Citation8–11). There are great methodological challenges in defining splanchnic microcirculation: results of a single site of measurement may not be applicable to overall tissue oxygenation. In our protocol, splanchnic oxygen delivery decreased during CPB in both study groups. We speculate that this was not only due to hemodilution but also to increased vascular resistance in the splanchnic region in response to CPB, even in the absence of vasoconstrictive agents. Taking into account the CPB related mesenteric endothelial dysfunction (Citation27) and increased splanchnic contractile response to α1-adrenergic agonists (Citation27,Citation28), vasopressor therapy may contribute to even more substantial hypoperfusion of splanchnic region during CPB.
Despite many anatomic and physiologic similarities between the porcine and human digestive and cardiovascular systems, caution must be taken when applying our findings to patients. The animals in this study had no underlying vascular disease and the observations of this study may not be directly generalized to adult patients with atherosclerotic alterations. Furthermore, a follow-up time would have allowed detection of clinical manifestations of impaired intestinal barrier function. Despite these limitations, this study provides important information on the hypothesis of impaired splanchnic integrity during CPB. It is the first study directly assessing intestinal mucosal integrity after prolonged CPB with two alternative circuit settings. Based on our results, the effects of MCPB on intestinal mucosal stability are not inferior to those of CCPB.
We conclude that after prolonged CPB, colonic mucosal damage was similarly observed in MCPB and CCPB as indicated by focal epithelial alterations under light microscopic evaluation, increased epithelial cell proliferation rate and decreased expression of tight junction protein claudin-4. Further studies are required to clarify the pathophysiological mechanisms behind these observations, as well as their clinical significance. However, our findings support the previous concerns about the splanchnic vulnerability during CPB and encourage into further attempts in optimizing CPB techniques.
Acknowledgments
We express our deepest gratitude to medical biostatistician Pasi Ohtonen for the expert guidance and assistance in this study. This study was supported by Finnish Foundation for Cardiovascular Research and Sigrid Juselius Foundation.
Declaration of interest: The authors report no conflicts of interest. The authors alone are responsible for the content and writing of the paper.
References
- Ackland G, Grocott MP, Mythen MG. Understanding gastrointestinal perfusion in critical care: So near, and yet so far. Crit Care. 2000;4:269–81.
- Tao W, Zwischenberger JB, Nguyen TT, Vertrees RA, McDaniel LB, Nutt LK, . Gut mucosal ischemia during normothermic cardiopulmonary bypass results from blood flow redistribution and increased oxygen demand. J Thorac Cardiovasc Surg. 1995;110:819–28.
- Haisjackl M, Birnbaum J, Redlin M, Schmutzler M, Waldenberger F, Lochs H, . Splanchnic oxygen transport and lactate metabolism during normothermic cardiopulmonary bypass in humans. Anesth Analg. 1998;86:22–7.
- Gårdebäck M, Settergren G, Brodin L, Jorfeldt L, Galuska D, Ekberg K, . Splanchnic blood flow and oxygen uptake during cardiopulmonary bypass. J Cardiothorac Vasc Anesth. 2002;16:308–15.
- Braun JP, Schroeder T, Buehner S, Dohmen P, Moshirzadeh M, Grosse J, . Splanchnic oxygen transport, hepatic function and gastrointestinal barrier after normothermic cardiopulmonary bypass. Acta Anaesthesiol Scand. 2004;48: 697–703.
- Oudemans-van Straaten HM, Jansen PG, Hoek FJ, van Deventer SJ, Sturk A, Stoutenbeek CP, . Intestinal permeability, circulating endotoxin, and postoperative systemic responses in cardiac surgery patients. J Cardiothorac Vasc Anesth. 1996;10:187–94.
- Sun YJ, Chen WM, Zhang TZ, Cao HJ, Zhou J. Effects of cardiopulmonary bypass on tight junction protein expression in intestinal mucosa of rats. World J Gastroenterol. 2008;14: 5868–75.
- Sicsic JC, Duranteau J, Corbineau H, Antoun S, Menestret P, Sitbon P, . Gastric mucosal oxygen delivery decreases during cardiopulmonary bypass despite constant systemic oxygen delivery. Anesth Analg. 1998;86:455–60.
- Haisjackl M, Germann R, Hasibeder W, Schwarz B, Salak N, Pajk W, . Mucosal tissue oxygenation of the porcine jejunum during normothermic cardiopulmonary bypass. Br J Anaesth. 1999;82:738–45.
- Nygren A, Thorén A, Houltz E, Ricksten SE. Autoregulation of human jejunal mucosal perfusion during cardiopulmonary bypass. Anesth Analg. 2006;102:1617–22.
- Dong GH, Wang CT, Li Y, Xu B, Qian JJ, Wu HW, Jing H. Cardiopulmonary bypass induced microcirculatory injury of the small bowel in rats. World J Gastroenterol. 2009;7: 3166–72.
- Benedetto U, Angeloni E, Refice S, Capuano F, Goracci M, Roscitano A, . Is minimized extracorporeal circulation effective to reduce the need for red blood cell transfusion in coronary artery bypass grafting? Meta-analysis of randomized controlled trials. J Thorac Cardiovasc Surg. 2009;138: 1450–3.
- McIlwain B, Timpa J, Kurndkar AR, Holt DW, Kelly DR, Hartman Y, . Plasma concentrations of inflammatory cytokines rise rapidly during ECMO-related SIRS due to the release of pre-formed stores in the intestine. Lab Invest. 2010;90:128–39.
- Khan JH, Lambert AM, Habib JH, Broce M, Emmett MS, Davis EA. Abdominal complications after heart surgery. Ann Thorac Surg. 2006;82:1796–801.
- Chang JX, Chen S, Ma LP, Jiang LY, Chen JW, Chang RM, . Functional and morphological changes of the gut barrier during the restitution process after hemorrhagic shock. World J Gastroenterol. 2005;11:5485–91.
- Keita AV, Söderholm JD. The intestinal barrier and its regulation by neuroimmune factors. Neurogastroenterol Motil. 2010;22:718–33.
- Van Itallie CM, Anderson JM. The molecular physiology of tight junction pores. Physiology (Bethesda). 2004;19: 331–8.
- Furuse M, Tsukita S. Claudins in occluding junctions of humans and flies. Trends Cell Biol. 2006;16:181–8.
- Furuse M, Hirase T, Itoh M, Nagafuchi A, Yonemura S, Tsukita S. Occludin: A novel integral membrane protein localizing at tight junctions. J Cell Biol. 1993;123:1777–88.
- Ikenouchi J, Furuse M, Furuse K, Sasaki H, Tsukita S, Tsukita S. Tricellulin constitutes a novel barrier at tricellular contacts of epithelial cells. J Cell Biol. 2005;171:939–45.
- Morita K, Furuse M, Fujimoto K, Tsukita S. Claudin multigene family encoding four-transmembrane domain protein components of tight junction strands. Proc Natl Acad Sci USA. 1999;96:511–6.
- Oshima T, Miwa H, Joh T. Changes in the expression of claudins in active ulcerative colitis. J Gastroenterol Hepatol. 2008;23(Suppl 2):S146–50.
- Troeger H, Loddenkemper C, Schneider T, Schreier E, Epple HJ, Zeitz M, . Structural and functional changes of the duodenum in human norovirus infection. Gut. 2009; 58:1070–7.
- Li Q, Zhang Q, Wang C, Liu X, Li N, Li J. Disruption of tight junctions during polymicrobial sepsis in vivo. J Pathol. 2009;218:210–21.
- Clark JA, Gan H, Samocha AJ, Fox AC, Buchman TG, Coopersmith CM. Enterocyte-Specific epidermal growth factor prevents barrier dysfunction and improves mortality in murine peritonitis. Am J Physiol Gastrointest Liver Physiol. 2009;297:G471–9.
- Van Itallie C, Rahner C, Anderson JM. Regulated expression of claudin-4 decreases paracellular conductance through a selective decrease in sodium permeability. J Clin Invest. 2001;107:1319–27.
- Doguet F, Litzler PY, Tamion F, Richard V, Hellot MF, Thuillez C, . Changes in mesenteric vascular reactivity and inflammatory response after cardiopulmonary bypass in a rat model. Ann Thorac Surg. 2004;77:2130–7.
- O'Dwyer C, Woodson LC, Conroy BP, Lin CY, Deyo DJ, Uchida T, . Regional perfusion abnormalities with phenylephrine during normothermic bypass. Ann Thorac Surg. 1997;63:728–35.