Abstract
We evaluated the in vitro antioxidant effect of alkyl-organotellurides A–D on lipid peroxidation and protein carbonylation in rat liver homogenates. The thiol oxidase and thiol peroxidase-like activities of compounds were investigated. δ-Aminolevulinic acid dehydratase (δ-ALA-D) activity was determined in rat liver homogenates. Compounds A–D protected against lipid peroxidation induced by Fe2+/EDTA and sodium nitroprusside (SNP). According to the confidence limits of the IC50 values of compounds A–D, the IC50 values for organotellurides followed the order: C (0.30 µM) ≤ B (0.40 µM) < D (0.68 µM) < A (2.90 µM), for Fe2+/EDTA, and B (0.21 µM) ≤ C (0.33 µM) ≤ D (0.43 µM) < A (1.21 µM) for SNP-induced lipid peroxidation. Compounds A–D reduced protein carbonyl content to control levels. The results demonstrated an inverse correlation between thiol oxidase and δ-ALA-D activities. This study supports an antioxidant effect of organotellurides A–D on rat liver.
Introduction
Evidence has been provided that organochalcogenides, such as organoselenium and organotellurium compounds, are promising pharmacological agentsCitation1–4. Organotellurium compounds have been synthesized since 1840Citation5, and it is known that these compounds are much more effective bioactive agents than their corresponding selenium and sulfur analogs, with evident chemoprotective, antioxidative, and cytotoxic propertiesCitation3,Citation6, making these compounds extremely attractive in medical therapies. However, little is known about the pharmacological and toxicological effects of these compoundsCitation7,Citation8. Engman et al.Citation1,Citation9 have investigated the possible anti-cancer properties of organotellurium compounds.
Several authors have reported that organotellurium compounds can exhibit antioxidant activity because of their glutathione peroxidase (GPx) mimetic propertiesCitation10 and peroxynitrite scavenger abilitiesCitation11. Moreover, studies have shown an antioxidant activity in vitroCitation11–13 and low toxicity ex vivoCitation11,Citation13,Citation14 of organotellurium compounds.
Oxidative stress is characterized by a significantly increased concentration of intracellular oxidizing species, such as reactive oxygen species (ROS), and is often accompanied by the simultaneous loss of antioxidant defense capacityCitation15,Citation16. In pathologic conditions an overproduction of ROS can occurCitation17. Besides that, ROS overproduction has been implicated in the installation and/or progression of a variety of human diseasesCitation18,Citation19 including diabetesCitation20, cancerCitation21, and various neurodegenerative diseasesCitation22. Thus, interest in the synthesis of antioxidant compounds that could potentially retard the development of diseases associated with oxidative stress has grown considerably in recent decadesCitation23,Citation24.
In this study, we evaluated the in vitro antioxidant effect of functionalized alkyl-organotellurides A–D against lipid peroxidation and protein carbonylation in rat liver homogenates. In addition, we examined the thiol peroxidase-like activity of functionalized alkyl-organotellurides A–D to determine the possible mechanism by which these compounds demonstrate antioxidant property. Furthermore, δ-aminolevulinic dehydratase (δ-ALA-D) and thiol oxidase activities were determined in rat liver homogenates.
Materials and methods
Synthesis of alkyl-organotellurides
Telluro-ketone A was synthesized by hydrotelluration of methyl vinyl ketone in 86% isolated yield by reacting elemental tellurium with n-butyllithium, followed by the addition of deoxygenated water and the enone as reported previouslyCitation25,Citation26. Tellurides B and C were prepared in good yields according to our previous protocolsCitation27–31 from telluride A. Finally, telluride D was prepared (65% isolated yield) from compound A by reacting with hydroxylamine hydrochlorideCitation32. summarizes the preparation of the mentioned compounds.
4-(Butyltellanyl)butan-2-one (A)
In a two-necked 50 mL flask under a nitrogen atmosphere were added sequentially elemental tellurium (0.511 g, 4.0 mmol) and dry tetrahydrofuran (THF; 5 mL). To the resulting mixture under vigorous stirring, at room temperature, was added n-butyllithium (4.0 mmol, 1.6 mol L−1 solution in hexane, 1.0 eq.). To the resulting pale yellow clean solution was added deoxygenated water (0.18 mL, 10 mmol). Methyl vinyl ketone (0.280 g, 4.0 mmol) was added in a portion at room temperature with vigorous stirring. The resulting mixture was stirred for 1 h at room temperature then quenched with water and diluted with hexane/ethyl acetate (5 mL, 1:1). The organic and aqueous phases were separated and the organic phase was washed with NH4Cl solution (3 × 15 mL) and brine (3 × 15 mL) and then dried with MgSO4. The solution was filtered and the solvent was evaporated. The residue was purified by silica gel column chromatography, eluting first with hexane and then with hexane/ethyl acetate (10:1). Yield: 0.87 g (86%). 1H NMR: (300 MHz, CDCl3, ppm) δ 0.92 (t, J = 7.4 Hz, 3H), 1.38 (sext, J = 7.4 Hz, 2H), 1.73 (q, J = 7.4 Hz, 2H), 2.15 (s, 3H), 2,69 (m, 4H), 3.03 (t, J = 7.4 Hz, 2H). Citation13C NMR: (75 MHz, CDCl3, ppm) δ 207.8, 46.4, 34.3, 29.8, 25.0, 13.3, 3.4, −7,0. 125Te MNR: (157 MHz, CDCl3, ppm, (PhSe)2) δ 279.6. LRMS: m/z (70 eV, rel. int.): 258 (M+, 7), 71 (40), 57 (24), 55 (26), 43 (100). IR: (ZnSe, cm−1) 2955, 2925, 2865, 1715. Anal. Calc.: C, 37.56; H, 6.30. Found: C, 37.32; H, 6.02%.
4-(Butyltelluro)butan-2-ol (B)
To a 125 mL round-bottomed flask were added telluride A (1.28 g, 5 mmol) and ethanol (20 mL). To the resulting solution was added an ethanolic solution of NaBH4 (0.21 g, 5.5 mmol in 6 mL ethanol). The mixture was stirred at room temperature and the progress of the reaction was monitored by thin layer chromatography (TLC). The reaction mixture was quenched with water (50 mL) and diluted with a mixture of hexane/ethyl acetate 1:1 (50 mL). The phases were separated and the aqueous phase was extracted with a mixture of hexane/ethyl acetate (1:1, 2 × 50 mL). The organic phases were combined, dried with MgSO4, and filtered and the solvents were removed under reduced pressure. The residue was purified by silica gel column chromatography eluting with a mixture of hexane/ethyl acetate (15:1). Yield: 0.89 g (70%). 1H NMR: (200 MHz, CDCl3, ppm) δ 0.85 (t, J = 7.2 Hz, 3H), 1.13 (d, J = 6.3 Hz, 3H), 1.31 (sext, J = 7.2 Hz, 2H), 1.65 (quint, J = 7.2 Hz, 2H), 1.76–1.85 (m, 2H), 2.53–2.69 (m, 4H), 3.75 (sext, J = 6 Hz, 1H). 13C NMR: (50 MHz, CDCl3, ppm) δ 69.1, 41.1, 34.2, 25.0, 23.2, 13.4, 2.7, −2.3. 125Te NMR: (157 MHz, CDCl3, ppm, (PhTe)2) δ 251.43. LRMS: m/z (70 eV, rel. int.) 260 (M+2, 13), 258 (M+, 13), 256 (7), 255 (3), 254 (2), 215 (3), 186 (8), 72 (5), 57 (73), 55 (100), 45 (44).
2-(Butyltellanyl)ethyl-2-methyl-1,3-dioxolane (C)
To a 50 mL two-necked flask equipped with a Dean–Stark reflux apparatus were added telluride A (1.28 g, 5 mmol), benzene (10 mL), ethylene glycol (0.44 mL, 8 mmol) and Amberlist® (30 mg). The reaction mixture was refluxed under a nitrogen atmosphere for 5 h. The mixture was filtered and the residue was washed with ethyl acetate. The solvents were removed under reduced pressure. The residue was purified by silica gel column chromatography eluting with a mixture of hexane/ethyl acetate (15:1). Yield: 1.41 g (96%). 1H NMR: (300 MHz, CDCl3, ppm) δ 0.92 (t, J = 7.3 Hz, 3H), 1.32 (s, 3H), 1.38 (sext, J = 7.3 Hz, 2 H), 1.72 (quint, J = 7.3 Hz, 2 H), 2.10–2.16 (m, 2 H), 2.60–2.66 (m, 4 H), 3.95 (m, 4 H). 13C NMR: (75 MHz, CDCl3, ppm) δ 110.2, 64.7, 42.1, 34.2, 25.0, 23.5, 13.3, 2.7, −5.6. 125Te NMR: (157 MHz, CDCl3, ppm, (PhTe)2) δ 263.3. LRMS: m/z (70 eV, rel. int.) 302 (M+, 3), 99 (4), 87 (100), 55 (15), 43 (58). IR: (film, cm−1) 2979, 2958, 2927, 2874, 1457, 1378, 1246, 1212, 1040, 944. Anal. Calc.: C, 40.13; H, 6.68. Found: C, 40.35; H, 6.46%.
4-(Butyltellanyl)butan-2-one oxime (D)
To a 50 mL round-bottomed flask were added telluride A (1.28 g, 5 mmol), hydroxylamine hydrochloride (0.552 g, 7.5 mmol), molecular sieves 3Å (1.25 g), and ethanol (20 mL). The mixture was stirred at room temperature, and the progress of the reaction was monitored by TLC. The mixture was filtered and the residue washed with hexane/ethyl acetate 1:1 (20 mL). The organic phase was sequentially washed with NaHCO3 (sat. sol., 2 × 20 mL), water (2 × 20 mL), and brine (20 mL). The organic phases were combined, dried with MgSO4, and filtered and the solvents were removed under reduced pressure. The residue was purified by silica gel column chromatography, eluting with a mixture of hexane/ethyl acetate (15:1). Yield: 0.88 g (65%). 1H NMR: (300 MHz, CDCl3, ppm) δ 0.91 (t, J = 7.2 Hz, 3H), 1.38 (sext, J = 7.2 Hz, 2H), 1.73 (quint, J = 7.2 Hz, 2H), 1.90 (s, 3H), 2.61–2.76 (m, 6H). 13C NMR: (75 MHz, CDCl3, ppm) δ 158.3, 79.4, 38.7, 34.1, 24.9, 13.4, 13.2, 2.8, −3.4. LRMS: m/z (70 eV, rel. int.) 271 (M+1, 11), 256 (6), 216 (44), 199 (7), 185 (5), 171 (4), 157 (2), 145 (6), 130 (8), 86 (44), 70 (16), 57 (100).
Chemicals
The chemical purity of organotellurides (99.9%) was determined by gas chromatography/high performance liquid chromatography (GC/HPLC). δ-Aminolevulinic acid (δ-ALA), p-dimethylaminobenzaldehyde, and reduced glutathione (GSH) were purchased from Sigma (St. Louis, MO, USA). All other chemicals were of analytical grade and obtained from standard commercial suppliers. Alkyl-organotellurides were dissolved in dimethylsulfoxide (DMSO).
Animals
Male adult Wistar rats, weighing 200–300 g, from our own breeding colony were used. The animals were kept in a separate animal room, on a 12 h light/dark cycle, at a room temperature of 22 ± 2°C, with free access to food (Guabi, RS, Brazil) and water. The animals were used according to the guidelines of the Committee on Care and Use of Experimental Animal Resources, Federal University of Santa Maria, Brazil.
Tissue preparation
Rats were euthanized and the liver tissue was rapidly dissected, placed on ice, and weighed. The liver tissue was immediately homogenized in cold 50 mM Tris-HCl, pH 7.4 (1/10, w/v). An aliquot of homogenate was used for the protein carbonyl assay. The homogenate was centrifuged at 2400 × g at 4°C for 10 min and a low-speed supernatant (S1) was used for lipid peroxidation and δ-ALA-D assays.
Thiol peroxidase-like and thiol oxidase assays were performed in the absence of S1.
Lipid peroxidation
This assay was carried out to determine the in vitro antioxidant effect of functionalized alkyl-organotellurides A–D. FeCl2/ethylenediaminetetraacetic acid (EDTA) or sodium nitroprusside (SNP) was used as the classical inductor of lipid peroxidation. Thiobarbituric acid reactive species (TBARS) were used as a measure of lipid peroxidation and determined as described by Ohkawa et al.Citation33. An aliquot of 200 μL of S1 was added to the reaction mixture containing: 100 μM FeCl2/50 μM EDTA or 50 μM SNP, 50 mM Tris-HCl, pH 7.4, and alkyl-organotellurides A–D at different concentrations (0.1–1 μM). The range of concentrations used for compound C was 0.001–1 μM. Compound A was used in a different range of concentrations in the lipid peroxidation assay induced with FeCl2/EDTA (0.1–5 μM), and the range of concentrations for compound B was 0.01–1 μM for lipid peroxidation induced with SNP. Vitamin E and trolox (0.1–50 μM) were used as positive controls. Afterward the mixture was pre-incubated for 1 h at 37°C. The reaction product was determined using 500 μL thiobarbituric acid (0.8%), 200 μL 8.1% sodium dodecyl sulfate (SDS), and 500 μL acetic acid buffer (pH 3.4), and incubated at 95°C for 2 h. The absorbance of samples was determined at 532 nm. Results are reported as nmol MDA (malondialdehyde) per gram of tissue.
Protein carbonyl content
Oxidatively modified proteins present an enhancement of carbonyl contentCitation34. This assay was utilized to determine the in vitro antioxidant effect of functionalized alkyl-organotellurides A–D against protein carbonylation induced by SNP. Carbonyl content was assayed by a method based on the reaction of protein carbonyls with 2,4-dinitrophenylhydrazine (DNPH) forming dinitrophenylhydrazone, a yellow compoundCitation35. Briefly, homogenate was diluted 1:10 (v/v) and incubated for 2 h with alkyl-organotellurides A–D at different concentrations (0.001–1 μM) and SNP (50 μM). Vitamin E (0.5 μM–5 mM) and trolox (0.5–100 μM) were used as positive controls. Then, 1 mL aliquots were mixed with 200 μL of 10 mM DNPH or 200 μL 2M HCl. After incubation at room temperature for 1 h in a dark environment, 500 μL of denaturing buffer (150 mM sodium phosphate buffer, pH 6.8, containing 3% SDS), 1.5 mL of heptane (99.5%), and 1.5 mL of ethanol (99.8%) were added sequentially, and mixed with vortex agitation for 40 s and centrifuged for 15 min. Next, the protein isolated from the interface was washed twice with 1 mL of ethyl acetate/ethanol 1:1 (v/v) and suspended in 1 mL of denaturing buffer. Each DNPH sample was read at 370 nm in a spectrophotometer against the corresponding HCl sample (blank), and the total carbonylation was calculated using a molar extinction coefficient of 22,000 M−1 cm−1 according to Levine et al.Citation35. The results are expressed as nmol carbonyl per millgram of protein.
Thiol peroxidase-like activity
The thiol peroxidase-like activity was examined to determine the possible mechanism by which functionalized alkyl-organotellurides A–D demonstrate antioxidant property. Free −SH groups were determined according to EllmanCitation36. The catalytic effect of alkyl-organotellurides A–D on the reduction of H2O2 by GSH was assessed using the rate of GSH oxidation. Alkyl-organotellurides A–D at different concentrations (0.1–100 μM) were incubated in the medium containing GSH (1.0 mM), with and without H2O2 (0.3 mM). At 120 min, aliquots of the reaction mixture (200 μL) were checked for the amount of GSH. The results are expressed as percentage of control.
δ-Aminolevulinic dehydratase activity
δ-ALA-D has been reported as being sensitive to pro-oxidants and heavy metalsCitation37. Since this enzyme is very sensitive to organochalcogen compounds, δ-ALA-D activity was used as a marker of toxicity. δ-ALA-D activity was assayed by the method of SassaCitation38 with some modifications. The principle of this method is based on enzyme incubation with an excess of δ-ALA. An aliquot of 200 μL of S1 was pre-incubated for 10 min at 37°C in the presence or absence of functionalized alkyl-organotellurides A–D at different concentrations (0.1–100 μM). Enzymatic reaction was initiated by adding the substrate, δ-ALA, to a final concentration of 2.2 mM in a medium containing 45 mM potassium phosphate buffer, pH 6.8. The incubation was carried out for 1 h at 37°C and stopped by adding trichloroacetic acid solution (10% TCA) with 10 mM HgCl2. Porphobilinogen (PBG), formed within a fixed time, was mixed with modified Ehrlich’s reagent, and the color developed was measured photometrically (555 nm) against a blank. The results are expressed as nmol PBG per milligram of protein per hour.
Thiol oxidase activity
The thiol oxidase activity was examined to determine the pro-oxidant property of functionalized alkyl-organotellurides A–D. The rate of thiol oxidation was determined in the presence of 50 mM Tris-HCl, pH 7.5, and alkyl-organotellurides A–D at different concentrations (0.1–100 μM). Incubation at 37°C was initiated by the addition of 1.0 mM reduced glutathione (GSH). Aliquots of the reaction mixture (200 μL) were checked for the amount of −SH groups at 412 nm. The rate of thiol oxidation was evaluated by measuring the disappearance of −SH groups. Free −SH groups were determined according to EllmanCitation36. Results are expressed as percentage of control.
Correlation between thiol oxidase and δ-ALA-D
Since the mechanism of δ-ALA-D inhibition may be linked to the oxidation of its sulfhydryl groupsCitation39, the correlation between δ-ALA-D activity and thiol oxidase property of functionalized alkyl-organotellurides A–D was performed. Through this relationship, we wanted to verify whether the mechanism of δ-ALA-D inhibition by organotellurides was related to the oxidation of cysteinyl residues located at the active site of the enzyme.
Protein determination
Protein was measured by the Coomassie blue method according to BradfordCitation40 using bovine serum albumin as standard.
Statistical analysis
Data were analyzed using a one-way analysis of variance (ANOVA), followed by Duncan’s multiple range test when appropriate. Results were considered significantly different at values of p < 0.05. The IC50 values (inhibitory concentrations) were calculated by the probit method using computer software SAS/STAT, and are reported as geometric means accompanied by their 95% confidence limits. Maximal inhibition (Imax) values were calculated at the most effective dose used. Pearson’s correlation coefficient was used for the estimation of correlation between parameters analyzed.
Results
Effect of functionalized alkyl-organotellurides A–D on lipid peroxidation induced by Fe2+/EDTA or SNP
Compound A at the concentration of 1.00 μM was effective in protecting against lipid peroxidation induced by Fe2+/EDTA in the liver (). Compounds D and B were effective at concentrations equal to or greater than 0.50 and 0.25 μM, respectively (). Compound C at the concentration of 0.01 μM reduced lipid peroxidation induced by Fe2+/EDTA (). According to the confidence limits of the IC50 values of compounds A–D, the IC50 values for functionalized alkyl-organotellurides followed the order: C ≤ B < D < A. Compounds A (IC50 = 2.90 μM), B (IC50 = 0.40 μM), C (IC50 = 0.30 μM), and D (IC50 = 0.68 μM) were more effective in reducing lipid peroxidation induced by Fe2+/EDTA in liver than the classical antioxidants, vitamin E (IC50 = 2.47 μM) and trolox (IC50 = 22.96 μM) (). The Imax values for compounds A, B, and C were around 70–80%, while for compound D the value was 50% (data not shown).
Figure 1. Effect of A (0.1–5 µM) (a), B (0.1–1 µM) (b), C (0.001– 1µM) (c), and D (0.1–1 µM) (d) on lipid peroxidation induced with Fe2+/EDTA. Data are reported as mean ± SD for four independent experiments, each one in duplicate. *, Denotes p < 0.05 as compared to the sample with Fe2+/EDTA (one-way ANOVA/Duncan). #, Denotes p < 0.05 as compared to control values (without Fe2+/EDTA and compounds) (one-way ANOVA/Duncan).
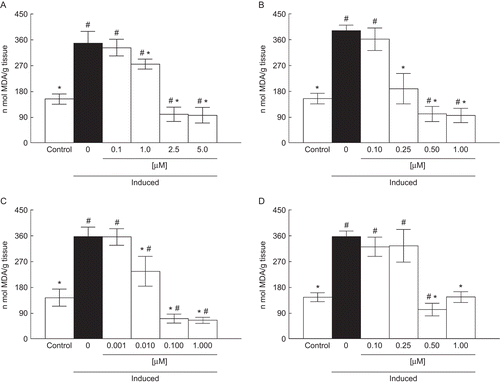
Table 1. Calculated IC50 values (µM) for lipid peroxidation and protein carbonyl content of functionalized alkyl-organotellurides in rat liver.
Compound A at the concentration equal to or greater than 0.50 μM reduced lipid peroxidation induced by SNP in the liver (). Compounds D and B were effective at concentrations of 0.25 and 0.10 μM, respectively (). Compound C at the concentration of 0.01 μM reduced lipid peroxidation induced by SNP (). According to the confidence limits of the IC50 values of compounds A–D, the IC50 values for functionalized alkyl-organotellurides followed the order: B ≤ C ≤ D < A. Compounds A (IC50 = 1.21 μM), B (IC50 = 0.21 μM), C (IC50 = 0.33 μM), and D (IC50 = 0.43 μM) were more effective in reducing lipid peroxidation induced by SNP in liver than vitamin E (IC50 = 2.97 μM) and trolox (IC50 = 27.20 μM) (). The Imax values for compounds A–D were around 70–80% (data not shown).
Figure 2. Effect of A (0.1–1 µM) (a), B (0.01–1 µM) (b), C (0.001–1 µM) (c), and D (0.1–1 µM) (d) on lipid peroxidation induced with SNP. Data are reported as mean ± SD for four independent experiments, each one in duplicate. *, Denotes p < 0.05 as compared to sample with SNP (one-way ANOVA/Duncan). #, Denotes p < 0.05 as compared to control values (without SNP and compounds) (one-way ANOVA/Duncan).
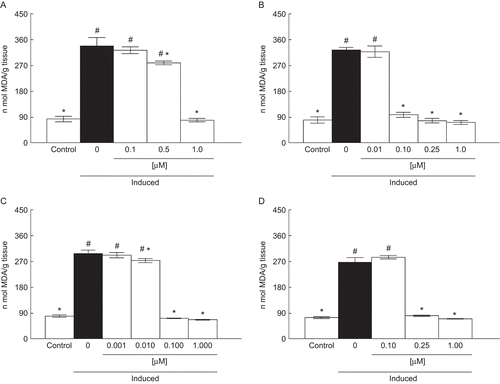
Effect of functionalized alkyl-organotellurides A–D on protein carbonyl content
Compounds A and C were effective in protecting against protein carbonylation induced by SNP in liver at the concentrations of 0.50 and 0.10 μM, respectively (). Compounds B and D, at the concentration of 0.25 μM, reduced protein carbonyl content (). According to the confidence limits of the IC50 values of compounds A–D, the IC50 values for functionalized alkyl-organotellurides followed the order: A = D ≥ B = C. Compounds A (IC50 = 0.81 μM), B (IC50 = 0.69 μM), C (IC50 = 0.71 μM), and D (IC50 = 0.83 μM) were more effective in reducing protein carbonyl formation in the liver than vitamin E (IC50 = 326.34 μM) and trolox (IC50 = 65.95 μM) (). The Imax values for compounds A–D were around 50% (data not shown).
Figure 3. Effect of A (0.1–1 µM) (a), B (0.1–1 µM) (b), C (0.001–1 µM) (c), and D (0.1–1 µM) (d) on protein carbonyl content. Data are reported as mean ± SD for four independent experiments, each one in duplicate. *, Denotes p < 0.05 as compared to the sample with SNP (one-way ANOVA/Duncan). #, Denotes p < 0.05 as compared to control values (without SNP and compounds) (one-way ANOVA/Duncan).
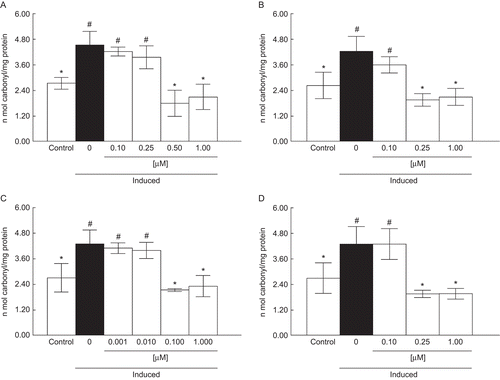
Effect of functionalized alkyl-organotellurides A–D on thiol peroxidase-like activity
Functionalized alkyl-organotellurides A–D at different concentrations (0.1–100 μM) did not present thiol peroxidase-like activity (data not shown).
Effect of functionalized alkyl-organotellurides A–D on δ-ALA-D activity
Functionalized alkyl-organotellurides A–D, at the concentration of 100 μM, reduced significantly the δ-ALA-D activity in rat liver homogenates (data not shown).
Effect of functionalized alkyl-organotellurides A–D on thiol oxidase activity
Functionalized alkyl-organotellurides A–D, at the concentration of 100 μM, increased the thiol oxidase activity (data not shown).
Correlation of thiol oxidase and δ-ALA-D activities
The results demonstrated a significant inverse correlation (r = 0.987, p < 0.005) between thiol oxidase and δ-ALA-D activity for compound A (). The r values for alkyl-organotellurides B, C, and D, which also presented significant inverse correlation between these parameters, were r = 0.916, r = 0.970, and r = 0.972, respectively (data not shown).
Discussion
The results of the present study showed that functionalized alkyl-organotellurides A–D, at low μM range, displayed an in vitro antioxidant activity in rat liver homogenates. Alkyl-organotellurides were able to prevent lipid peroxidation induced by Fe2+/EDTA and SNP in rat liver homogenates. It is known that iron complexes, such as Fe2+/EDTA, stimulate lipid peroxidation in cellsCitation41. SNP has been suggested to cause cytotoxicity through the release of cyanide and/or nitric oxideCitation42. In the present study, we demonstrated that low concentrations of functionalized alkyl-organotellurides were required to reduce lipid peroxidation induced by Fe2+/EDTA or SNP in rat liver homogenates. The IC50 values for functionalized alkyl-organotellurides A–D of lipid peroxidation induced by Fe2+/EDTA or SNP were close. In this way, we can infer that the antioxidant property of the studied alkyl-organotellurides did not depend on the inductor used. Moreover, compounds B, C, and D demonstrated higher efficiency in reducing lipid peroxidation induced by both inductors than compound A, as can be seen by the IC50 values. The IC50 values for functionalized alkyl-organotellurides followed the order C ≤ B < D < A for lipid peroxidation induced by Fe2+/EDTA or SNP. However, it is difficult to clearly explain why compound A displayed the lowest efficiency against lipid peroxidation. Based on chemical structures of alkyl-organotellurides A–D there is not an obvious explanation for the lowest antioxidant activity of compound A. Conversely, the literature data indicate that the chemical structure of organochalcogens has an important role in establishing their application as biological antioxidantsCitation43,Citation44.
Different from the results for lipid peroxidation, alkyl-organotellurides A, B, C, and D demonstrated similar effects on protein carbonyl content, suggesting that structural differences did not affect the antioxidant effect on proteins. Furthermore, carbonyl protein is the most used biomarker for protein oxidative damage, and it reflects the cellular damage induced by multiple forms of ROSCitation45. Taken together, the results of the current study demonstrated that functionalized alkyl-organotellurides, at low concentrations, displayed an antioxidant effect on lipid and protein oxidation.
Some authors have demonstrated that tellurium compounds are effective antioxidant agents, even more active than their selenium and sulfur analogsCitation1,Citation46. The antioxidant activity of diorganoyl tellurides and diselenidesCitation8 has been attributed, at least in part, to the glutathione peroxidase-like activity of these compoundsCitation10,Citation43. In contrast, functionalized alkyl-organotellurides A–D did not display glutathione peroxidase-like activity, discarding that the antioxidant activity of these compounds could be related to this mechanism. However, other mechanisms could be involved in the antioxidant property of the studied functionalized alkyl-organotelluridesCitation11. In fact, more studies are necessary to elucidate the exact mechanism involved in the antioxidant effect caused by alkyl-organotellurides A–D.
Although functionalized alkyl-organotellurides presented an antioxidant effect, the literature data report that they induce toxicityCitation7. In this way, previous works have indicated that alkyl-organotellurides inhibit cysteinyl-containing enzymes, such as Na+/K+ ATPaseCitation47, δ-ALA-DCitation37,Citation39, and squaleno monooxygenaseCitation48. Thus, the toxicity of organotellurium compounds can be related to interaction with the thiol groups of important biomoleculesCitation8, the replacement of selenium in selenoproteins, such as thioredoxinCitation1, and the capacity of tellurium compounds to induce the formation of ROSCitation49.
Surprisingly, the present study demonstrated that functionalized alkyl-organotellurides a–d were good antioxidants, and affected δ-ALA-D activity exclusively at high concentrations. In fact, δ-ALA-D is a group of sulfhydryl-containing enzymesCitation50, being extremely sensitive to the presence of pro-oxidant elements, and numerous compounds that oxidize sulfhydryl groups, such as selenium and tellurium compoundsCitation39,Citation51,Citation52, modify its activity. Particularly, organotellurium compounds may interact with thiol groups of this enzyme, oxidizing sulfhydryl groups of the active site, changing the configuration of the enzyme and impairing its activity. A previous study from our research group reported that organochalcogen compounds, at low concentrations, reduced δ-ALA-D activityCitation37,Citation39,Citation51. Conversely, we demonstrated here that functionalized alkyl-organotellurides a–d did not inhibit δ-ALA-D activity at concentrations for which they displayed antioxidant activity. In fact, these compounds inhibited enzyme activity at the concentration of 100 μM, a concentration 100 times higher than those at which alkyl-organotellurides presented the antioxidant effect. This result highlights this class of compounds as good antioxidant agents with low potential toxicity, demonstrated in rat liver homogenates in vitro. The thiol oxidase activity is an estimation of the cytotoxicity of moleculesCitation8. Organotellurides A–D showed thiol oxidase activity only at the concentration of 100 μM, indicating an advantage regarding the possible toxicological properties of these compounds. Recently, our research group reported that the mechanism involved in δ-ALA-D inhibition caused by telluroacetylenes in vitro is related to their ability to oxidize sulfhydryl groups from the enzyme structureCitation52. In conformity, our results demonstrated an inverse correlation between thiol oxidase and δ-ALA-D activities, meaning that δ-ALA-D activity decreases as the compound’s ability to oxidize sulfhydryl groups increases. Therefore, at the concentration for which compounds have the thiol oxidase property, they also inhibit δ-ALA-D activity, suggesting that oxidation of enzyme sulfhydryl groups could be involved in the inhibition caused by functionalized alkyl-organotellurides. Thus, similar to other organochalcogensCitation7,Citation39,Citation51–53, functionalized alkyl-organotellurides oxidize thiol groups to disulfidesCitation8, clearly demonstrated by the thiol oxidase activity and the results obtained with δ-ALA-D activity in rat liver homogenates in vitro.
In conclusion, the results of the present investigation support the in vitro antioxidant effect of functionalized alkyl-organotellurides A–D in rat liver homogenates. Of particular importance, functionalized alkyl-organotellurides A–D inhibited δ-ALA-D activity at a concentration 100 times higher than those for which they displayed the antioxidant effect. Additional studies are necessary to investigate the possible antioxidant effect of these compounds in an in vivo model of oxidative damage.
Declaration of interest
The financial support by UFSM (FIPE), FAPERGS, CAPES, FAPESP, and CNPq is gratefully acknowledged.
References
- Engman L, Kanda T, Gallegos A. Water-soluble organotellurium compounds inhibit thioredoxin reductase and the growth of human cancer cells. Anticancer Drug Des 2000;15:323–30.
- Nogueira CW, Quinhones EB, Jung EAC, Zeni G, Rocha JBT. Anti-inflammatory and antinociceptive activity of diphenyl diselenide. Inflamm Res 2003;63:52–6.
- Cunha LOR, Urano ME, Chagas JR, Almeida PC, Bincoletto C, Tersariol ILS, et al. Tellurium-based cysteine protease inhibitors: evaluation of novel organotellurium (IV) compounds as inhibitors of human cathepsin B. Bioorg Med Chem Lett 2005;15:755–60.
- Savegnago L, Jesse CR, Pinto LG, Rocha JBT, Barancelli DA, Nogueira CW. Diphenyl diselenide exerts antidepressant-like and anxiolytic-like effects in mice: involvement of l-arginine-nitric oxide-soluble guanylate cyclase pathway in its antidepressant-like action. Pharmacol Biochem Behav 2008;88:418–26.
- Wöhler F. Ann Chem 1840;35:111.
- Rooseboom M, Vermeulen NPE, Durgut F: Comparative study on the bioactivation mechanisms and cytotoxicity of Te-phenyl-L-tellurocysteine, Se-phenyl-L-selenocysteine, and S-phenyl-L-cysteine. Chem Res Toxicol 2002;15:1610–18.
- Meotti FC, Borges VC, Zeni JBT, Nogueira CW. Potential renal and hepatic toxicity of diphenyl diselenide, diphenyl ditelluride and Ebselen for rats and mice. Toxicol Lett 2003;143:9–16.
- Nogueira CW, Zeni G, Rocha JBT. Organoselenium and organotellurium compounds: toxicology and pharmacology. Chem Rev 2004;104:6255–85.
- Engman L, Al-Maharik N, McNaughton M. Thioredoxin reductase and cancer cell growth inhibition by organotellurium compounds that could be selectively incorporated into tumor cells. Bioorg Med Chem 2003;11:5091–100.
- Ren X, Xue Y, Zhang K, Liu J, Luo G, Zheng J, et al. A novel dicyclodextrinyl ditelluride compound with antioxidant activity. FEBS Lett 2001;507:377–80.
- Kanski J, Drake J, Aksenova M. Antioxidant activity of the organotellurium compound 3-[4-(N,N-dimethylamino)benzenetellurenyl] propanesulfonic acid against oxidative stress in synaptosomal membrane systems and neuronal cultures. Brain Res 2001;911:12–21.
- Ávila DS, Beque MC, Folmer V, Braga AL, Zeni G, Nogueira CW, et al. Diethyl 2-phenyl-2-tellurophenyl vinylphosphonate: an organotellurium compound with low toxicity. Toxicology 2006;224:100–7.
- Savegnago L, Borges VC, Alves D, Jessé CR, Rocha JBT, Nogueira CW. Evaluation of antioxidant activity and potential toxicity of 1-buthyltelurenyl-2-methylthioheptene. Life Sci 2006;79:1546–52.
- Ávila DS, Gubert P, Corte CLD, Alves D, Nogueira CW, Rocha JBT, et al. A biochemical and toxicological study with diethyl 2-phenyl-2-tellurophenyl vinylphosphonate in a sub-chronic intraperitoneal treatment in mice. Life Sci 2007;80:1865–72.
- Arteel GE, Sies H. The biochemistry of selenium and the glutathione system. Environ Toxicol Pharmacol 2001;10:153–8.
- Lecanu L, Greeson J, Papadopoulos V. Beta-amyloid and oxidative stress jointly induce neuronal death, amyloid deposits, gliosis, and memory impairment in the rat brain. Pharmacology 2006;76:19–33.
- Khan TH, Sultana S. Antioxidant and hepatoprotective potential of Aegle marmelos Correa. against CCl4-induced oxidative stress and early tumor events. J Enzyme Inhib Med Chem 2009;24:320–7.
- Ercan S, Cakmak A, Kosecik M. Total oxidant and antioxidant levels and oxidative stress index levels in children with congenital heart disease. Acta Paediatr 2008;97:86–7.
- Nabben M, Hoeks J. Mitochondrial uncoupling protein 3 and its role in cardiac-and skeletal muscle metabolism. Phys Behav 2008;94:259–69.
- Augustyniak K, Zavodnik I, Palecz D, Szosland K, Bryszewska M. The effect of oxidizing agent and diabetes mellitus on the human red blood cell membrane potential. Clin Biochem 1996;29:283–6.
- Gey KF. The relationship of antioxidant status and the risk of cancer and cardiovascular disease: a critical evaluation of observational data. In: Nohl H, Esterbauer H, Rice-Evans C, eds. Free Radicals in the Environment, Medicine and Toxicology. London: Richelieu Press, 1994:181–219.
- Wang BB, Wang J, Zhou SR. The association of mitochondrial aldehyde dehydrogenase gene (ALDH2) polymorphism with susceptibility to late-onset Alzheimer’s disease in Chinese. J Neurol Sci 2008;268:172–5.
- Ayhan-Kilcigil G, Kus C, Coban T, Can-Eke B, Iscan M. Antioxidant and neuroprotective effects of synthesized sintenin derivatives. J Enzyme Inhib Med Chem 2004;19:129–35.
- Yang LX, Zhang LJ, Huang KX, Kun Li X, Hu LH, Wang XY, et al. Antioxidant and neuroprotective effects of synthesized sintenin derivatives. J Enzyme Inhib Med Chem 2009;24:425–31.
- Dos Santos AA, Comasseto JV. β-Butyltellanyl carbonyl compounds: a useful source of masked metal homoenolates. J Braz Chem Soc 2005;16:511–13.
- Comasseto JV, Gariani RA, Princival JL, Dos Santos AA, Zinn FK. Hydrochalcogenation of activated olefines. Synthesis of functionalized dialkylchalcogenides. Organomet Chem 2008;693:2929–36.
- Princival JL, Barros SMG, Comasseto JV, Dos Santos AA. β-Butyltelluro-2-butanol: a route to reactive 1,4-dianion intermediates. Tetrahedron Lett 2005;46:4423–5.
- Dos Santos AA, Ferrarini RS, Princival JL, Comasseto JV. Organotellurides as a source of organometallics: application in the synthesis of (+/-)-frontalin. Tetrahedron Lett 2006;47:8933–5.
- Comasseto JV, Dos Santos AA. Organotellurides as precursors of reactive organometallics. Phosphorus Sulfur Silicon Relat Elem 2008;183:939–47.
- Ferrarini RS, Princival JL, Comasseto JV, Dos Santos AA. A concise enantioselective synthesis of (+)-endo-brevicomin accomplished by a tellurium/metal exchange reaction. Braz Chem Soc 2008;19:811–12.
- Wendler EP, Dos Santos AA. The use of butyl organotellurides in the synthesis of natural bioactive compounds. Synlett 2009:1034–40.
- Bigdeli MA, Alvi Nikje MM, Jafari S, Heravi MM. Regioselective synthesis of syn-oximes using 3Å molecular sieves in a solventless system. J Chem Res Synop 2002;1:20–1.
- Ohkawa H, Ohishi N, Yagi K. Assay for lipid peroxides in animal tissues by thiobarbituric acid reaction. Anal Biochem 1979;95:351–8.
- Yan LJ, Traber MG, Packer L. Spectrophotometric method for determination of carbonyls in oxidatively modified apolipoprotein B of human low-density lipoproteins. Anal Biochem 1995;228:349–51.
- Levine RL, Garland D, Oliver CN. Determination of carbonyl content in oxidatively modified proteins. Methods Enzymol 1990;186:464–78.
- Ellman GL. Tissue sulfhydryl groups. Arch Biochem Biophys 1959;82:70–7.
- Nogueira CW, Borges VC, Zeni G, Rocha JBT. Organochalcogens effects on δ-aminolevulinate dehydratase activity from human erythrocytic cells in vitro. Toxicology 2003;191:169–78.
- Sassa S. Delta aminolevulinic acid dehydratase assay. Enzyme 1982;28:133–45.
- Barbosa NBV, Rocha JBT, Zeni G, Emanuelli T, Beque MC, Braga AL. Effect of organic selenium on δ-aminolevulinate dehydratase from liver, kidney, and brain of adult rats. Toxicol Appl Pharmacol 1998;149:243–53.
- Bradford MM. Rapid and sensitive method for quantitation of microgram quantities of protein utilizing principle of protein-dye binding. Anal Biochem 1976;72:248–54.
- Gogvadze V, Walter PB, Ames BN. The role of Fe2+-induced lipid peroxidation in the initiation of the mitochondrial permeability transition. Arch Biochem Biophys 2003;414:255–60.
- Bates JN, Baker MT, Guerra R, Harrison DG. Nitric oxide generation from nitroprusside by vascular tissue: Biochem Pharmacol 1990;42:S157–65.
- Meotti FC, Stangherlin EC, Zeni G, Nogueira CW, Rocha JBT. Protective role of aryl and alkyl diselenides on lipid peroxidation. Environ Res 2004;94:276–82.
- Prigol M, Wilhelm EA, Schneider CC, Nogueira CW. Protective effect of unsymmetrical dichalcogenide, a novel antioxidant agent, in vitro and an in vivo model of brain oxidative damage. Chem Biol Interact 2008;176:129–36.
- Dalle-Donne I, Aldini G, Carini M, Colombo R, Rossi R, Milzani A. Protein carbonylation, cellular dysfunction, and disease progression. J Cell Mol Med 2006;10:389–406.
- Wieslander E, Engman L, Svensjo E, Erlansson M, Johansson U, Linden M, et al. Antioxidative properties of organotellurium compounds in cell system. Biochem Pharmacol 1998;55:573–84.
- Borges VC, Rocha JBT, Nogueira CW. Effect of diphenyl diselenide, diphenyl ditelluride and ebselen on cerebral Na+, K+-ATPase activity in rats. Toxicology 2005;215:191–7.
- Laden BP, Porter TD. Inhibition of human squalene monooxygenase by tellurium compounds: evidence of interaction with vicinal sulfhydryls. J Lipid Res 2001;42:235–40.
- Chen F, Vallyathan V, Castranova V, Shi X. Cell apoptosis induced carcinogenic metals. Mol Cell Biochem 2001;221:183–8.
- Gibson KD, Neuberger A, Scott JJ. The purification and properties of delta-aminolevulinic acid dehydratase. Biochem J 1955;61:618–28.
- Luchese C, Zeni G, Rocha JBT, Nogueira CW, Santos FW. Cadmium inhibits δ-aminolevulinate dehydratase from rat lung in vitro: interaction with chelating and antioxidant agents. Chem Biol Interact 2007;165:127–37.
- Souza ACG, Luchese C, Neto JSS, Nogueira CW. Antioxidant effect of a novel class of telluroacetylene compounds: studies in vitro and in vivo. Life Sci 2009;84: 351–7.
- Maciel EN, Bolzan RC, Braga AL, Rocha JBT. Diphenyl diselenide and diphenyl ditelluride differentially affect delta-aminolevulinate dehydratase from liver, kidney, and brain of mice. J Biochem Mol Toxicol 2000;310: 319–14.