Abstract
Objectives. To establish a set of reference values for regional dispersion of repolarization of the right atrium in the in situ heart of pigs and to see if the global dispersion of repolarization could be estimated from regional mapping. Design. Monophasic action potential (MAP) were sequentially recorded from 28 ± 3 sites in seven different regional areas of the right atrium: lateral, anterior and posterior wall, septum, sinoatrial node (SAN), appendage, and near the tricuspid annulus (TA) in 10 healthy pigs using the CARTO mapping system. Results. The activation time (AT), MAP duration (MAPd) and end of repolarization time (EOR) of the whole right atrium were 68 ± 7, 239 ± 20 and 270 ± 23 ms, respectively. There were no significant differences on MAPd and EOR among the seven regional areas, nor between each of the regional and global values. The global dispersions of the MAPd and EOR were 75 ± 19 and 103 ± 13 ms, which were significantly greater than those obtained from any of the seven regional areas and those between two remote regions, SAN vs. TA and SAN vs. appendage regions. Conclusions. The data of regional and global dispersion of repolarization in healthy pigs can serve as reference values for evaluation of increased dispersion of repolarization. The global dispersions of MAPd and EOR in the right atrium were poorly estimated from regional mapping, suggesting the importance of global mapping in evaluating the dispersion of atrial repolarization.
Experimental evidence suggests that regional differences in action potential morphology can provide a substrate for initiation and maintenance of reentrant arrhythmias in the atrium (Citation1–3). Standard microelectrode recordings have demonstrated a potential substrate for atrial refractory heterogeneity, in terms of regional variability in action potential morphology between the right and left atria or among several regions within the right atrium (Citation4–6). Global sequence and dispersion of atrial repolarization in pigs and in patients have earlier been reported (Citation7,Citation8). However, very little is known about the in vivo regional heterogeneity of atrial repolarization. In the present study, by using the monophasic action potentials (MAPs) recording technique and an electro-anatomical mapping system (CARTO, Biosense Webster, Waterloo, Belgium), we studied the MAPs from seven different regions of the right atrium in healthy pigs in order to establish a set of reference values for evaluation of increased dispersion of repolarization in the in situ heart of pigs and to see if the global dispersion of repolarization could be estimated from regional mapping.
Methods
Subjects
Ten healthy pigs weighing 50–55 kg were studied. All the pigs were pre-medicated with azaperon (6 mg/kg) and thiopental (10–15 mg/kg), and anesthesia was maintained with an infusion of pancuronium (0.3 mg/kg/h), katamine (10 mg/kg/h) and midazolam (0.06 mg/kg/h) during the study. The pigs were intubated and artificially ventilated throughout the study.
The investigation conformed with the Guide for the Care and Use of Laboratory Animals and with the principles outlined in the Declaration of Helsinki. The study protocol was approved by the local ethics committee and the electrophysiologic procedures were in accordance with the local institutional guidelines.
CARTO mapping system
The nonfluoroscopic navigation and mapping system has been described in detail (Citation9). In brief, it is composed of a miniature passive magnetic field sensor incorporated into an ablation/mapping catheter, an external ultra-low magnetic field emitter (location pad), and a processing unit (CARTO, Biosense Webster). The spatial and temporal characteristics of the sensed magnetic fields contain the information needed to solve a set of over-determined algebraic equations yielding the location (x, y, and z) and orientation (roll, yaw, and pitch) of the catheter tip. This information enables tracking of the catheter tip while it is deployed within the heart cavity. The resolution of the location capabilities of the CARTO system was quantified previously and shown to be <1 mm for both in vitro and in vivo studies (Citation10).
MAP recording
To facilitate the MAP recording procedure and to improve the quality of the MAP recordings, a modified Navi-Star catheter (Cordis-Webster) was used, which has a contact ball of 0.5 mm in length and 1 mm in diameter at the end of the tip electrode. MAPs were recorded during sinus rhythm in all pigs. To minimize the influence of variations in heart rate, the cycle length stability monitor function of the CARTO system was used to avoid signal sampling when the cycle length variation was greater than 10% of the initial cycle length.
The Navi-Star recording catheter was introduced into the right atrium percutaneously through the right femoral vein. The MAPs were recorded sequentially from one site at a time in a random order at a filter band of 0.05–400 Hz. In the real-time monitor window of the CARTO system, one lead ECG, the MAP and a unipolar electrogram were displayed. When the amplitude and morphology of the MAP appeared satisfactory (Citation11,Citation12), the signals were captured into a sampling window for further inspection. Accepted MAP signals, together with 12-lead ECG, were stored using a sampling frequency of 1 kHz to the hard disk for off-line measurement.
To evaluate the activation and repolarization of different regions, the right atrium was divided into seven regional areas: the anterior, posterior and lateral free wall, the septum, auricle regions and the inferior region near the tricuspid annulus (TA); the high lateral part of the posterior wall where the sinoatrial node (SAN) located was separated from the posterior wall and defined as the SAN area (). MAPs were recorded in each region to obtain global as well as regional information about the repolarization of the right atrium. In order to avoid bias from variation in number of recordings among regions, recordings within 3 mm distance were counted as from the same site and only one of them used for data analysis.
MAP analysis
The MAP analysis was performed off-line using the double-annotation feature of the CARTO system. The first annotation line was set at the onset of the rapid phase of the MAP upstroke, representing local activation, and the second at 90% repolarization, representing local end of repolarization (). The two annotation lines were both manually set and carefully checked under a time scale of 100 mm/s and 50 mm/s, respectively.
Figure 2. Example screen captures from the CARTO MAP mapping system. Left: Sample window of the CARTO system showing simultaneous recordings of the time reference electrogram from coronary sinus (upper tracing), the monophasic action potential (MAP, middle tracing) and the surface ECG (lower tracing) from pig 1 (time scale 100 mm/s). On the MAP recording, the first annotation line (F, red) was set at the onset of the rapid phase of the MAP upstroke, representing the regional activation and the second line (S, yellow) at the 90% repolarization point, representing the local end of repolarization. The MAP duration is the time interval between the two annotation lines. Right: Typical MAP recordings from pig 4 (upper tracing), pig 7 (middle tracing) and pig 5 (lower tracing), time scale 50 mm/s. Note that MAP recordings from Pig 7 (middle tracing) are from a lower site near the tricuspid annulus and contain a contaminant of QRS complex; however, the measurement of MAP intervals is not influenced.
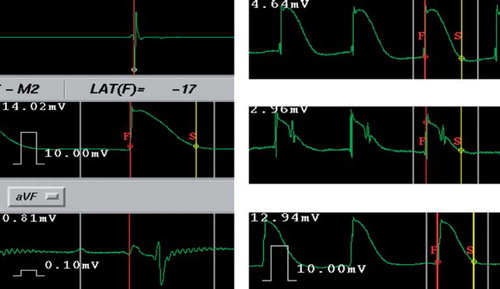
Activation time (AT) was defined as the time interval from the earliest recorded atrial activation to the local activation. MAP duration (MAPd) was defined as the time from the local activation to the local 90% of repolarization, i.e. the time interval between the two annotation lines. The end of repolarization time (EOR) was from the earliest atrial activation to the local 90% repolarization, which was equal to the sum of AT and MAPd at that recording site. Taking the maximal slope of an atrial electrogram from the coronary sinus as time reference, the relative values of AT and EOR were obtained (). MAPs were usually measured only from the beat captured in the sampling window of the CARTO system. However, when baseline disturbances and/or MAP distortions were present, the previous beat was also measured for necessary corrections.
According to the above definitions the relative measurement values were converted into the actual values of AT, EOR and MAPd. The maximal differences of AT, MAPd and EOR were defined as the maximal dispersion in these parameters. The values obtained from all the recordings were defined as global dispersions.
Statistical analysis
Results were presented as mean ± 1 S.D. in ms. Statistical tests between measurement values for sub-groups and between subgroups and the whole atrium were performed using the Mann-Whitney test. P-values of less than 0.05 were considered significant.
Results
MAPs were obtained from 28 ± 3 (range 23 to 32) sites in each pig. The size of each region ranged from 2.5 to 4.5 cm2 and the recording number in each region was 4 ± 0.5 (range 2 to 10). The mean values and the maximal dispersions of AT, MAPd and EOR from all the sites (global) and from the seven regional areas are presented in .
Table I. Mean value and maximal dispersions of AT, MAPd and EOR among global and regional recordings.
The average activation time for the whole right atrium was 68 ± 7 ms. The earliest activated region was located at the high lateral posterior wall of the right atrium – the SAN region – in all pigs. The activation propagated eccentrically and mostly (n = 8) ended in the TA region. A second latest activated region was observed in the auricle region (n = 7). No significant difference of regional AT was observed among the lateral free wall, septum, anterior and posterior wall.
The average global values of MAPd and EOR were 239 ± 20 ms and 270 ± 23 ms, respectively. There were no significant differences observed on MAPd among the seven regional areas and between the regional and the global values. The EOR in the inferior TA region, 293 ± 17 ms, was significantly greater than that in the SAN region, 245 ± 25 ms, p < 0.0001. No other significant differences on EOR were observed among the other regional areas.
The maximal global dispersions of MAPd and EOR were 75 ± 19 ms and 103 ± 13 ms, respectively. Among the seven regional areas, no significant difference of the dispersions of these parameters was observed, but all the regional dispersions were significantly smaller than the global ones, p < 0.01.
The maximal dispersions of MAPd and EOR between SAN and TA (SAN-TA) regions and between SAN and auricle (SAN-auricle) regions, i.e. between the earliest and latest activation regions, were also calculated and compared with the global values (). The dispersions of MAPd between both paired regions (58 ± 16 ms and 47 ± 15 ms, respectively) and the dispersion of EOR between the SAN-auricle regions (68 ± 21 ms) were significantly smaller than the global ones (75 ± 19 ms and 103 ± 13 ms, dispersion of MAPd and EOR respectively, p < 0.05). The dispersion of EOR between the SAN-TA regions was also much smaller than the global one, but with no statistical significance (83 ± 29 vs. 103 ± 13 ms respectively, p = 0.065).
Table II. Maximum dispersions of AT, MAPd and EOR among all the sites and between two remote regions.
Discussion
Unlike the findings in cellular and tissue preparation studies (Citation4–6), the present in vivo study in healthy pigs indicated no significant regional heterogeneity in repolarization throughout the right atrium. There were no significant differences on MAPd observed among seven different regions of the right atrium, including the regions around the SAN and near the TA. The EOR in the SAN region was significantly earlier than that in the inferior TA region, mainly due to the greater AT delay between these two remote areas, but no significant difference was found among the other regions. All dispersions of MAPd and EOR obtained from each individual region in the right atrium, including those between two remote regions, were markedly smaller than that among all the sites, i.e. the global dispersions.
Previous studies of regional variations in atrial repolarization
Standard microelectrode experiments have demonstrated a potential substrate for heterogeneity in atrial refractoriness, in terms of regional variability in action potential morphology (Citation4–6). The most detailed findings have been reported in dogs. As early as in 1968, Hogan and colleagues found consistent differences in action potential morphology and duration between the crista terminalis and several other right atrial regions (Citation4). Later, Spach et al. (Citation5) observed a progressive decrease in plateau amplitude and action potential duration from the crista terminalis to the pectinate muscles in multi-cellular preparations of the right atrium in dogs, and attributed this to the individual cellular properties of each region. In rabbits, action potential differences between the right and left atrial roofs (Citation6) and between the crista terminalis and pectinate muscles (Citation13) appeared to involve differences in transient outward K+ current (Ito). More recently, Feng et al. (Citation14) demonstrated systematic variations in action potential properties and ionic currents among myocytes from different right atrial regions and suggested that the spatial variation in ionic current density might explain the action potential differences.
In vivo studies of regional variation of atrial repolarization/refractoriness in normal atria are seldom reported. Li et al. (Citation15) compared the effective refractory period (ERP) between the right and left atrial free wall in dogs in vivo, and found the ERP was shorter in the left atrial free wall. Kamalvand et al. (Citation3) found that the ERP at the right atrial appendage was significantly longer than that at the mid-lateral right atrial wall in patients with normal atria, which was not consistent with the results of cellular studies (Citation14).
Comparison with previous studies
Although there is no definitive explanation for the different results between the previous studies and the current study, the inter-species (pig/dog or rabbit) differences as well as the differences in the pattern of atrial repolarization between isolated-preparation and in vivo study may play a role.
Different animals were studied in the present and previous studies. Structurally, the sinus node, atrio-ventricular node and His-Purkinje network are similar in the hearts of larger mammals, including humans. Many ionic channels and currents have been found to be present in pigs as in other larger mammals. However, the magnitude of these ionic currents have been demonstrated to be very different in the various species (Citation6,Citation13,Citation14), indicating that although the sequence of depolarization and repolarization is qualitatively similar in mammals, there may be important quantitative differences.
Secondly, in vitro recordings may not be representative in in vivo settings. In vivo electrophysiology, unlike that in vitro, is influenced by many factors such as the nervous system, circulating factors, metabolism and mechanical stretch (Citation16–18). Stuyvers et al. (Citation18) recently showed that physiological and electrophysiological parameters could be altered due to metabolic failure as stimulation rates increase, particularly in muscles thicker than 0.2 mm. Another example is the study of Liu and colleagues (Citation19). In their in vivo murine models, they found that all the MAPs from the left ventricular endocardium and epicardium demonstrated a similar morphology with a distinct plateau phase. However, in excised myocardial tissue preparations or single cell experiments, the typical murine cardiac MAP recordings were with short duration and lack of a plateau (Citation20,Citation21). Previous study (Citation22) demonstrated that reentry could occur in a three-dimensional plane using the epicardial and endocardial surfaces connected by transmural muscle fibers. In situ recording may provide repolarization of the whole endocardium, which may also contribute to the difference between ours and previous studies.
Another element that might also be mentioned is the number of recordings. Kamalvand et al. (Citation3) found that the ERP at the right atrial appendage was significantly longer than that at the mid-lateral right atrial wall by measuring at one site in each region in patients without clinical atrial fibrillation, but the phenomena were absent in patients with paroxysmal atrial fibrillation. Some studies (Citation14,Citation23) have demonstrated that the appendage and sino-atrial node cells had the greatest variability in morphology compared to other cells in atrium. In the present study, using the MAP recording and CARTO mapping system we recorded 28 ± 3 MAPs from seven different regions of the right atrium and found no significant difference on MAP duration among these regions. The global dispersions of repolarization in this study were also markedly greater than those in previous studies with less recording numbers (Citation14,Citation15,Citation24), and were poorly estimated by regional mapping, including that between two remote regions. In this respect, detailed mapping is more reliable in obtaining the global information on dispersion of repolarization as reported in our early studies in healthy pigs and in patients with or without atrial fibrillation (Citation7,Citation8).
Potential clinical implications
There is increasing evidence suggesting that the repolarization heterogeneity plays a role in the genesis and/or perpetuation of atrial fibrillation (Citation1–3). Although the present study provides only data of regional and global repolarization of the right atrium in healthy pigs, they could nevertheless serve as a set of reference values. Pigs are one of the easily available large animal in vivo models for atrial fibrillation (Citation25–27), and therefore basic values of repolarization from healthy pigs are useful in evaluation of increased dispersion of repolarization in pathological status such as in atrial fibrillation. To our knowledge, the data of regional atrial repolarization in pigs have not been reported before.
It has earlier been generally accepted that atrial fibrillation is mainly a left atrial disease (Citation28). However, the importance of substrates in the right atrium for perpetuation of AF has recently been highlighted again and even ablation of complex fractionate atrial electrograms is added to the right atrium for retaining sinus rhythm, especially in patients with longstanding persistent AF (Citation29,Citation30). In this regard, our data of the right atrial repolarization are useful in studying underlying mechanisms of and ablation strategies against atrial fibrillation, at least in pig models.
Conclusion
The data of regional and global dispersion of repolarization in healthy pigs can serve as reference values for evaluation of increased dispersion of repolarization. The global dispersions of MAPd and EOR of the right atrium were poorly estimated from regional mapping, indicating the importance of global mapping in evaluating the dispersion of atrial repolarization.
Declaration of interest: The authors report no conflicts of interest. The authors alone are responsible for the content and writing of the paper.
References
- Diker E, Ozdemir M, Aydogdu S, Tezcan UK, Korkmaz S, Kütük E, . Dispersion of repolarization in paroxysmal atrial fibrillation. Int J Cardiol. 1998;63:281–6.
- Li Z, Hertervig E, Yuan S, Yang Y, Lin Z, Olsson SB. Dispersion of atrial repolarization in patients with paroxysmal atrial fibrillation. Europace. 2001;3:285–91.
- Kamalvand K, Tan K, Lloyd G, Gill J, Bucknall C, Sulke N. Alterations in atrial electrophysiology associated with chronic atrial fibrillation in man. Eur Heart J. 1999;20:888–95.
- Hogan PM, Davis LD. Evidence for specialized fibers in the canine right atrium. Circ Res. 1968;23:387–96.
- Spach MS, Dolber PC, Anderson PA. Multiple regional differences in cellular properties that regulate repolarization and contraction in the right atrium of adult and newborn dogs. Circ Res. 1989;65:1594–611.
- Qi A, Yeung-Lai-Wah J, Xiao J, Kerr C. Regional differences in rabbit atrial repolarization: Importance of transient outward current. Am J Physiol. 1994;266:H643–9.
- Li Z, Hertervig E, Kongstad O, Holm M, Grins E, Olsson SB, . Global repolarization sequence of the right atrium. Monophasic action potential mapping in healthy pigs. PACE. 2003;26:1803–8.
- Hertervig E, Li Z, Kongstad O, Holm M, Grins E, Olsson SB, . Global dispersion of atrial repolarization in healthy pigs and patients. Scand Cardiovasc J. 2003;37:329–33.
- Gepstein L, Evans SJ. Electroanatomical mapping of the heart: Basic concepts and implications for the treatment of cardiac arrhythmias. Pacing Clin Electrophysiol. 1998; 21:1268–78.
- Gepstein L, Hayam G, Ben-Haim SA. A novel method for nonfluoroscopic catheter-based electroanatomical mapping of the heart. In vitro and in vivo accuracy results. Circulation. 1997;95:1611–22.
- Franz MR. Monophasic action potential recordings: What are they, how can they be recorded, what is their use? Franz MRC, Schmitt B, Zrenner. Monophasic action potentials. Berlin: Springer; 1997 22–39.
- Olsson SB, Yuan S. Technique and use of monophasic action potential recordings. WJ Mandel, Cardiac arrhythmias: Their mechanisms, diagnosis and management. 3rd. Philadelphia: J.B. Lippincott Company; 1995. 785–810.
- Yamashita T, Nakajima T, Hazama H, Hamada E, Murakawa Y, Sawada K, . Regional differences in transient outward current density and inhomogeneities of repolarization in rabbit right atrium. Circulation. 1995;92:3061–9.
- Feng J, Yue L, Wang Z, Nattel S. Ionic mechanisms of regional action potential heterogeneity in the canine right atrium. Circ Res. 1998;83:541–51.
- Li D, Zhang L, Kneller J, Nattel S. Potential ionic mechanism for repolarization differences between canine right and left atrium. Circ Res. 2001;88:1168–75.
- Yoshioka K, Gao DW, Chin M, Stillson C, Penades E, Lesh M, . Heterogeneous sympathetic innervation influences local myocardial repolarization in normally perfused rabbit hearts. Circulation. 2000;101:1060–6.
- Krummen DE, Narayan SM. Mechanisms for the initiation of human atrial fibrillation. Heart Rhythm. 2009;6:s12–6.
- Stuyvers B, McCulloch A, Guo J, Duff H, ter Keurs H. Effect of stimulation rate, sarcomere length and Ca2 + on force generation by mouse cardiac muscle. J Physiol. 2002;544: 817–30.
- Liu G, Iden J, Kovithavongs K, Gulamhusein R, Duff H, Kavanagh K. In vivo temporal and spatial distribution of depolarization and repolarization and the illusive murine T wave. J Physiol. 2004;555:267–79.
- Aslanili OV, Boyett MR, Dobrzynski H, Li J, Zhang. Mechanism of transition from normal to reentrant electrical activity in a model of rabbit atrial tissue: Interaction of tissue heterogeneity and anisotropy. Biophys J. 2009;96:798–817.
- Nakamura H, Ding WG, Sanada M, Maeda K, Kawai H, Maegawa H, . Presence and functional role of the rapidly activating delayed rectifier K(+) current in left and right atrial of adult mice. Eur J Pharmacol. 2010;649:14–22.
- Betts TR, Ho SY, Sanchez-Quintana D, Roberts PR, Anderson RH, Morgan JM. Three-dimensional mapping of right atrial activation during sinus rhythm and its relationship to endocardial architecture. J Cardiovasc Electrophysiol. 2002; 13:1152–9
- Misier AR, Opthof T, van Hemel NM, Defauw JJ, de Bakker JM, Janse MJ, . Increased dispersion of “refractoriness” in patients with idiopathic paroxysmal atrial fibrillation. J Am Coll Cardiol. 1992;19:1531–5.
- Fedorov VV, Chang R, Glukhov AV, Kostecki G, Janks D, Schuessler RB, . Complex interactions between the sinoatrial node and atrium during reentrant arrhythmias in the canine heart. Circulation. 2010;122:782–9.
- Nishida K, Michael G, Dobrev D, Nattel S. Animal models for atrial fibrillation: Clinical insights and scientific opportunities. Europace. 2010;12:160–72.
- Iino K, Yui N, Ooya T, Kawabata R, Tomita S, Watanabe G. Successful low-energy cardioversion using a novel biodegradable gel pad: Feasibility of treating postoperative atrial fibrillation in animals. J Thorac Cardiovasc Surg. 2007; 134:1519–25.
- Kneip CF, Mallet RT, Williams AG, Hamdan MH, Smith ML. Vagal modulation of heart rate variability during atrial fibrillation in pigs. Exp Biol Med. 2010;235:1007–14.
- Calkins H, Brugada J, Packer DL, Cappato R, Chen SA, Crijns HJ, . HRS/EHRA/ECAS Expert Consensus Statement on catheter and surgical ablation of atrial fibrillation: Recommendations for personnel, policy, procedures and follow-up: A report of the Heart Rhythm Society (HRS) Task Force on catheter and surgical ablation of atrial fibrillation. Heart Rhythm. 2007;4:816–61.
- Solheim E, Off MK, Hoff PI, Schuster P, Ohm OJ, Chen J. Characteristics and distribution of complex fractionated atrial electrograms in patients with paroxysmal and persistent atrial fibrillation. J Interv Card Electrophysiol. 2010;28: 87–93.
- Hocini M, Nault I, Wright M, Veenhuyzen G, Narayan SM, Jaïs P, . Disparate evolution of right and left atrial rate during ablation of long-lasting persistent atrial fibrillation. J Am Coll Cardiol. 2010;55:1007–16.