Abstract
Sixteen novel depsides were synthesized for the first time. Their chemical structures were clearly determined by 1H NMR, ESI mass spectra, and elemental analyses. All the compounds were assayed for antibacterial activities against three Gram-positive bacterial strains (Bacillus subtilis ATCC 6633, Staphylococcus aureus ATCC 6538, and Streptococcus faecalis ATCC 9790) and three Gram-negative bacterial strains (Escherichia coli ATCC 35218, Pseudomonas aeruginosa ATCC 13525, and Enterobacter cloacae ATCC 13047) by the MTT method. Compound 2-(2-methoxy-2-oxoethyl)phenyl 5-bromonicotinate (5) exhibited significant antibacterial activities against E. coli ATCC 35218 with an MIC of 0.78 μg/mL, which was superior to the positive control kanamycin B. In addition, compound 5 showed potent inhibitory activity against E. coli-induced interleukin-8 production.
Introduction
Depsides are compounds comprising two or more aromatic rings bound by a phenolic oxygen–ester linkage. It is reported that several well-characterized depsides exhibit antiproliferative, antibacterial, analgesic, antipyretic, anticancer, anti-human immunodeficiency virus (HIV)-1 integrase, and antiviral propertiesCitation1–9. In addition, as inhibitors of prostaglandin biosynthesis and leukotriene B4 biosynthesis, depsides are potent nonsteroidal antiinflammatoriesCitation10. Inflammation is a host response to a wide variety of tissue injuries characterized by the recruitment of leukocytes from the blood to the injured tissue. This movement is directed by chemokines, among which interleukin-8 (IL-8) plays an important roleCitation11. Interleukin-8 (IL-8) is produced by a number of cell types (e.g. T-lymphocytes, monocytes, endothelial cells, epithelial cells, and neutrophils) in response to a variety of stimuli, e.g. lipopolysaccharides (LPS), IL-1, and tumor necrosis factor (TNF)Citation12,Citation13. Raised levels of IL-8 have been detected in several disease statesCitation14, and experiments using monoclonal antibodies have indicated that inhibition of the action of this chemokine in some models of disease can lead to beneficial effectsCitation13,Citation15. Recently, a new depside, named jaboticabin (), was demonstrated to inhibit chemokine IL-8 production before and after cigarette smoke treatment of cellsCitation16.
In this study, we have synthesized 16 novel depsides and evaluated their antibacterial activity against six bacterial strains (Bacillus subtilis ATCC 6633, Staphylococcus aureus ATCC 6538, Streptococcus faecalis ATCC 9790, Escherichia coli ATCC 35218, Pseudomonas aeruginosa ATCC 13525, and Enterobacter cloacae ATCC 13047), and found that compound 5 exhibited significant antibacterial activities against E. coli ATCC 35218 with a minimum inhibitory concentration (MIC) of 0.78 μg/mL, which was superior to the positive control kanamycin B. In addition, in view of its significant antibacterial activity, compound 5 was selected to act against the IL-8 production induced by E. coli in gastric mucosal cells. Based on the data obtained, we found that compound 5 showed potent inhibitory activity against E. coli-induced interleukin-8 production.
Experimental
Chemistry
General
Melting points (uncorrected) were determined on an XT4 MP apparatus (Taike Corp., Beijing, China). Electrospray ionization (ESI) mass spectra were obtained on a Mariner System 5304 mass spectrometer, and 1H nuclear magnetic resonance (NMR) spectra were recorded on a Bruker PX500 or DPX300 spectrometer at 25°C with tetramethylsilane (TMS) and solvent signals allotted as internal standards. Chemical shifts are reported in ppm (δ). Elemental analyses were performed on a CHN-O-Rapid instrument and were within ± 0.4 % of the theoretical values.
Separation of the compounds by column chromatography was carried out with silica gel 60 (200–300 mesh ASTM; E. Merck). The quantity of silica gel used was 50–100 times the weight charged on the column. Then, the eluates were monitored using thin layer chromatography (TLC).
General experimental procedure for the synthesis of compounds 3–18
A solution of 2-hydroxyphenylacetic acid (7.6 g, 50 mmol) in methanol or isobutyl alcohol (50 mL) containing concentrated H2SO4 (5 mL) was refluxed overnight. Water (100 mL) was added, the organic phases were washed with saturated NaCl (100 mL) and dried over Na2SO4, and the solvents were evaporated. This step yielded compound 2. To a stirred solution of compound 2 (10 mmol) in dichloromethane (50 mL) was added differently substituted nicotinic acid or benzoic acid, N,N-dimethylaminopyridine (248 mg, 2.03 mmol), and N,N-dicyclohexylcarbodiimide (2.26 g, 11 mmol). Then, the mixture was refluxed overnight. Flash chromatography (acetate:petroleum ether 1:5 or 1:2) afforded the corresponding depsides (compounds 3–18) as powder or oil.
2-(2-Methoxy-2-oxoethyl)phenyl 2-bromonicotinate (3) White oil. Yield 80%. 1H NMR (300 MHz, DMSO-d6): 3.52 (s, 3H); 3.71 (s, 2H); 7.27–7.37 (m, 2H); 7.36–7.42 (m, 2H); 7.64–7.72 (m, 1H); 8.47–8.56 (m, 1H); 8.69–8.71 (m, 1H). MS (ESI): 349.9 (C15H13BrNO4, [M + H]+). Anal. Calcd for C15H12BrNO4: C, 51.45; H, 3.45%; Found: C, 51.27; H, 3.58%.
2-(2-Methoxy-2-oxoethyl)phenyl 2-chloronicotinate (4) White oil. Yield 83%. 1H NMR (300 MHz, DMSO-d6): 3.50 (s, 3H); 3.72 (s, 2H); 7.28–7.36 (m, 2H); 7.39–7.42 (m, 2H); 7.66–7.70 (m, 1H); 8.49–8.53 (m, 1H); 8.68–8.70 (m, 1H). MS (ESI): 306.0 (C15H13ClNO4, [M + H]+). Anal. Calcd for C15H12ClNO4: C, 58.93; H, 3.96%; Found: C, 58.78; H, 3.87%.
2-(2-Methoxy-2-oxoethyl)phenyl 5-bromonicotinate (5) White powder. Yield 82%, mp: 67–68°C, 1H NMR (300 MHz, DMSO-d6): 3.48 (s, 3H); 3.75 (s, 2H); 7.27–7.44 (m, 4H); 8.60–8.61 (t, J = 2.01 Hz, 1H); 9.06–9.07(d, J = 2.01 Hz, 1H); 9.19–9.20 (d, J = 2.01 Hz, 1H). MS (ESI): 349.1 (C15H13BrNO4, [M + H]+). Anal. Calcd for C15H12BrNO4: C, 51.45; H, 3.45%; Found: C, 51.32; H, 3.61%.
2-(2-Methoxy-2-oxoethyl)phenyl 5-chloronicotinate (6) White powder. Yield 77%, mp: 67–68°C, 1H NMR (300 MHz, DMSO-d6): 3.45 (s, 3H); 3.73 (s, 2H); 7.26–7.42 (m, 4H); 8.61–8.63 (t, J = 2.01 Hz, 1H); 9.07–9.08 (d, J = 2.01 Hz, 1H); 9.18–9.20 (d, J = 2.01 Hz, 1H). MS (ESI): 306.0 (C15H13ClNO4, [M + H]+). Anal. Calcd for C15H12ClNO4: C, 58.93; H, 3.96%; Found: C, 58.81; H, 3.76%.
2-(2-Methoxy-2-oxoethyl)phenyl 5-hydroxynicotinate (7) White powder. Yield 79%, mp: 106–108°C, 1H NMR (300 MHz, DMSO-d6): 3.48 (s, 3H); 3.69 (s, 2H); 7.27–7.34 (m, 2H); 7.39– 7.44 (m, 2H); 7.72–7.74 (m, 1H); 8.43–8.45 (m, 1H); 8.70–8.71 (m, 1H); 10.51 (s, 1H). MS (ESI): 288.0 (C15H14NO5, [M + H]+). Anal. Calcd for C15H13NO5: C, 62.72; H, 4.56%; Found: C, 62.61; H, 4.41%.
2-(2-Isobutoxy-2-oxoethyl)phenyl 5-bromonicotinate (8) White oil. Yield 83%. 1H NMR (300 MHz, DMSO-d6): 0.83 (s, 3H); 0.85 (s, 3H); 1.77–1.90 (m, 1H); 3.54 (s, 2H); 3.79–3.81 (d, J = 6.6 Hz, 2H); 6.71–6.82 (m, 2H); 7.04–7.11 (m, 2H); 7.64–7.72 (m, 1H); 8.47–8.56 (m, 1H); 8.69–8.71 (m, 1H). MS (ESI): 392.0 (C18H19BrNO4, [M + H]+). Anal. Calcd for C18H18BrNO4: C, 55.12; H, 4.63%; Found: C, 55.31; H, 4.49%.
2-(2-Isobutoxy-2-oxoethyl)phenyl 5-chloronicotinate (9) White oil. Yield 78%. 1H NMR (300 MHz, DMSO-d6): 0.82 (s, 3H); 0.84 (s, 3H); 1.75–1.88 (m, 1H); 3.52 (s, 2H); 3.77–3.80 (d, J = 6.6 Hz, 2H); 6.74–6.83 (m, 2H); 7.06–7.13 (m, 2H); 7.64–7.73 (m, 1H); 8.49–8.56 (m, 1H); 8.67–8.70 (m, 1H). MS (ESI): 348.0 (C18H19ClNO4, [M + H]+). Anal. Calcd for C18H18ClNO4: C, 62.15; H, 5.22%; Found: C, 62.28; H, 5.41%.
2-(2-Isobutoxy-2-oxoethyl)phenyl 2-bromonicotinate (10) White oil. Yield 76%. 1H NMR (300 MHz, DMSO-d6): 0.83 (s, 3H); 0.84 (s, 3H); 1.74–1.82 (m, 1H); 3.50 (s, 2H); 3.75–3.83 (d, J = 6.6 Hz, 2H); 6.71–6.81 (m, 2H); 7.08–7.14 (m, 2H); 7.62–7.71 (m, 1H); 8.47–8.53 (m, 1H); 8.66–8.72 (m, 1H). MS (ESI): 392.0 (C18H19BrNO4, [M + H]+). Anal. Calcd for C18H18BrNO4: C, 55.12; H, 4.63%; Found: C, 55.28; H, 4.41%.
2-(2-Isobutoxy-2-oxoethyl)phenyl 2-chloronicotinate (11) White oil. Yield 80%. 1H NMR (300 MHz, DMSO-d6): 0.84 (s, 3H); 0.86 (s, 3H); 1.72–1.80 (m, 1H); 3.53 (s, 2H); 3.73–3.89 (d, J = 6.6 Hz, 2H); 6.74–6.84 (m, 2H); 7.14–7.19 (m, 2H); 7.56–7.68 (m, 1H); 8.34–8.46 (m, 1H); 8.62–8.71 (m, 1H). MS (ESI): 348.0 (C18H19ClNO4, [M + H]+). Anal. Calcd for C18H18ClNO4: C, 62.15; H, 5.22%; Found: C, 62.31; H, 5.36%.
2-(2-Isobutoxy-2-oxoethyl)phenyl 5-hydroxynicotinate (12) White oil. Yield 77%. 1H NMR (300 MHz, DMSO-d6): 0.82 (s, 3H); 0.84 (s, 3H); 1.68–1.74 (m, 1H); 3.54 (s, 2H); 3.68–3.79 (d, J = 6.6 Hz, 2H); 6.62–6.78 (m, 2H); 7.11–7.18 (m, 2H); 7.53–7.69 (m, 1H); 8.52–8.63 (m, 1H); 8.67–8.78 (m, 1H); 10.55 (s, 1H). MS (ESI): 330.1 (C18H20NO5, [M + H]+). Anal. Calcd for C18H19NO5: C, 65.64; H, 5.81%; Found: C, 65.49; H, 5.67%.
2-(2-Isobutoxy-2-oxoethyl)phenyl 4-fluorobenzoate (13) White oil. Yield 76%. 1H NMR (300 MHz, DMSO-d6): 0.82 (s, 3H); 0.85 (s, 3H); 1.68–1.74 (m, 1H); 3.54 (s, 2H); 3.68–3.79 (d, J = 6.6 Hz, 2H); 6.62–6.78 (m, 2H); 7.11–7.18 (m, 2H); 7.41–7.47 (m, 2H); 8.15–8.23 (m, 2H). MS (ESI): 331.1 (C19H20FO4, [M + H]+). Anal. Calcd for C19H19FO4: C, 69.08; H, 5.80%; Found: C, 69.22; H, 5.62%.
2-(2-Isobutoxy-2-oxoethyl)phenyl 4-chlorobenzoate (14) White oil. Yield 80%. 1H NMR (300 MHz, DMSO-d6): 0.81 (s, 3H); 0.84 (s, 3H); 1.62–1.71 (m, 1H); 3.53 (s, 2H); 3.56–3.72 (d, J = 6.6 Hz, 2H); 6.61–6.77 (m, 2H); 7.15–7.24 (m, 2H); 7.45–7.49 (m, 2H); 8.12–8.22 (m, 2H). MS (ESI): 347.1 (C19H20ClO4, [M + H]+). Anal. Calcd for C19H19ClO4: C, 65.80; H, 5.52%; Found: C, 65.68; H, 5.65%.
2-(2-Isobutoxy-2-oxoethyl)phenyl 4-bromobenzoate (15) White oil. Yield 74%. 1H NMR (300 MHz, DMSO-d6): 0.82 (s, 3H); 0.85 (s, 3H); 1.61–1.74 (m, 1H); 3.52 (s, 2H); 3.59–3.71 (d, J = 6.6 Hz, 2H); 6.58–6.73 (m, 2H); 7.09–7.22 (m, 2H); 7.37–7.42 (m, 2H); 8.19–8.25 (m, 2H). MS (ESI): 391.0 (C19H20BrO4, [M + H]+). Anal. Calcd for C19H19BrO4: C, 58.33; H, 4.89%; Found: C, 58.46; H, 4.71%.
2-(2-Isobutoxy-2-oxoethyl)phenyl 4-nitrobenzoate (16) White oil. Yield 72%. 1H NMR (300 MHz, DMSO-d6): 0.81 (s, 3H); 0.84 (s, 3H); 1.57–1.68 (m, 1H); 3.54 (s, 2H); 3.62–3.78 (d, J = 6.6 Hz, 2H); 6.49–6.66 (m, 2H); 7.13–7.27 (m, 2H); 7.31–7.42 (m, 2H); 8.23–8.28 (m, 2H). MS (ESI): 358.1 (C19H20NO6, [M + H]+). Anal. Calcd for C19H19NO6: C, 63.86; H, 5.36%; Found: C, 63.71; H, 5.19%.
2-(2-Isobutoxy-2-oxoethyl)phenyl 4-methylbenzoate (17) White oil. Yield 77%. 1H NMR (300 MHz, DMSO-d6): 0.83 (s, 3H); 0.85 (s, 3H); 1.52–1.64 (m, 1H); 2.38 (s, 1H); 3.58 (s, 2H); 3.67–3.83 (d, J = 6.6 Hz, 2H); 6.37–6.56 (m, 2H); 7.17–7.24 (m, 2H); 7.37–7.43 (m, 2H); 8.27–8.31 (m, 2H). MS (ESI): 327.1 (C20H23O4, [M + H]+). Anal. Calcd for C20H22O4: C, 73.60; H, 6.79%; Found: C, 73.48; H, 6.91%.
2-(2-Isobutoxy-2-oxoethyl)phenyl 4-methoxybenzoate (18) White oil. Yield 81%. 1H NMR (300 MHz, DMSO-d6): 0.82 (s, 3H); 0.84 (s, 3H); 1.57–1.64 (m, 1H); 3.47 (s, 3H); 3.56 (s, 2H); 3.59–3.66 (d, J = 6.6 Hz, 2H); 6.61–6.75 (m, 2H); 7.13–7.24 (m, 2H); 7.35–7.41 (m, 2H); 8.24–8.27 (m, 2H). MS (ESI): 343.1 (C20H23O5, [M + H]+). Anal. Calcd for C20H22O5: C, 70.16; H, 6.48%; Found: C, 70.32; H, 6.61%.
Biological activity
Cell culture
The human gastric epithelial cancer cell line AGS (ATCC, WA, USA) was grown in Ham’s F12 containing 10% fetal bovine serum (FBS), l-glutamine, 100 U mL−1 penicillin G, and 100 mg mL−1 streptomycin. Cell cultures were maintained at 37°C in a humidified atmosphere of 95% air and 5% CO2.
E. coli culture
The strain used in this study was Gram-negative bacteria E. coli ATCC 35218, which was cultured on MH medium (casein hydrolysate 17.5 g, soluble starch 1.5 g, beef extract 1000 mL) for 1 day at 37°C before use.
Preparation of E. coli water extract
E. coli ATCC 35218 was harvested from agar plants and then suspended in distilled water at a concentration of 2. 5 × 108 CFU mL−1. After vortex-mixing for 1 min, the suspension was incubated at room temperature for 40 min and then centrifuged at 20,000 g for 20 min. Finally the supernatant was filtered through a 0.2 mm filter and stored at −20°C until use.
IL-8 assessment
Briefly, AGS cells, grown for 2 days on 24-well plates to ∼80% confluence in Ham’s F12 medium, were washed three times with serum-free Ham’s F12 and then preincubated with compound 5 and aspirin in serial concentrations of 15, 30, and 60 μmol L−1 with a concentration of dimethylsulfoxide (DMSO) lower than 1% for 1 h. After that, cells were incubated with 10% E. coli water extract (v/v) for 12 h. The supernatant was aspirated, centrifuged at 500 g for 10 min, and then stored at −80°C. The levels of IL-8 in the culture supernatant were determined by a commercially available enzyme-linked immunosorbent assay (ELISA) kit (Rapidbio, USA) according to the instructions of the manufacturer.
MTT assay for cellular viability
Cell viability was assessed using the MTT (3-(4,5-dimethylthiazol-2-yl)-2,5-diphenyltetrazoliumbromide) assay. Briefly, AGS cells, grown for 24 h on 24-well plates, were incubated with compound 5 and aspirin at serial concentrations of 15, 30, and 60 μmol L−1 for 48 h with the concentration of DMSO lower than 1%. Then MTT (5 mg L−1) was added to each well for a 4 h period. After the formation of formazan crystals, the culture medium supernatant was removed from the wells without disruption of the precipitate. The formazan crystals were then dissolved in 150 μL DMSO/well. The absorbance was measured at 570 nm using a microplate spectrophotometer (Dynex Technologies, Chantilly, VA).
Statistical analysis
One-way analysis of variance was performed to compare differences between groups. Two-tailed probability values were derived, and a p value of < 0.05 was considered statistically significant.
Results and discussion
Chemistry
Sixteen depsides were synthesized for the first time. The synthesis of compounds 3–18 followed the general pathway outlined in . They were prepared through two steps. First, a solution of 2-hydroxyphenylacetic acid (compound 1) in methanol or isobutyl alcohol containing concentrated H2SO4 was refluxed overnight. This step yielded the corresponding ester (compound 2). Second, the coupling reaction between the obtained esters and the differently substituted nicotinic acid or benzoic acid was performed by using N,N-dicyclohexylcarbodiimide (DCC) and 4-dimethylaminopyridine (DMAP) in anhydrous CH2Cl2. Then, compounds 3–18 were obtained by subsequent purification with flash chromatography. All of the synthetic compounds gave satisfactory analytical and spectroscopic data, which were in full accordance with their depicted structures.
Antibacterial activity
All the compounds prepared were evaluated for their antibacterial activities against three Gram-positive bacterial strains (B. subtilis ATCC 6633, S. aureus ATCC 6538, and S. faecalis ATCC 9790) and three Gram-negative bacterial strains (E. coli ATCC 35218, P. aeruginosa ATCC 13525, and E. cloacae ATCC 13047) by the MTT method, and the results are shown in .
Table 1. MICs (minimum inhibitory concentrations) (μg/mL) of the synthetic compounds.
As shown in , in general, compounds 3–12 exhibited better antibacterial activity than compounds 13–18 against E. coli ATCC 35218, which indicated that nicotinic acid derivatives had better antibacterial activity than that of benzoic acid derivatives. Among them, compounds 3, 5, and 6 displayed potent activity, with MIC values of 1.562 μg/mL, 0.78 μg/mL, and 1.562 μg/mL against E. coli ATCC 35218, which were superior to the positive control kanamycin B. Compound 4 exhibited significant activity, with an MIC value of 3.125 μg/mL against E. coli ATCC 35218, which was comparable to the positive control kanamycin B. In view of its significant antibacterial activity, compound 5 (MIC: 0.78 μg/mL) was selected to act against the IL-8 production induced by E. coli in gastric mucosal cells.
IL-8 assessment
Gram-negative bacterial infections rapidly induce an inflammatory response in which the cytokine network plays a major role. LPS released from these bacteria may be a major immunogen contributing to the cytokine burst by the LPS ± LPS binding protein (BP) ± CD14 complex formationCitation17,Citation18. IL-8 is a cytokine implicated in some cancers and a wide range of chronic inflammatory conditions, including rheumatoid arthritis, heart disease, and gastritis. In addition, IL-8 has been shown previously to be derived after stimulation with E. coli in transformed epithelial cell-linesCitation18,Citation19. In this study, Gram-negative bacteria E. coli were used to stimulate the human gastric epithelial cancer cell line AGS to produce IL-8, then compound 5 was assessed by enzyme-linked immunosorbent assay (ELISA).
Fortunately, we found that compound 5 exhibited a strong attenuation of IL-8 production induced by E. coli water extract in AGS cells, as shown in . Aspirin was used as a reference. AGS cells were preincubated with or without aspirin or compound 5 in serial concentrations of 15, 30, and 60 μmol L−1 for 1 h and then stimulated by 10% E. coli water extract (v/v) for 12 h. The levels of IL-8 in the culture supernatant were determined by ELISA. “Basal” represents cells without incubation of E. coli water extract, and “Control” shows cells incubated with E. coli water extract but without any agent. The data obtained indicated that E. coli alone stimulated AGS cells to produce as much as 1100 pg/mL IL-8. Compound 5 showed a strong attenuation of IL-8 production induced by E. coli in AGS cells.
Figure 2. Inhibitory activities of compound 5 on IL-8 production (pg/mL) induced by E. coli water extract. Results are mean ± SEM of 3–5 experiments. Comparison 15, 30, and 60 μmol L−1 of all agents versus control: ***p < 0.001; comparison compound 5 versus aspirin: ###p < 0.001. C, concentration of agent.
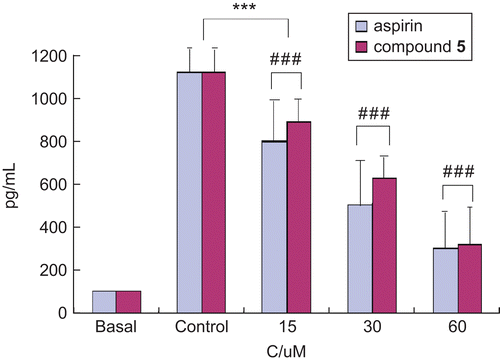
Different dose-dependent attenuations of E. coli-induced IL-8 production were seen with the addition of aspirin and compound 5. Compound 5 significantly decreased the IL-8 level in a dose-dependent way at concentrations of 15, 30, and 60 μmol L−1 compared with control, and the lowest IL-8 production was observed when the concentration of compound 5 was 60 μmol L−1. The same inhibition of E. coli-induced IL-8 production could be observed when aspirin was preincubated with AGS cells followed by the addition of E. coli water extract. However, the IL-8 production was decreased to 890 pg/mL and 610 pg/mL when the concentrations of aspirin were 15 μmol L−1 and 30 μmol L−1 respectively, which were lower than those of compound 5, indicating that aspirin showed a slightly stronger inhibition of E. coli-induced IL-8 production than compound 5 at the two concentrations. However, there was scarcely any difference at the concentration 60 μmol L−1.
In addition, cell viability was assessed using the MTT assay to see whether compound 5 and aspirin affected cell viability at the concentrations tested (15, 30, and 60 μmol L−1), as shown in . AGS cells were incubated with aspirin or compound 5 at serial concentrations of 15, 30, and 60 μmol L−1. The MTT assay was performed 48 h later. The results shown in demonstrate that compound 5 and aspirin did not affect cell viability at the concentrations tested (15, 30, and 60 μmol L−1). Based on the data obtained in this study, it can be concluded that compound 5 would be a potential and promising anti-inflammatory agent.
Conculsion
In this study, 16 novel depsides were synthesized for the first time. All the compounds were assayed for antibacterial activities against three Gram-positive bacterial strains and three Gram-negative bacterial strains by the MTT method. Compound 5 exhibited significant antibacterial activities against E. coli ATCC 35218, with an MIC of 0.78 μg/mL, and was selected to act against the IL-8 production induced by E. coli in gastric mucosal cells. Compound 5 significantly decreased the IL-8 level in a dose-dependent manner at concentrations of 15, 30, and 60 μmol L−1 compared with control, and the lowest IL-8 production was observed when the concentration of compound 5 was 60 μmol L−1.
Declaration of interest
The work was financed by grants (Project 30772627) from the National Natural Science Foundation of China.
References
- Vartia KO. Antibiotics in lichens. In: Ahmadjian V, Hale ME, eds. The Lichens, 3rd ed. New York: Academic Press, 1973:547–61.
- Hale ME. The Biology of Lichens. London: Edward Arnold, 1983.
- Ingolfsdottir K, Bloomfield SF, Hylands PJ. In vitro evaluation of the antimicrobial activity of lichen metabolites as potential preservatives. Antimicrob Agents Chemother 1985;28:289–92.
- Sankawa U, Shibuya M, Ebizuka Y, Noguchi H, Kinoshita T, Iitaka Y, et al. Depside as potent inhibitor of prostaglandin biosynthesis: a new active site model for fatty acid cyclooxygenase. Prostoglandins 1982;24:21–34.
- Proksa B, Adamcova J, Sturdikova M, Fuska J.Metabolites of Pseudevernia furfuracea (L.) Zopf. and their inhibition potential of prototypic enzymes. Pharmazie 1994;49:282–3.
- Yamamoto Y, Miura Y, Kinoshita Y, Higuchi M, Yamada Y, Murakami A, et al. Screening of tissue cultures and thalli of lichens and some of their active constituents for inhibition of tumor-promoter-induced Epstein-Barr virus activation. Chem Pharm Bull 1995;43:1388–90.
- Kumar KCS, Muller K.Lichen metabolites. 2. Antiproliferative and cytotoxic activity of gyrophoric, usnic, and diffractaic acid on human keratinocyte growth. J Nat Prod 1999;62:821–3.
- Nielsen J, Nielsen PH, Frisvad JC. Fungal depside, guisinol, from a marine derived strain of Emericella ungui. Phytochemistry 1998;50:263–5.
- Neamati N, Hong HX, Mazumder A, Wang SM, Sunder S, Nicklaus M, et al. Depsides and depsidones as inhibitors of HIV-1 integrase: discovery of novel inhibitors through 3D database searching. J Med Chem 1997;40:942–51.
- Kumar KCS, Muller K.Depsides as non-redox inhibitors of leukotriene B4 biosynthesis and HaCaT cell growth, 2. Novel analogues of obtusatic acid. Eur J Med Chem 2000;35:405–11.
- Lindenmeyer MT, Hrenn A, Kern C, Castro V, Murillo R, Muller S, et al. Sesquiterpene lactones as inhibitors of IL-8 expression in HeLa cells. Bioorg Med Chem 2006;14:2487–97.
- Attwood MR, Conway EA, Dunsdon RM, Greening JR, Handa BK, Jones PS, et al. Peptide based inhibitors of interleukin-8: structural simplification and enhanced potency. Bioorg Med Chem Lett 1997;7:429–32.
- Sekido N, Mukaida N, Harada A, Nakanishi I, Watanabe Y, Matsushima K. Prevention of lung reperfusion injury in rabbits by a monoclonal antibody against interleukin-8. Nature (London) 1993;365:654–7.
- Koch AE, Kunkel SE, Burrows JC, Evanoff HL, Haines GK, Pope RM, et al. Structure and neutrophil-activating properties of a novel inflammatory peptide (ENA-78) with homology to interleukin 8. J Immunol 1991;147:2187–95.
- Mulligan MS, Jones ML, Bolanski MA, Baganoff MP, Deppeler CL, Meyers DM, et al. Inhibition of lung inflammatory reactions in rats by an anti-human IL-8 antibody. J Immunol 1993;150:5585–95.
- Reynertson KA, Wallace AM, Adachi S, Gil RR, Yang H, Basile MJ, et al. Bioactive depsides and anthocyanins from jaboticaba (Myrciaria cauliflora). J Nat Prod 2006;69:1228–30.
- Dentener MA, Bazil V, Asmuth EJ, Ceska M, Buurman WA. Involvement of CD14 in lipopolysaccharide-induced tumor necrosis factor-alpha, IL-6 and IL-8 release by human monocytes and alveolar macrophages. J Immunol 1993;150:2885–91.
- Brauner A, Soederhaell M, Jacobson SH, Lundahl J, Andersson U, Andersson J. Escherichia coli-induced expression of IL-1 [alpha], IL-1 [beta], IL-6 and IL-8 in normal human renal tubular epithelial cells. Clin Exp Immunol 2001;124, 423–8.
- Lammers KM, Helwig U, Swennen E, Rizzello F, Venturi A, Caramelli E, et al. Effect of probiotic strains on interleukin 8 production by HT29/19A cells. Am J Gastroenterol 2002;97:1182–6.