Abstract
A novel series of complexes of the type [M(C36H22N6)X]X2, where M = Cr(III), Mn(III), Fe(III); X = Cl−, NO3−, CH3COO−; and (C36H22N6) corresponds to the tetradentate macrocyclic ligand, have been synthesized by condensation of 1,8-diaminonaphthalene and isatin in the presence of trivalent metal salts in methanolic medium. The complexes have been characterized by elemental analysis, conductance and magnetic measurements, and UV/Vis, IR, and mass spectroscopy. On the basis of these studies, a five coordinate square pyramidal geometry for all of these complexes is proposed. All synthesized macrocyclic complexes have been tested for in vitro antimicrobial activities against some pathogenic bacterial strains, viz. Staphylococcus aureus, Bacillus subtilis (Gram-positive), Escherichia coli, Pseudomonas aeruginosa (Gram-negative), and two fungal strains, viz. Aspergillus niger, Aspergillus flavus. The MICs shown by the complexes against these microbial strains have been compared with MICs shown by standard antibiotic ciprofloxacin and the antifungal drug amphotericin-B.
Abbreviations: | ||
B.M., | = | Bohr magneton; |
CFU, | = | colony forming unit; |
DMF, | = | N,N-dimethylformamide; |
DMSO, | = | dimethylsulfoxide; |
IR, | = | infrared; |
MIC, | = | minimum inhibitory concentration; |
MRI, | = | magnetic resonance imaging; |
MTCC, | = | Microbial Type Culture Collection; |
MHA, | = | Mueller Hinton agar; |
MHB, | = | Mueller Hinton broth; |
MBC, | = | minimum bactericidal concentration; |
SDA, | = | Sabouraud dextrose agar; |
DOTA, | = | tetra-azacyclododecanetetra-acetic acid; |
NBA, | = | nitrobenzyl alcohol; |
NCCLS, | = | National Committee for Clinical Laboratory Standards. |
Introduction
The design and study of well-arranged metal-containing macrocycles is an interesting field of chemistryCitation1. Some synthetic macrocyclic complexes (e.g. Cu-complex) have been investigated for accelerating the photodegradation of hazardous pollutantsCitation2. The in situ one-pot template condensation reaction lies at the heart of macrocyclic chemistryCitation3,Citation4. Therefore, template reactions have been widely used for the synthesis of macrocyclic complexes where generally the transition metal ions are used as the templating agentCitation5. There is continued interest in synthesizing macrocyclic complexes because of their potential application in fundamental and applied sciencesCitation6,Citation7. Synthetic macrocyclic complexes mimic some naturally occurring macrocycles because of their resemblance to many natural macrocycles, such as metalloproteins and metalloenzymesCitation8. Macrocyclic nickel complexes find use in DNA recognition and oxidationCitation9. Macrocyclic copper complexes find use in DNA binding and cleavageCitation10 and copper containing proteins have been identifiedCitation11. Macrocyclic metal complexes of lanthanides, e.g. Gd3+, are used as MRI contrast agentsCitation12. A macrocyclic metal chelating agent (DOTA) is useful for detecting tumor lesionsCitation13. The chemistry of macrocyclic complexes is also important, due to their use as dyes and pigmentsCitation14 as well as nuclear magnetic resosnance (NMR) shift reagentsCitation15. Some macrocyclic complexes have received special attention because of their mixed soft–hard donor character and versatile coordination behaviorCitation16 and also their pharmacological properties, i.e. toxicity against bacterial and fungal growthCitation17. Prompted by these facts, in the present article, the synthesis and characterization of chromium(III), manganese(III), and iron(III) macrocyclic complexes derived from 1,8-diaminonaphthalene and isatin are discussed. The complexes were characterized using various physicochemical techniques such as electronic, IR, and mass spectroscopy, elemental analysis, and magnetic susceptibility and conductivity measurements. All synthesized macrocyclic complexes were also tested for their in vitro antimicrobial activities against some bacterial strains, viz. Staphylococcus aureus (MTCC 96), Bacillus subtilis (MTCC 121) (Gram-positive), Escherichia coli (MTCC 1652), and Pseudomonas aeruginosa (MTCC 741) (Gram-negative) and two fungal strains, viz. Aspergillus niger (MTCC 282) and Aspergillus flavus (MTCC 871). The MICs shown by the complexes against these microbial strains have been compared with MICs shown by standard antibiotic ciprofloxacin and antifungal drug amphotericin-B.
Experimental
Materials
All the chemicals and solvents used in this study were of AnalaR grade. 1,8-Diaminonaphthalene and isatin were procured from Acros, and metal salts were purchased from S.D.-Fine, Merck, and Ranbaxy, and were used as received.
Isolation of complexes
All the complexes were synthesized by template method, i.e. by condensation of 1,8-diaminonaphthalene and isatin in the presence of the respective trivalent metal salts. To a hot stirred methanolic solution (∼50 mL) of 1,8-diaminonaphthalene (10 mmol) was added trivalent chromium, manganese, or iron salt (5 mmol) dissolved in the minimum quantity of MeOH (∼20 mL). The resulting solution was refluxed for 0.5 h. Afterward, isatin (10 mmol) was added to the refluxing mixture and refluxing was continued for 8–10 h. The mixture was concentrated to half of its volume, cooled at room temperature, and kept in a desiccator overnight. After that, dark colored precipitates formed which were filtered, washed with methanol, acetone, and diethylether, and dried in vacuo. Yield was obtained of ∼60–75%. The complexes were soluble in DMF and DMSO. They were found to be thermally stable up to ∼260–285°C, after which decomposition occurred.
The template condensation of 1,8-diaminonaphthalene and isatin in the presence of trivalent metal salts, in the molar ratio 2:2:1, is shown by the following scheme:
where M = Cr(III), Mn(III), Fe(III); X = Cl−, NO3−, CH3COO− for Cr(III), Fe(III); X = CH3COO− for Mn(III).
Analytical and physical measurements
The microanalysis of C, H, and N was carried out by elemental analyzer (PerkinElmer 2400) at SAIF, Punjab University, Chandigarh. Magnetic susceptibility measurements were carried out at IIC, IIT Roorkee, on a vibrating sample magnetometer (Model PAR 155). The metal contents in the complexes were determined by the literature methodCitation18. IR spectra were recorded on a Fourier transform (FT-IR) spectrophotometer (PerkinElmer) in the range 4000–200 cm−1 using Nujol mull. Electronic spectra (in DMSO) were recorded on a Cary 14 spectrophotometer at room temperature. Fast atom bombardment (FAB) mass spectra (at room temperature) were recorded on a time-of-flight mass spectrometry electrospray (TOF MS ES+) machine. The conductivity was measured on a digital conductivity meter (HPG system, G-3001). Melting points were determined by using capillaries in an electrical melting point apparatus.
Biological activity
Test microorganisms
Four bacteria, Staphylococcus aureus (MTCC 96), Bacillus subtilis (MTCC 121) (Gram-positive), Escherichia coli (MTCC 1652), and Pseudomonas aeruginosa (MTCC 741) (Gram-negative), and two fungi, Aspergillus niger (MTCC 282) and Aspergillus flavus (MTCC 871), were used in the present study.
In vitro antibacterial activity
The antibacterial activities of all the synthesized macrocyclic complexes were evaluated by the agar well diffusion methodCitation19. All the cultures were adjusted to 0.5 McFarland standard, which is visually comparable to a microbial suspension of approximately 1.5 × 10Citation8 CFU/mLCitation20. Twenty milliliters of MHA medium was poured into each Petri plate and the agar plates were swabbed with a 100 µL inoculum of each test bacterium and kept for 15 min for adsorption. Using a sterile cork borer of 8 mm diameter, wells were bored into the seeded agar plates and these were loaded with a 100 µL volume at concentration of 4.0 mg/mL of each complex reconstituted in DMSO. All the plates were incubated at 37°C for 24 h. The antibacterial activity of each complex was evaluated by measuring the zone of growth inhibition against the test organisms with a zone reader (Hi Antibiotic zone scale). DMSO was used as a negative control whereas ciprofloxacin was used as a positive control. The experiments were performed in triplicate and the results are given as mean ± standard deviation.
Determination of minimum inhibitory concentration (MIC) of chemically synthesized complexes
The minimum inhibitory concentration is the lowest concentration of an antimicrobial compound that will inhibit the visible growth of a microorganism after overnight incubation. The MICs of the various complexes against the bacterial strains were determined through a macrodilution tube method as recommended by the NCCLSCitation21. In this method, various test concentrations of the synthesized complexes were made, from 128 to 0.25 µg/mL, in sterile tubes, nos. 1 to 10. A volume of 100 µL of sterile MHB medium was poured into each sterile tube followed by the addition of 200 µL test compound in tube 1. Two-fold serial dilutions were carried out from tube 1 to tube 10, and excess broth (100 µL) was discarded from the last tube, no. 10. To each tube, 100 µL of standard inoculum (1.5 × 10Citation8 CFU/mL) was added. Ciprofloxacin was used as control. Turbidity was observed after incubating the inoculated tubes at 37°C for 24 h. The minimum bactericidal concentration is the lowest concentration of antimicrobial that will prevent the growth of an organism after subculture onto antibiotic-free medium. The MBC was determined by spreading 100 µL of the complex from the test concentration that fell below the MIC to the MIC itself. All the plates were incubated for 24 h at 37°C. The growth was observed on each plate.
In vitro antifungal activity
The antifungal activities of the all the synthesized macrocyclic complexes were evaluated by the poisoned food techniqueCitation22. Molds were grown on SDA medium at 25°C for 7 days and used as inocula. Fifteen milliliters of molten SDA medium (45°C) was poisoned by the addition of a 100 µL volume of each compound having a concentration of 4.0 mg/mL, reconstituted in DMSO, poured into a sterile Petri plate, and allowed to solidify at room temperature. The solidified poisoned agar plates were inoculated at the center with fungal plugs (8 mm diameter), obtained from the actively growing colony, and incubated at 25°C for 7 days. DMSO was used as the negative control whereas amphotericin-B was used as the positive control. The experiments were performed in triplicate. The diameter of the fungal colony was measured and expressed as percent mycelial inhibition determined by applying the formula given by Al-Burtamani et al.Citation22:
Inhibition of mycelial growth % = (dc – dt)/dc × 100
where dc is the average diameter of the fungal colony in negative control plates, and dt is the average diameter of the fungal colony in experimental plates.
Results and discussion
Chemistry
The analytical data show the suggested formula for macrocyclic complexes as [M(C36H22N6)X]X2, where M = Cr(III), Mn(III), Fe(III); X = Cl−, NO3−, CH3COO−; and (C36H22N6) corresponds to the tetradentate macrocyclic ligand. Measurements of molar conductance in DMSO showed that these chelates are 1:2 electrolytesCitation23 (conductance 160–180 ohm−1 cmCitation2 mol−1). Various attempts such as crystallization using mixtures of solvents and low temperature crystallization were unsuccessful in obtaining a single crystal suitable for X-ray crystallography. However, the analytical, spectroscopic, and magnetic data enabled us to predict the possible structure of the synthesized complexes. All complexes gave satisfactory elemental analysis results as shown in .
Table 1. Analytical data of trivalent chromium, manganese, and iron complexes derived from 1,8-diaminonaphthalene and isatin.
IR spectra
It was noted that a pair of medium intensity bands were present in the spectrum of 1,8-diaminonaphthalene at 3350 and 3390 cm−1, corresponding to the ν(NH2) group, which were absent in the IR spectra of all the complexes. Further, no strong absorption band was observed near 1734 cm−1, indicating the absence of the >C=O group of the isatin moiety. The disappearance of these bands and appearance of a new strong absorption band near 1590–1629 cm−1 confirmed condensation of the carbonyl group of isatin and amino group of diaminonaphthalene and formation of the macrocyclic Schiff baseCitation24, as these bands may be assigned due to ν(C=N) stretching vibrationsCitation25. The value of the ν(C=N) stretching vibration was found to be lower (1590–1629 cm−1) than the expected value (1670–1695 cm−1). This lower value of ν(C=N) stretching may be explained on the basis of the drift of lone-pair density of the azomethine nitrogen toward the metal atomCitation26, indicating that coordination takes place through the nitrogen of the (C=N) group. The various absorption bands in the region of 1450–1600 cm−1 may be assigned due to ν(C=C) aromatic stretching vibrations of the naphthalene and isatin ring moietiesCitation26. The bands in the region 740–785 cm−1 may be assigned to ν(C-H) out of plane bending of aromatic ringsCitation27,Citation28. The presence of absorption bands at 1410–1445, 1295–1320, and 1015–1030 cm−1 in the IR spectra of all the nitrato complexes suggests that the nitrate groups are coordinated to the central metal ion in a unidentate fashionCitation29. The IR spectra of all the acetate complexes showed an absorption band in the region 1650–1680 cm−1, assigned to ν(COO−)as asymmetric stretching vibrations of the acetate ion, and another in the region 1258–1290 cm−1 that can be assigned to ν(COO−)s symmetric stretching vibration of the acetate ion. The difference νas − νs, which is around 390–370 cm−1, greater than 144 cm−1, indicates the unidentate coordination of the acetate group with the central metal ionCitation30.
The far infrared spectra showed bands in the region 420-450 cm−1, corresponding to ν(M-N) vibrationsCitation31–33. The presence of bands in all complexes in the region 420–450 cm−1 originates from (M-N) azomethine vibrational modes and identifies coordination of the azomethine nitrogenCitation34. The bands present in the range 300–320 cm−1 may be assigned to ν(M-Cl) vibrationCitation31–33. The bands present in the region 220–250 cm−1 in all nitrato complexes are related to the ν(M-O) stretching vibrationCitation31,Citation32.
Mass spectra
The FAB mass spectra of the Cr(III), Mn(III), Fe(III) macrocyclic complexes were recorded using an m-nitrobenzyl alcohol (NBA) matrix. All the spectra exhibited parent peaks due to molecular ions [M]+ and [M + 2]+. The proposed molecular formula of these complexes was confirmed by comparing their molecular formula weights with m/z values. The molecular ion [M]+ and [M + 2]+ peaks obtained for the various complexes are shown in . The data are in good agreement with the proposed molecular formula for these complexes, i.e. [M(C36H22N6)X]X2. This confirms formation of the macrocyclic frame. In addition to the molecular ion peaks, the spectra exhibited other peaks assignable to various fragments arising from thermal cleavage of the complexes (). The peak intensity gives an idea of the stability of the fragments.
Table 2. FAB mass spectral data of the trivalent chromium, manganese, and iron complexes derived from 1,8-diaminonaphthalene and isatin.
Magnetic measurements and electronic spectra
Chromium complexes
The magnetic moment of chromium(III) complexes was found in the range of 3.90–4.30 B.M., at room temperature, which is close to the predicted values for three unpaired electrons in the metal ionCitation26. The electronic spectra of the chromium complexes showed bands at ∼9010–9320 cm−1, 13,030–13,350 cm−1, 17,460–18,320 cm−1, 27,420–27,850 cm−1, and 34,810 cm−1. However, these spectral bands cannot be interpreted in terms of a four or six coordinated environment around the central metal atom. In turn, the spectra are consistent with that of five coordinated chromium(III) complexes, whose structure has been confirmed with the help of X-ray measurementsCitation35. On the basis of the analytical data, spectral studies, and electrolytic nature of these complexes, a five coordinated square pyramidal geometry may be assigned for these complexes. Thus, assuming the symmetry C4V for the complexesCitation36–38, the various spectral bands may be assigned as: 4B1→4Ea, 4B1→4B2, 4B1→4A2, and 4B1→4Eb.
Manganese complex
The magnetic moment of the manganese(III) complex was found to be 4.89 B.M., which indicates the high spin (d4) system26. The electronic spectra of the manganese complex showed three d-d bands, which lay in the regions 12,350–12,590, 16,050–18,820, and 35,420–35,700 cm−1. The higher energy band at 35,440–35,750 cm−1 may be assigned to charge transfer transitions. The spectra resemble those reported for five coordinate square pyramidal manganese complexesCitation36–38. This idea is further supported by the presence of the broad ligand field band at 20,400 cm−1, diagnostic of C4V symmetry, and thus the various bands may be assigned as follows: 5B1→5A1, 5B1→5B2, and 5B1→5E, respectively. The band assignment in single electron transition may be made as: dz2→dx2-y2, dxy→dx2-y2, and dxz, dyz→dx2-y2, respectively, in order of increasing energy.
Iron complexes
The magnetic moment of iron complexes lay in the range of 5.81–5.90 B.M., corresponding to the five unpaired electrons, which is close to the predicted high spin values for these metal ionsCitation26. The electronic spectra of the iron complexes showed various bands at 9820–9970, 15,520–15,575, and 27,550-27,730 cm−1, and these bands do not suggest octahedral or tetrahedral geometry around the metal atom. The spectral bands are consistent with the range of spectral bands reported for five coordinate square pyramidal iron(III) complexesCitation37–39. Assuming C4V symmetry for these complexes, the various bands can be assigned as: dxy→dxz, dyz and dxy→dz2. Any attempt to make accurate assignment is difficult due to interactions of the metal–ligand π-bond systems lifting the degeneracy of the dxz and dyz pair.
Biological activity
All the synthesized macrocyclic complexes were screened for their antibacterial and antifungal activities. All the tested complexes possessed variable antibacterial and antifungal activities against both Gram-positive (S. aureus, B. subtilis) and Gram-negative (E. coli, P. aeruginosa) bacteria and against the fungal strains (A. niger, A. flavus). To compare the antibacterial and antifungal activities shown by the synthesized complexes, a standard antibiotic, namely ciprofloxacin, and standard antifungal drug, amphotericin-B, were used ( and , and ). On the basis of the MICs shown by these complexes against the bacteria, compounds (4) and (7) were found to be most effective against both Gram-positive bacterial strains S. aureus and B. subtilis, showing an MIC of 8 µg/mL. In the whole series, the MIC of complexes (1) and (5) was found to be 32 µg/mL for S. aureus, whereas the MIC of complexes (1), (2), and (6) was 32 µg/mL for B. subtilis (, ). Complexes (4) and (7) showed an MIC of 32 µg/mL against P. aeruginosa and MIC of 64 µg/mL against E. coli. Similarly, the MBC of complexes (4) and (7) was found to be 16 µg/mL for S. aureus and B. subtilis and 64 µg/mL for P. aeruginosa, whereas the MBC of complexes (1), (2), and (6) was 64 µg/mL for B. subtilis. Similarly, complexes (1) and (5) showed an MBC of 64 µg/mL against S. aureus ().
Table 3. In vitro antibacterial activity of synthesized macrocyclic complexes through agar well diffusion method.
Table 4. Minimum inhibitory concentration (MIC) (in μg/mL) of synthesized macrocyclic complexes by using macrodilution method.
Table 5. Minimum bactericidal concentration (MBC) (in µg/mL) of synthesized macrocyclic complexes by using macrodilution method.
Figure 1. Comparison of MIC (μg/mL) of the synthesized macrocyclic complexes with standard antibiotic. a, Staphylococcus aureus (MTCC 96); b, Bacillus subtilis (MTCC 121); c, Escherichia coli (MTCC 1652); d, Pseudomonas aeruginosa (MTCC 741). Ciprofloxacin, standard antibiotic.
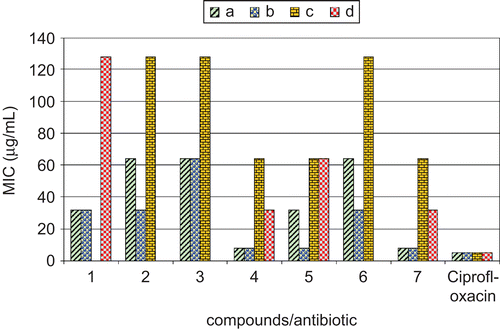
The antifungal activities of all the complexes were determined against two fungal strains, i.e. Aspergillus niger and Aspergillus flavus, and then compared with the standard antifungal drug amphotericin-B (, ). The antifungal activities (percentage inhibition) are given in . In the whole series, complexes (3) and (5) showed the highest percentage inhibition, around 65%, against fungal strain A. niger, whereas (2) and (5) showed the highest percentage inhibition, around 61%, against A. flavus. None of the tested complexes restricted fungal growth excellently (). However, of all the tested compounds, (1) and (4) showed about 55% inhibition of mycelial growth against A. niger, whereas compounds (1), (3), and (6) showed 50–55% inhibition of mycelial growth against A. flavus (, ).
Table 6. In vitro antifungal activities of synthesized complexes through poisoned food method.
Conclusions
Based on various studies such as elemental analysis, conductance measurements, magnetic susceptibilities, and IR, electronic, and MS spectral studies, a five coordinate square pyramidal geometry for all of these complexes is proposed. The proposed structure is shown in .
Figure 3. Proposed structure of the synthesized macrocyclic complexes, where M = Cr(III), Mn(III), Fe(III); X = Cl−, NO3−, CH3COO− for Cr(III), Fe(III); X = CH3COO− for Mn(III).
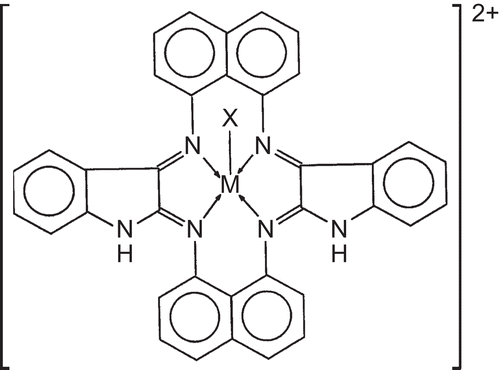
It has been suggested that chelation/coordination reduces the polarity of the metal ion mainly because of partial sharing of its positive charge with the donor group within the whole chelate ring systemCitation40. This process of chelation thus increases the lipophilic nature of the central metal atom, which, in turn, favors its permeation through the lipid layer of the membrane, thus causing the metal complex to cross the bacterial membrane more effectively, and hence increasing the activity of the complex. Besides this, many other factors such as solubility, dipole moment, and conductivity influenced by the metal ion may be possible reasons for the remarkable antibacterial activities of these complexesCitation41.
Acknowledgments
The authors would like to thank the authorities of NIT, Kurukshetra, for providing necessary research facilities.
Declaration of interest
One of the authors (D.P.S.) thanks the University Grants Commission, New Delhi, for financial support in the form of a Major Research Project (MRP-F. No.32-196/2006 (SR)) and another of the authors (K.K.) for the award of Project Fellowship under the above project.
References
- Chandra S, Gupta LK, Agrawal S. Modern spectroscopic and biological approach in the characterization of a novel 14-membered [N4] macrocyclic ligand and its transition metal complexes. Transit Met Chem 2007;32:240–5.
- Hanafy AI, Maki ABKT, Mostafa MM. Synthesis, structural and catalytic activity of binaphthyl macrocyclic complexes. Transit Met Chem 2007;32:960–6.
- Curtis NF. Macrocyclic coordination compounds formed by condensation of metal-amine complexes with aliphatic carbonyl compounds. Coord Chem Rev 1968;3:3–47.
- Niasari MS, Bazarganipour M, Ganjali MR, Norouzi P. Bis(macrocyclic)dinickel(II) complexes containing phenylene bridges between 13-membered triaza dioxa macrocyclic ligands: in-situ one pot template synthesis, characterization and catalytic oxidation of cyclohexene. Transit Met Chem 2007;32:9–15.
- Niasari MS, Davar F. In situ one-pot template synthesis (IOPTS) and characterization of copper(II) complexes of 14-membered hexaaza macrocyclic ligand 3,10-dialkyl-dibenzo-1,3,5,8,10,12-hexaazcyclo tetradecane. Inorg Chem Commun 2006;9:175–9.
- Kumar DS, Alexander V. Synthesis of lanthanide(III) complexes of chloro and bromo substituted 18-membered tetraaza macrocycles. Polyhedron 1999;18:1561–8.
- Ilhan S, Temel H, Ziyadanogullari R, Sekerci M. Synthesis and spectral characterization of macrocyclic Schiff base by reaction of 2,6-diaminipyridine and 1,4-bis(2-carboxyaldehydephenoxy) butane and its Cu(II), Ni(II), Pb(II), Co(III), La(III) complexes. Transit Met Chem 2007;32:584–90.
- Shakir M, Khatoon S, Parveen S, Azim Y. Synthesis and spectral studies of a 12-membered tetraimine macrocyclic ligand and its complexes. Transit Met Chem 2007;32:42–6.
- Muller JG, Chen X, Dadiz AC, Rokita SE, Burrows CJ. Macrocyclic nickel complexes in DNA recognition and oxidation. Pure Appl Chem 1993;65:545–50.
- Liu J, Lu TB, Deng H, Ji LN, Qu LH, Zhou H. Synthesis, DNA-binding and cleavage studies of macrocyclic Cu(II) complexes. Transit Met Chem 2003;28:116–21.
- Akilan P, Thirumavalavan M, Kandaswamy M. Synthesis of mono and binuclear copper(II) complexes using new macrocyclic tricompartmental unsymmetrical ligands: magnetic, electrochemical and catalytic studies. Polyhedron 2003;22:3483–92.
- Singh DP, Kumar R, Malik V, Tyagi P. Template synthesis, spectroscopic studies and biological activities of macrocyclic complexes derived from thiocarbohydrazide and glyoxal. J Enzyme Inhib Med Chem 2007;22:177–82.
- Kosmos C, Snook D, Gooden CS, Courtenay Luck NS, McCalla MJ, Meares CF, et al. Development of humoral immune responses against a macrocyclic chelating agent (DOTA) in cancer patients receiving radioimmunoconjugates for imaging and therapy. Cancer Res 1992;52:904–11.
- Seto J, Tamura S, Asai N, Kishii N, Kijima Y, Matsuzawa N. Macrocyclic functional dyes: applications to optical disk media, photochemical hole burning and non-linear optics. Pure Appl Chem 1996;68:1429–34.
- Dong W, Yang R, Yan L. Rare earth complexes of a new Schiff base macrocyclic ligand derived from 2,2′-(ethylenedioxy) bis-benzaldehyde and 2,2′-(ethylenedioxy) bis-benzylamine. Indian J Chem 2001;40A:202–6.
- Chandra S, Gupta R, Gupta N, Bawa SS. Biologically relevant macrocyclic complexes of copper spectral, magnetic, thermal and antibacterial approach. Transit Met Chem 2006;31:147–51.
- Chandra S, Gupta LK, Agrawal S. Synthesis spectroscopic and biological approach in the characterization of novel [N4] macrocyclic ligand and its transition metal complexes. Transit Met Chem 2007;32:558–63.
- Vogel AI. A Text Book of Quantitative Chemical Analysis, 5th ed. London: Longman, 1989.
- Ahmad I, Beg AJ. Antimicrobial and phytochemical studies on 45 Indian medicinal plants against multidrug resistant human pathogens. J Ethnopharmacol 2001;74:113–23.
- Andrews JM. Determination of minimum inhibitory concentrations. J Antimicrob Chemother 2001;48:5–16.
- NCCLS. Method for Dilution Antimicrobial Susceptibility Test for Bacteria that Grow Aerobically, Approved Standards, 5th ed. Villanova, PA: National Committee for Clinical Laboratory Standards, 2000.
- Al-Burtamani SKS, Fatope MO, Marwah RG, Onifade AK, Al-Said SH. Chemical composition, antibacterial and antifungal activities of the essential oil of Haplophyllum tuberculatum from Oman. J Ethnopharmocol 2005;96:107–12.
- Geary WJ. The use of conductivity measurements in organic solvents for the characterization of coordination compounds. Coord Chem Rev 1971;7:81–122.
- Singh DP, Kumar R, Tyagi P. Template synthesis, spectroscopic studies and biological screening of macrocyclic complexes derived from thiocarbohydrazide and benzil. Transit Met Chem 2006;31:970–3.
- Singh DP, Kumar K, Dhiman SS, Sharma J. Biologically active macrocyclic complexes derived from diaminonaphthalene and glyoxal: template synthesis and spectroscopic approach. J Enzyme Inhib Med Chem 2009;24:795–803.
- Singh DP, Kumar K, Sharma C. Antimicrobial active macrocyclic complexes of Cr(III), Mn(III), and Fe(III) with their spectoscopic approach. Eur J Med Chem 2009;44:3299–304.
- Chandra S, Gupta LK. Designing and synthesis of macrocyclic Schiff base ligand: study of interaction with Mn(II), Co(II), Ni(II) and Cu(II) and biological screening. J Indian Chem Soc 2005;82:454–8.
- Singh DP, Shishodia N, Yadav BP, Rana VB. Trivalent transition metal ion directed macrocycles. J Indian Chem Soc 2004;81:287–90.
- Chandra S, Gupta LK. Spectroscopic characterization of tetradentate macrocyclic ligand: its transition metal complexes. Spectrochim Acta A 2004;60:2767–74.
- Nakamoto K. Infrared and Raman Spectra of Inorganic and Coordination Compounds, 5th ed. New York:Wiley InterScience, 1997.
- Shakir M, Nasman OSM, Varkey SP. Binuclear [N6] 22-membered macrocyclic transition metal complexes: synthesis and characterization. Polyhedron 1996;15:309–14.
- Shakir M, Islam KS, Mohamed AK, Shagufa M, Hasan SS. Macrocyclic complexes of transition metals with divalent polyaza units. Transit Met Chem 1999;24:577–80.
- Chandra S, Kumar R. Synthesis and spectral studies on mononuclear complexes of chromium(III) and manganese(II) with 12-membered tetradentate N2O2, N2S2 and N4 donor macrocyclic ligand. Transit Met Chem 2004;29:269–75.
- Singh DP, Kumar R, Malik V, Tyagi P. Synthesis and characterization of complexes of Co(II), Ni(II), Cu(II), Zn(II), Cd(II) with macrocycle 3,4,11,12-tetraoxo-1,2,5,6,9,10,13,14-octaazacyclohexadeca-6,8,14,16-tetraene and their biological screening. Transit Met Chem 2007;32:1051–5.
- Wood JS. Stereochemical electronic structural aspects of five coordination compounds. Prog Inorg Chem 1972;16:227.
- Singh DP, Kumar R, Singh J. Synthesis and spectroscopic studies of biologically active compounds derived from oxalyldihydrazide and benzil, and their Cr(III), Fe(III) and Mn(III) complexes. Eur J Med Chem 2009;44:1731–6.
- Singh DP, Kumar R. Trivalent metal ion directed synthesis and characterization of macrocyclic complexes. J Serb Chem Soc 2007;72:1069–74.
- Singh DP, Kumar R, Singh J. Antibacterial activity and spectral studies of trivalent chromium, manganese, iron macrocyclic complexes derived from oxalyldihydrazide and glyoxal. J Enzyme Inhib Med Chem 2009;24:883–9.
- Lever ABP. Inorganic Electronic Spectroscopy, 2nd ed. Amsterdam: Elsevier, 1984.
- Chohan ZH, Pervez H, Rauf A, Khan KM, Supuran CT. Isatin-derived antibacterial and antifungal compounds and their transition metal complexes. J Enzyme Inhib Med Chem 2004;19:417–23.
- Chohan ZH, Scozzafava A, Supuran CT. Unsymmetrical 1,1-disubstituted ferrocenes: synthesis of Co(II), Cu(II), Ni(II) and Zn(II) chelates of ferrocenyl-1-thiadiazolo-1′-tetrazole, -1-thidiazolo-1′-triazole and -1-tetrazolo-1′-triazole with antimicrobial properties. J Enzyme Inhib Med Chem 2002;17:261–6.