Abstract
In this study, two Pt(II) and three Pt(IV) complexes with the structures of [PtL2Cl2] (1), [PtL2I2] (2), [PtL2Cl2(OH)2] (3), [PtL2Cl2(OCOCH3)2] (4), and [PtL2Cl4] (5) (L = benzimidazole as carrier ligand) were synthesized and evaluated for their in vitro antiproliferative activities against the human MCF-7, HeLa, and HEp-2 cancer cell lines. The influence of compounds 1–5 on the tertiary structure of DNA was determined by their ability to modify the electrophoretic mobility of the form I and II bands of pBR322 plasmid DNA. The inhibition of BamH1 restriction enzyme activity of compounds 1–5 was also determined. In general, it was found that compounds 1–5 were less active than cisplatin and carboplatin against MCF-7 and HeLa cell lines (except for 1, which was found to be more active than carboplatin against the MCF-7 cell line). Compounds 1 and 3 were found to be significantly more active than cisplatin and carboplatin against the HEp-2 cell line.
Introduction
Platinum-based drugs such as cisplatin, carboplatin, and oxaliplatin are widely used against various solid tumors including genitourinary, colorectal, and non-small cell lung cancersCitation1. In general, the platinum-based drugs suffer from two main disadvantages: chemoresistance to the drugs can occur and, second, they are non-selective toward cancer cells, which lead to severe toxic side effects, primarily kidney toxicity and neurotoxicityCitation2.
Although there is some evidence to suggest that other biological targets may be important in the mechanism, it is generally accepted that DNA is the primary biological target of the platinum drugsCitation3,Citation4. Numerous studies show that, in DNA, cisplatin forms ~90% intrastrand cross-links (CLs) between neighboring purine bases (1,2-GG or 1,2-AG intrastrand CLs), and remaining lesions are intrastrand CLs between purine bases separated by a third base, interstrand CLs, and monofunctional adductsCitation5.
Cellular resistance to cisplatin is largely attributed to up-regulation of DNA repair and damage tolerance pathways, lowered intracellular accumulation, and inactivation by thiol-containing reductants such as glutathione and metallothionein, and alterations in proteins involved in apoptosisCitation6. One strategy to overcome cisplatin resistance is to design new platinum complexes that specifically deal with some or even all of the resistance mechanismsCitation7.
In addition to square-planar Pt(II) complexes, an attempt has also been made recently to synthesize octahedral Pt(IV) complexes, since some of these complexes are toxic to tumors that are resistant to cisplatinCitation8. The antitumor activity of Pt(IV) complexes has been suggested to require in vivo reduction to the kinetically more labile, and therefore reactive, Pt(II) derivativesCitation9. On the other hand, there are a few papers reporting that Pt(IV) complexes can bind to DNA and RNA fragments without being reducedCitation10,Citation11.
In the literature it is reported that the reduction potentials of diam(m)ine Pt(IV) complexes are dependent on the nature of the axial and equatorial ligands, but axial ligands generally exert the stronger influenceCitation12,Citation13. The axial ligands of Pt(IV) complexes offer a unique ability in drug design to modify the pharmacokinetic parameters of a prodrug, including the rate of reduction, the lipophilicity, molecular targeting, and microenvironmental targeting, in order to reduce side effects, increase activity, and target tumor sites, or the axial ligands themselves can confer additional cytotoxicity upon releaseCitation12,Citation14.
Although no platinum(IV) complex is an approved drug yet, some Pt(IV) complexes have shown sufficient promise to enter clinical trials: iproplatin, [cis,trans,cis-[PtCl2(OH)2(isopropylamine)2], tetraplatin, [PtCl4(cyclohexane-1,2-diamine)], and satraplatin, cis,trans-[PtCl2(OAc)2(NH3) cyclohexylamine] have been tested in clinical trialsCitation15. At present, the most interesting candidate is satraplatin, (formerly JM216), which is in advanced phase III clinical trials showing activity in hormone-refractory prostate cancer in combination with prednisone as second-line chemotherapyCitation16.
In previous studies, taking into consideration the fact that variations in the chemical structure of the ammine groups of the cisplatin can have significant effects on the cytotoxic activity and toxicity of platinum complexes, we synthesized some Pt(II) and also Pt(IV) complexes with 2-substituted benzimidazole ligandsCitation17–24. It was determined that some of these platinum complexes have in vitro cytotoxic activities on RDCitation19, HeLaCitation21,Citation22,Citation24, HEp-2Citation23, and MCF-7Citation20–22,Citation24 cell lines.
In the present study, with the aim of determining the effect of axial and equatorial ligand variation on the cytotoxic activities of the Pt(II) and Pt(IV) complexes, we synthesized some Pt(II) and Pt(IV) complexes with the structures of [PtL2Cl2] (1), [PtL2I2] (2), [PtL2Cl2(OH)2] (3), [PtL2Cl2(OCOCH3)2] (4), and [PtL2Cl4] (5) (L = benzimidazole as carrier ligand) and tested for their preliminary in vitro antiproliferative activities against the human MCF-7 breast, HeLa cervix, and HEp-2 larynx carcinoma cell lines. The plasmid DNA interaction and the inhibition of BamH1 restriction enzyme activity of the compounds 1–5 were also studied.
Experimental
Materials and instruments
All chemicals and solvents used in the synthesis were purchased from Merck or Aldrich Chemical Co. Cisplatin and carboplatin used in the cytotoxicity test were purchased from Sigma Co. Infrared (IR) spectra were recorded on KBr pellets and Nujol mulls on a Mattson 1000 FTIR spectrometer in the range of 4000–200 cm−1. For the region 400–200 cm−1 the samples were prepared as Nujol mulls on CsI windows. Elemental analyses were performed with a Leco 932 CHNS analyser, and 1H nuclear magnetic resonance (NMR) spectra were recorded in dimethylsulfoxide (DMSO)-d6 (Merck) on a Varian Mercury 400 MHz FT NMR spectrometer at the Central Laboratory of the Faculty of Pharmacy, Ankara University (Ankara, Turkey). Thin-layer chromatography (TLC) was performed on aluminum plates pre-coated with Merck silica gel 60 F254. Plates were visualized by ultraviolet (UV) light, Dragendorff reagent, or iodine vapor.
Synthesis of ligand, Pt(II) and Pt(IV) complexes
Benzimidazole (L)
L, used as carrier ligand in the structure of 1–5, was prepared according to the published procedureCitation25. Mp 170°C, (169–170°C26); yield 59%. 1H NMR (400 MHz, DMSO-d6) δ 12.40 (broad s, 1H, N-H, exchangeable with D2O), 8.22 (s, 1H, ArH), 7.58–7.60 (dd, J = 9.2 and 2.8 Hz, 2H, ArH), 7.17–7.20 (dd, J = 9.2 and 2.8 Hz, 2H, ArH). IR (KBr, cm−1) ν 3300–2300 (N-H) cm−1.
[Dichloro-bis(benzimidazole)platinum(II)] [PtL2Cl2] (1)
To a stirred aqueous solution of K2PtCl4 (0.830 g, 2.00 mmol) was added a solution of benzimidazole (L) (0.472 g, 4.00 mmol) in an ethanol–water mixture (8:12 mL) dropwise over 2 h at room temperature. The pH was adjusted to ~7 and kept constant with the addition of 0.1 M NaHCO3. The reaction mixture, protected from light, was heated at 60°C for 2 days. After that time the mixture was cooled to room temperature. The resulting crude precipitate was filtered off and purified by repeated washing with small portions of water, ethanol, and diethylether, and dried in vacuo to yield 0.755 g (75.16%). 1H NMR (400 MHz, DMSO-d6) δ 13.44 (s, 2H, 2 × N-H, exchangeable with D2O), 8.84 (s, 2H, 2 × ArH), 7.80–7.79 (d, J = 7.2 Hz, 2H, ArH), 7.50–7.48 (d, J = 7.2 Hz, 2H, ArH), 7.25–7.18 (m, 4H, 2 × ArH). IR (KBr, cm−1) ν 3287 (N-H), 326 (Pt-Cl) cm−1. Anal. Calcd. for C14H12Cl2N4Pt: C, 33.48; H, 2.41; N, 11.15. Found: C, 33.46; H, 2.64; N, 11.09%.
[Diiodo-bis(benzimidazole)platinum(II)] [PtL2I2] (2)
K2PtCl4 (0.622 g, 1.50 mmol) and KI (0.996 g, 6.00 mmol) were dissolved in water (15 mL) and stirred at 60°C for 45 min. Then a solution of benzimidazole (L) (0.331 g, 2.80 mmol) in an ethanol–water mixture (6:10 mL) was added dropwise over 2 h at room temperature to the resulting K2PtI4. The reaction mixture, protected from light, was heated at 60°C for 2 days. The resulting crude yellowish precipitate was filtered off and purified by repeated washing with small portions of water, ethanol, and diethylether, and dried in vacuo to yield 0.410 g (39.92%). 1H NMR (400 MHz, DMSO-d6) δ 13.38 (broad s, 2H, 2 × N-H, exchangeable with D2O), 8.98 (s, 2H, 2 × ArH), 8.05–8.03 (m, 2H, 2 × ArH), 7.67–7.64 (m, 2H, 2 × ArH), 7.38–7.20 (m, 4H, 2 × ArH). IR (KBr, cm−1) ν 3310 (N-H) cm−1. Anal. Calcd. for C14H12I2N4Pt: C, 24.54; H, 1.77; N, 8.18. Found: C, 24.13; H, 2.00; N, 8.41%.
[Dichloro-dihydroxy-bis(benzimidazole)platinum(IV)] [PtL2Cl2(OH)2] (3)
To a suspension of 1 (0.115 g, 0.23 mmol) in water (15 mL) was added an aqueous solution of 30% H2O2 (1 mL), and the reaction mixture, protected from light, was stirred at 60°C for 5 days. The resulting crude yellow precipitate was filtered off and purified by repeated washing with small portions of water, ethanol, acetone, and diethylether, and dried in vacuo to yield 0.049 g (39.72%). 1H NMR (400 MHz, DMSO-d6) δ 13.50 (s, 2H, 2 × N-H, exchangeable with D2O), 8.92 (s, 1H, 2 × ArH), 8.70 (s, 1H, 2 × ArH), 7.90–7.84 (m, 1H, 2 × ArH), 7.68–7.58 (m, 2H, 2 × ArH), 7.33–7.28 (m, 4H, 2 × ArH), 7.13–7.09 (m, 1H, ArH), 2.59 (s, 2H, Pt-OH, exchangeable with D2O). IR (KBr, cm−1) ν 3500 (N-H), 3445 (PtO-H), 540 (Pt-O), and 348 (Pt-Cl) cm−1. Anal. Calcd. for C14H14Cl2N4O2Pt: C, 31.36; H, 2.63; N, 10.45. Found: C, 31.30; H, 2.29; N, 10.03%.
[Diacetato-dichloro-bis(benzimidazole)platinum)(IV)] [PtL2Cl2(OCOCH3)2] (4)
1 (0.452 g, 0.90 mmol) was dissolved in 1.37 mL acetic acid, 0.23 mL acetic acid anhydride, and 0.12 mL of 30% H2O2. The reaction mixture, protected from light, was stirred at room temperature for 6 days. The resulting crude precipitate was filtered off and purified by repeated washing with small portions of water, ethanol, acetone, and diethylether, and dried in vacuo to yield 0.130 g (23.28%). 1H NMR (400 MHz, DMSO-d6) δ 13.90 (s, 2H, 2 × N-H, exchangeable with D2O), 8.77 (s, 2H, 2 × ArH), 7.61–7.59 (d, J =8.11 Hz, 2H, 2 × ArH), 7.39–7.37 (d, J =8.41 Hz, 2H, 2 × ArH), 7.27–7.23 (m, 2H, 2 × ArH), 7.07–7.03 (m, 2H, 2 × ArH), 1.89 (s, 6H, 2 × OCOCH3). IR (KBr, cm−1) ν 3557 (N-H), 1664(C=O), and 340 (Pt-Cl) cm−1. Anal. Calcd. for C18H18Cl2N4O4Pt: C, 34.85; H, 2.92; N, 9.03. Found: C, 34.49; H, 2.62; N, 9.20%.
[Tetrachloro-bis(benzimidazole)platinum(IV)] [PtL2Cl4] (5)
4 (0.062 g, 0.10 mmol) was dissolved in aqueous 15% HCl (5 mL). The reaction mixture, protected from light, was stirred at room temperature for 3 days. The resulting crude yellow precipitate was filtered off and purified by repeated washing with small portions of water, ethanol, acetone, and diethylether, and dried in vacuo to yield 0.035 g (61.07%). 1H NMR (400 MHz, DMSO-d6) δ 13.80 (s, 2H, 2 × N-H, exchangeable with D2O), 8.88 (s, 2H, 2 × ArH), 7.73–7.66 (m, 2H, 2 × ArH), 7.43–7.23 (m, 4H, 2 × ArH), 7.18–7-06 (m, 2H, 2 × ArH). IR (KBr, cm−1) ν 3510 (N-H), 335 (Pt-Cl) cm−1. Anal. Calcd. for C14H12Cl4N4Pt: C, 29.34; H, 2.11; N, 9.77. Found: C, 29.29; H, 2.26; N, 9.52%.
Preliminary cytotoxicity test
Cell lines and growth conditions
Cisplatin and carboplatin were obtained from Sigma with a purity of more than 99.9%. The human MCF-7 breast, HeLa cervix, and HEp-2 larynx cancer cell lines used in this study were obtained from the Department of Virology, Faculty of Veterinary Medicine, University of Ankara. The cells were grown in Dulbecco’s (Seromed, Germany) minimal essential medium (DMEM) enriched with 10% fetal calf serum (FCS) (Biochrom, Germany), 100 mg/mL streptomycin, and 100 IU/mL penicillin in a humidified atmosphere of 5% CO2 at 37°C. The cells were harvested using trypsin (Bibco Life Technologies, UK)/Versene (0.05:0.02%) solution. Mycoplasma contamination was routinely monitored, and only Mycoplasma-free cultures were used.
In vitro chemosensitivity assay
The preliminary in vitro testing of compounds 1–5 on antitumor activity was carried out on human MCF-7 breast, HeLa cervix, and HEp-2 larynx cancer cells according to a previously published microtiter testCitation27. Briefly, the cells were seeded into 96-well plates (Greiner GmbH, Germany) in a volume of 100 µL to give 18–22 cells/microscopic area. After attachment to the culture surface, the cells were incubated in an atmosphere containing 5% CO2 at 37°C for 24 h. At the end of this period the growth medium was carefully removed by suction, and 100 µL of fresh medium was added into each well. The medium used contained an adequate volume of a stock solution of the respective compound in order to obtain the desired test concentration (1, 5, 10, 20, 40, and 80 µM; solvent, dimethylformamide (DMF); the complexes tested were added to the culture medium such that final DMF was 0.1% (v/v)). Sixteen wells were used for each complex (1–5, and reference compounds cisplatin and carboplatin) tested at individual concentrations, while 16 wells were reserved for the cell culture control, which contained the corresponding amount of DMF. After 72 h of incubation at 37°C, the medium was removed and the cells were fixed with 100 µL of 1% glutardialdehyde in phosphate-buffered saline (PBS) per well for 25 min. The fixative was replaced by 150 µL PBS/well and the plates were stored in a refrigerator (4°C). Cell biomass was determined by a crystal violet staining techniqueCitation28. Absorbance was measured at 492 nm using a Titertek Multiscan plus MKII Autoreader. The results correspond to three independent experiments.
IC50 values of the complexes 1–5 and reference compounds cisplatin and carboplatin were calculated (using Prism4 GraphPad software) from the dose–survival curves for the growth inhibition of all cell lines.
All cytotoxicity tests were performed as three independent experiments.
Interaction with pBR322 plasmid DNA
Cisplatin, ethidium bromide, and agarose, and plasmid DNA pBR322 and enzyme BamH1, were purchased from Sigma and Promega, respectively.
The interaction of cisplatin and synthesized compounds 1–5 with pBR322 plasmid DNA (Promega, cat. no: D1511) was studied by agarose gel electrophoresis based on a method described by StellwagenCitation29. Stock solutions of the tested substances in DMF were prepared and used within 1 h. The final amount of DMF never exceeded 0.1%. pBR322 plasmid DNA (0.15 µg/mL) was incubated in the presence of increasing concentrations of 1–5 and cisplatin ranging from 0.625 to 160 µM and 0.625 to 10 µM, respectively, in a shaking water bath at 37°C for 4 h. Five microliters of drug–plasmid DNA mixture was loaded onto 1.5% agarose gel and electrophoresis was carried out under TAE (Tris base, acetic acid, and ethylenediaminetetraacetic acid (EDTA)) buffer for 2 h at 5 V/cm. At the end of electrophoresis, the gel was stained in the same buffer containing ethidium bromide (0.5 mg/mL) and visualized under UV transillumination using a gel documentation system (Vilber Lourmat, Marne la Vallée, France). The experiments were repeated three times.
Restriction endonuclease reaction with BamH1 enzyme
In this series of experiments, a set of synthesized compounds 1–5 and cisplatin–plasmid DNA mixtures the same as that described previously was first incubated for 4 h in a shaking water bath at 37°C and then subjected to enzyme BamH1 digestion. To each 10 µL 1–5/cisplatin–plasmid DNA mixture was added 3 µL of 10 × RE buffer followed by the addition of 0.3 µL BamH1 (3 U). The mixtures were left in a shaking water bath at 37°C for 1.5 h, at the end of which the reaction was terminated by rapid cooling. Following the digestion reaction, products were separated on 1.5% agarose gel, and then the gel was stained with ethidium bromide and visualized under UV transillumination using a gel documentation system (Vilber Lourmat) to detect digestion.
Result and discussion
Chemistry
The carrier ligand benzimidazole (L) was prepared according to the Phillips methodCitation25 as shown in , and its melting point was in accordance with the literatureCitation26.
The Pt(II) and Pt(IV) compounds 1–5 were synthesized and characterized by elemental analysis, IR, and 1H NMR spectra (). Among the compounds synthesized, 1, which was reported previously by usCitation20, was synthesized in this study as reported previously. The elemental analysis data for each complex were in good agreement with the empirical formula proposed.
The IR spectrum of L showed a very broad band in the region 3300–2300 cm−1 due to the imidazole N-H. The Pt(II) complexes exhibited N-H stretching bands centered at 3310 and 3287 cm−1 sharper than that of the free ligand, due to the breaking of tautomerism, indicating that the N-H group was not involved in the coordinationCitation26,Citation30. According to the kinetic trans effectCitation31, the synthesis method used is expected to yield complexes with cis geometry. In the far-IR region of the complexes 1, 3–5 spectra, a new broad band with a half-width of about 30 cm−1 appeared, assigned to ν (Pt-Cl) centered at ~337 cm−1. It is well known that cis-dichloro complexes should show two bands of medium intensity because the vibrations are additive, but in a lot of cases the second band is only a shoulderCitation32. Although no shoulder was apparently observable at the Pt-Cl stretching band of compounds 1,3-5 the broad nature of the band suggested the presence of overlapping bands in this domain. The Pt-I band of the diiodo complex 2 should show at 195–183 cm−1 in the far-IR region of the complex’s spectrumCitation33, but the ν (Pt-I) stretching band for 2 could not be measured on the spectrophotometer used.
The IR spectra of the Pt(IV) complexes showed some characteristic changes when compared to those of the Pt(II) complexes and the free ligand spectrum. Pt-Cl stretching vibrations were shifted (~15 cm−1) toward higher frequencies in the spectra of Pt(IV) complexes 3–5 upon oxidationCitation34. Pt(IV) complexes 3–5 exhibited only one ν (Pt-Cl) absorption, which is consistent with the literatureCitation35. In the IR spectra of 3–5, N-H stretching vibrations appeared at the highest frequencies from 3557 to 3500 cm−1. Being influenced by the oxidation states of the central metal, the N-H vibrations in the Pt(IV) compounds were broad in comparison with that in the Pt(II) compound 1Citation22,Citation36. The hydroxyplatinum(IV) complex 3, which was obtained by the oxidation reaction of 1 with hydrogen peroxide, was readily identified by its characteristic PtO-H stretching band at 3445 cm−1 and Pt-O stretching band at 540 cm−1 Citation22,Citation37,Citation38.
The insolubility of the complexes in the other organic solvents made it necessary to record 1H NMR spectra in DMSO-d6. All 1H NMR measurements were recorded immediately, in order to avoid the ligand exchange reaction between the platinum complexes synthesized and DMSO-d6. The 1H-NMR spectral data of the ligand and the complexes are presented above in the “Experimental” section. The 1H NMR spectra of all complexes were consistent with their corresponding protons, both in the chemical shifts and in the number of hydrogensCitation39. The spectra of the complexes compared to those of the free ligand showed considerable differences. The large downfield shifts in the imidazole N-H signal in the spectra of all complexes with respect to their ligands are a result of an increase in the N-H acid character after platinum binding. In all the complexes the aromatic proton resonances were observed in two main groups of lines. Two protons of the lower field pair were shifted downfield considerably, while the others were shifted slightly. It was assumed that the protons nearest to the tertiary nitrogen of the imidazole ring were the most deshielded, and would be observed at a lower field due to coordination of the benzimidazole ring to platinum through the -N= group.
Biological evaluation
The preliminary in vitro antiproliferative activities of compounds 1–5 and cisplatin and carboplatin used as reference compounds were determined on the human MCF-7, HeLa, and HEp-2 cell lines, according to a previously published microtiter assayCitation27,Citation28, and the results are shown in . The Pt(II) complex 1, bearing chloro ligands as leaving groups, was found to be more active than 2, bearing iodo ligands as leaving groups, on all cell lines used. These findings are consistent with data reported in the literature that the cisplatin analogs, containing chloro ligands, exhibit much stronger therapeutic features than analogs in which the ligands are azides, cyanides, iodides, or rhodanatesCitation40. Moreover, other data in the literature report that dibromo and diiodo analogs of cisplatin hydrolyze at progressively slower rates compared to the dichloro complex, and this reactivity is reflected in the order of antitumor potency: I− < Br− < Cl−. It was reported that this could be attributed to an increase in kinetic reactivity toward water in going from the iodo to the chloro complex. This order implies that both electronic and steric factors influence the activityCitation41. It is also reported that the binding to DNA of these drugs with halogeno leaving groups is almost certainly preceded by aquation, with the loss of one or more of the leaving groupsCitation42, and also iodo is generally a poorer leaving group than chloro in the structure of Pt(II) complexesCitation43. In the test on the HEp-2 cell line, compound 1, which was found to be almost 12-fold more active than cisplatin, caused a significant reduction of cell growth.
Table 1. IC50 (μM) values of Pt(II) and Pt(IV) complexes on selected human tumor cell lines.
In the case of the Pt(IV) complexes, the compounds 3 bearing hydroxo and 4 bearing acetato groups as axial ligands were found to be two-fold more active than cisplatin and as active as cisplatin, respectively, against the HEp-2 cancer cell line. It is interesting to note that 3 did not show any significant cytotoxicity against the other two cancer cell lines used. Among the complexes synthesized, Pt(IV) complex 5 bearing chloro axial ligands had no activity against MCF-7 and HEp-2 cell lines at the concentration range tested.
In order to detect whether synthesized compounds 1–5 induce conformational changes in the DNA helix and whether there is a relationship between the plasmid-DNA binding affinity and the cytotoxicity of the compounds, the effect of the binding of compounds 1–5 to pBR322 plasmid DNA was determined by the ability of these compounds to alter the electrophoretic mobility and intensity of the covalently closed circular form I and open circular form II bands (). When pBR322 plasmid DNA was allowed to interact with increasing concentrations of cisplatin (), the mobility of both form I and form II bands increased essentially at the same rate, so that the bands remained parallel at all concentrations of cisplatin. The behavior of the gel electrophoretic mobility of both forms of pBR322 plasmid DNA–cisplatin adducts is consistent with previous reportsCitation44,Citation45.
Figure 1. Electrophoretograms relating to the interaction of pBR322 plasmid DNA with increasing concentration of cisplatin and 1–5. Lane 1 in both electrophoretograms contains untreated pBR322 plasmid DNA to serve as a control. For cisplatin, lane 2: 0.625 µM, lane 3: 1.25 µM, lane 4: 2.5 µM, lane 5: 5 µM, lane 6: 10 µM. For 1–5, lane 2: 0.625 µM, lane 3: 1.25 µM, lane 4: 2.5 µM, lane 5: 5 µM, lane 6: 10 µM, lane 7: 20 µM, lane 8: 40 µM, lane 9: 80 µM, lane 10: 160 µM. Roman numerals I and II indicate form I (covalently closed circular) and form II (open circular) plasmids, respectively.
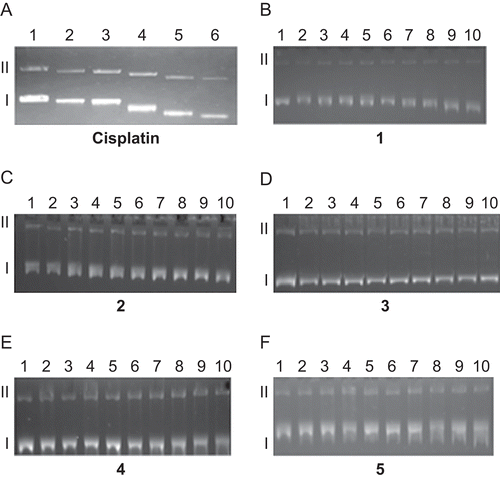
However, the increase in mobility of the form II band of the plasmid DNA was more pronounced in the case of 2 than 1; for 1 and 2 the increase in mobility of the form I and form II bands was smaller than that induced by cisplatin. The lower activity of 1 and 2 as compared to cisplatin may be interpreted by their reduced ability to bind with DNA because of a greater steric constraint introduced by bulkier benzimidazole carrier ligands. The change in mobility of pBR322 plasmid DNA bands as a result of the interaction with cisplatin is believed to be due to intrastrand bifunctional Pt(GG) and monofunctional Pt(G) adductsCitation5,Citation46. This may be interpreted in that the increase in mobility of form I and form II bands of pBR322 plasmid DNA with increasing concentration of 1 and 2 is indicative of the conformational change that occurs on the formation of bifunctional and monofunctional platinum adducts. For Pt(IV) complexes 3–5, no significant changes in mobility of the forms I and II bands of plasmid DNA were observed at the concentration range tested for 4 h incubation time.
In order to assess whether the benzimidazole–platinum complexes show affinity toward the guanine-guanine (GG) region, we carried out restriction endonuclease analysis of the compound–pBR322 plasmid DNA adducts digested by BamH1 enzyme. BamH1 is a restriction enzyme that recognizes G/GATCC and hydrolyzes the phosphodiester bond between adjacent guanine sites. pBR322 plasmid DNA has only one binding site for BamH1 that converts form I and form II DNA to linear form III DNACitation44,Citation47.
As the concentration of the compounds tested was increased it was seen that BamH1 digestion was increasingly prevented (). This is probably due to conformational change in the DNA brought about by covalent binding of the compounds with the GG region of the plasmid DNA. Arranging in the order of decreasing prevention of BamH1 digestion by the compounds gives: cisplatin > 1, 2 > 5 > 4 > 3. These results are consistent with the data reported in literature that the reduction rates and potentials of some diam(m)ine Pt(IV) complexes are dependent on the nature of the axial and equatorial ligands, but the axial ligands generally exert the stronger influence. It has also been reported that reduction occurs most readily when the axial ligands are chloro, least readily when they are hydroxo, and is intermediate when they are carboxylatoCitation13.
Figure 2. Electrophoretograms relating to the incubated mixtures of pBR322 plasmid DNA and increasing concentration of cisplatin and 1–5 followed by BamH1 digestion. For both cisplatin and 1–5, lane 1 is untreated and undigested with BamH1, lane 2 is untreated pBR322 plasmid DNA but digested with BamH1. For cisplatin, lane 3: 0.625 µM, lane 4: 1.25 µM, lane 5: 2.5 µM, lane 6: 5 µM, lane 7: 10 µM, lane 8: 20 µM. For 1–5, lane 3: 0.625 µM, lane 4: 1.25 µM, lane 5: 2.5 µM, lane 6: 5 µM, lane 7: 10 µM, lane 8: 20 µM, lane 9: 40 µM, lane 10: 80 µM, lane 11: 160 µM. Roman numerals I, II, and III indicate form I (covalently closed circular), form II (open circular), and form III (linear) plasmids, respectively.
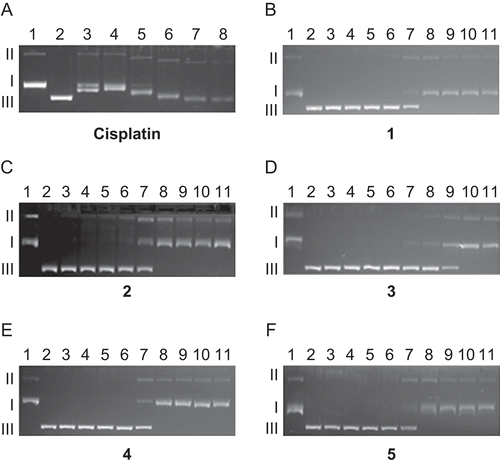
In the literature, it has been reported that steric constraint, hindering access to DNA for platinum complexes, would be greater in Pt(IV) complexes than in Pt(II) complexes, and Pt(IV)–DNA adducts are formed slowly as compared with Pt(II)–DNA adductsCitation10,Citation48. Furthermore, small changes in mobility of the form I band of pBR322 plasmid DNA treated with some Pt(II) and Pd(II) complexes containing sterically demanding carrier-ligands were attributed to monofunctional bindingCitation49. Considering the data reported in the literature and the preliminary results obtained in this study, it may be concluded that although Pt(IV) complexes 3–5 did not modify the electrophoretic mobility of plasmid DNA significantly, the formation of adequate adducts, presumably monofunctional adducts, may be sufficient to cause enough conformational change in pBR322 plasmid DNA treated with these compounds such that BamH1 digestion is prevented below their concentration, 10 µM. The conclusion drawn for Pt(IV) complexes 3–5 that their binding to plasmid DNA may be monofunctional for the incubation time of 4 h is consistent with the data obtained in the literature that monofunctional binding of octahedral Pt(IV) to DNA through the N7 guanine base is feasibleCitation11.
In summary, it was found that there is no clear correlation between the axial and equatorial ligand variation and plasmid DNA binding affinity and the in vitro antiproliferative activity of the platinum(II) and platinum(IV) complexes synthesized, which modified the tertiary structure of pBR322 plasmid DNA. It can also be concluded that the in vitro antiproliferative activity of compounds 1, 3, and 4 against the HEp-2 cell line is noteworthy, and must be taken into consideration in future studies.
Acknowledgements
We would like to thank Prof. Dr. İbrahim Burgu (Faculty of Veterinary Medicine, Ankara University) for his valuable support.
Declaration of interest
Financial support of part of this work (ECZ-F-EMB(SU) 2006-1) by the Research Foundation of Mersin University is gratefully acknowledged.
References
- Jung Y, Lipard SJ. Direct cellular responses to platinum-induced DNA damage. Chem Rev 2007;107:1387–407.
- Rabik CA, Dolan ME. Molecular mechanisms of resistance and toxicity associated with platinating agents. Cancer Treat Rev 2007;33:9–23.
- Jamieson ER, Lippard SJ. Structure, recognition, and processing of cisplatin-DNA adducts. Chem Rev 1999;99:2467–98.
- ChválováK, Brabec V, KaśpárkováJ. Mechanism of the formation of DNA-protein cross-links by antitumor cisplatin. Nucleic Acids Res 2007;35:1812–21.
- Fichtinger-Schepman AMJ, Van der Veer JL, den Hartog JHJ, Lohman PHM, Reedijk J. Adducts of the antitumor drug cis-diamminedichloroplatinum(II) with DNA: formation, identification, and quantitation. Biochemistry 1985;24:707–13.
- Fuertes MA, Alonso C, Perez JM. Biochemical modulation of cisplatin mechanisms of action: enhancement of antitumor activity and circumvention of drug resistance. Chem Rev 2003;103:645–62.
- Brabec V, Kasparkova J. Modfication of DNA by platinum complexes. Relation to resistance of tumors to platinum antitumor drugs. Drug Resist Update 2005;8:131–46.
- Reedijk J. Improved understanding in platinium antitumour chemistry. Chem Commun 1996;7:801–6.
- Wong E, Giandomenico CM. Current status of platinum-based antitumor drugs. Chem Rev 1999;99:2451–66.
- NovákováO, Vrána O, Kıseleva VI, Brabec V. DNA interactions of antitumor platinum(IV)complexes. Eur J Biochem 1995;228:616–24.
- Ellis LT, Er HM, Hambley TW. The influence of the axial ligands of a series of platinum(IV) anti-cancer complexes on their reduction to platinum(II) and reaction with DNA. Aust J Chem 1995;48:793–806.
- Hall MD, Hambley TW. Platinum(IV) antitumour compounds: their bioinorganic chemistry. Coord Chem Rev 2002;232:49–67.
- Choi S, Filotto C, Bisanzo M, Delaney S, Lagasee D, Whitworth JL, et al. Reduction and anticancer activity of platinum(IV) complexes. Inorg Chem 1998;37:2500–4.
- Hall MD, Mellor HR, Callaghan R, Hambley TW. Basis for design and development of platinum(IV) anticancer complexes. J Med Chem 2007;26:3403–11.
- Abu-Surrah AS, Kettunen M. Platinum group antitumor chemistry: design and development of new anticancer drugs complementary to cisplatin. Curr Med Chem 2006;13:1337–57.
- Kerr C. Satraplatin for hormone-refractory prostate cancer. Lancet Oncol 2007;8:290.
- GümüşF, İzgüF, Algül Ö. Synthesis and structural characterization of some 5(6)-substituted-2-hydroxymethylbenzimidazole derivatives and their platinum(II) complexes and determination of their in vitro antitumor activities by “Rec-Assay” test. FABAD J Pharm Sci 1996;21:7–15.
- GümüşF, Algül Ö. DNA binding studies with cis-dichlorobis(5(6)-non/chlorosubstituted-2-hydroxymethyl-benzimidazole)platinum(II) complexes. J Inorg Biochem 1997;68:71–4.
- Gümüş F, Pamuk İ, Özden, T, Yıldız S, Diril N, Öksüzoğlu E, et al. Synthesis, characterization and in vitro cytotoxic, mutagenic and antimicrobial activity of platinum(II) complexes with substituted benzimidazole ligands. J Inorg Biochem 2003;94:255–62.
- GümüşF, Algül ÖEren, G, Eroğlu H, Diril N, Gür S, et al. Synthesis, cytotoxic activity on MCF-7 cell line and mutagenic activity of platinum(II) complexes with 2-substituted benzimidazole ligands. Eur J Med Chem 2003;38:473–80.
- Gökçe M, Utku S, Gür S, Özkul A, GümüşF. Synthesis, in vitro cytotoxic and antiviral activity of cis-[Pt(R(-) and S(+)-2-α-hydroxybenzylbenzimidazole)2Cl2] complexes. Eur J Med Chem 2005;40:135–41.
- Utku S, GümüşF, Gür S, Özkul A. Synthesis and cytotoxic activity of platinum(II) and platinum(IV) complexes with 2-hydroxymethylbenzimidazole or 5(6)-choloro-2-hydroxymethylbenzimidazole ligands against MCF-7 and HeLa cell lines. Turk J Chem 2007;31:503–14.
- Utku S, GümüşF, Karaoğlu T, Özkul A. Cytotoxic activity of platinum(II) and platinum(IV) complexes bearing 5(6)-non/chlorosubstituted-2-hydroxymethylbenzimidazole ligands against Hep-2 cell line. Ankara Ecz Fak Derg 2007;36:21–30.
- GümüşF, Eren G, Açık L, Çelebi A, Öztürk F, Yılmaz Ş, et al. Synthesis, cytotoxic, and DNA interactions of new cisplatin analogues containing substituted benzimidazole ligands. J Med Chem 2009;52:1345–57.
- Preston PN. Synthesis, reactions, and spectroscopic properties of benzimidazoles. Chem Rev 1974;74:279–314.
- Rabıger DJ, Joullie MM. The ionization constant, ultraviolet and infrared spectra of some substituted benzimidazoles. J Org Chem 1964;29:476–82.
- Bernhard G, Reile H, Birnböck H, Spruss T, Schönenberger H. Standardized kinetic microassay to quantify differential chemosensitivity on the basis of proliferative activity. J Cancer Res Clin Oncol 1992;118:35–43.
- Reile H, Birnböck H, Bernhard G, Spruss T, Schönenberger H. Computerized determination of growth kinetic curves and doubling times from cells in microculture. Anal Biochem 1990;187:262–7.
- Stellwagen N. Nucleic Acid Electrophoresis. Berlin: Springer, 1998.
- Maurya RC, Mishra DD. Studies on some novel mixed-ligand cyanonitrosyl hetero complexes of chromium(I) with potentially bi- and tri-dentate benzimidazole derivatives. Syn React Inorg Met Org Chem 1990;20:1013–24.
- TunalıNK, Özkar S. Anorganik Tepkime Mekanizmaları. Anorganik Kimya. Ankara: Gazi Publishing, 1999:373–402.
- Mylonas S, Valavanidis A; Voukouvalidis V, Polyssiou M. Platinum(II)and palladium(II) complexes with amino acid derivatives. Synthesis, interpretation of IR and 1H NMR spectra and conformational implications. Inorg Chim Acta 1981;55:125–8.
- Dodoff N, Grancharov K, Gugova R, Spassovska N. Platinum(II) complexes of benzoic- and 3-methoxybenzoic acid hydrazides. Synthesis, characterization, and cytotoxic effect. J Inorg Biochem 1994;54:221–33.
- Giandomenico CM, Abrams MJ, Murrer BA, Vollano JF, Rheinheimer MI, Wyer SB, et al. Carboxylation of kinetically inert platinum(IV) hydroxy complexes. An entree into orally active platinum(IV) antitumor agents. Inorg Chem 1995;34:1015–21.
- Miller B, Altman J, Beck W. Platinum(IV) complexes of vicinal-1,2-diamines, and bis(vicinal-1,2-diamines) with an acylamimo function. Evidence for a platinum hydroperoxide intermediate upon oxidation. Inorg Chim Acta 1997;264:101–8.
- Khokhar AR, Deng Y, Al-Baker S, Yoshida M, Siddik ZH. Synthesis and antitumor activity of ammine/amine platinum(II) and (IV) complexes. J Inorg Biochem 1993;51:677–87.
- Lee EJ, Jun MJ, Sohn YS. Synthesis and properties of novel platinum(IV) complexes involving asymmetric chiral diamines as carrier ligands. Bull Korean Chem Soc 1999;20:1295–8.
- Hambley TW, Battle AR, Deacon GB. Lawrenz ET, Fallon GD, Gatehouse BM, et al. Modifying the properties of platinum(IV) complexes in order to increase biological effectiveness. Inorg Biochem 1999;77:3–12.
- Lippert B. Platinum nucleobase chemistry. Prog Inorg Chem 1989;37:1–97.
- Sawicka M, Kalinowska M, Skierska J, Lewandowski W. A review of selected anti-tumour therapeutic agents and reasons for multidrug resistance occurrence. J Pharm Pharmacol 2004;56:1067–81.
- Kushev D, Grünert R, Spassovska N, Golovinsky E, Bednarski PJ. Unusual reactivity of cytotoxic cis-dihydrazide Pt(II) complexes in aqueous solution. J Inorg Biochem 2003;96:469–77.
- Reedijk J. The relevance of hydrogen bonding in the mechanism of action of platinum antitumor compounds. Inorg Chim Acta 1992;198–200:873–81.
- Whittaker J, McFadyen WD, Wickham G, Wakelin LPG, Murray V. The interaction of DNA-targeted platinum phenanthridinium complexes with DNA. Nucleic Acids Res 1998;26:3933–9.
- Ushay HM, Tullius TD, Lippard SJ. Inhibition of the BamHI cleavage and unwinding of pBR322 deoxyribonucleic acid by the antitumor drug cis-dichlorodiammineplatinum(II). Biochemistry 1981;20:3744–8.
- Cheng H, Huq F, Beale P, Fisher K. Synthesis, characterization, activities, cell uptake and DNA binding of trinuclear complex: [{trans-PtCl(NH3)}2µ-{trans-Pd(NH3)(2-hydroxypyridine)-(H2N(CH2)6NH2)2]Cl4. Eur J Chem 2006;41:896–903.
- Keck MV, Lippard SJ. Unwinding of supercoiled DNA by platinum-ethidium and related complexes. J Am Chem Soc 1992;114:3386–90.
- Bowler BE, Hollis LS, Lippard SJ. Synthesis and DNA binding and phonicking properties of acridine orange linked by a polymethylene tether to (1,2-diaminoethane)dichloroplatinum(II). J Am Chem Soc 1984;106:6102–4.
- Perez JM, Camazon M, Alvarez-Valdes A, Quiroga AG, Kelland LR, Alonso C, et al. Synthesis, characterization and DNA modification induced by a novel Pt(IV)-bis(monoglutarate) complex which induced apoptosis in glioma cells. Chem-Biol Interact 1999;117:99–115.
- Neplechova K, Kasparkova J, Vrana O, Novakova O, Habtemariam A, Watchman B, et al. DNA interactions of new antitumor aminophosphine platinum(II) complexes. Mol Pharmacol 1999;56:20–30.