Abstract
Inhibitions of 30 nM rabbit muscle 1-phosphofructokinase (PFK-1) by lithium, potassium, and sodium salts showed inhibition or not depending upon the anion present. Generally, potassium salts were more potent inhibitors than sodium salts; the extent of inhibition by lithium salts also varied with the anion. Li2CO3 was a relatively potent inhibitor of PFK-1 but LiCl and lithium acetate were not. Our results suggest that extents of inhibition by monovalent salts were due to both cations and anions, and the latter needs to be considered before inhibition can be credited to the cation. An explanation for monovalent salt inhibitions is proffered involving interactions of both cations and anions at negative and positive sites of PFK-1 that affect enzyme activity. Our studies suggest that lithium cations per se are not inhibitors: the inhibitors are the lithium salts, and we suggest that in vitro studies involving the effects of monovalent salts on enzymes should involve more than one anion.
Keywords::
Abbreviations: | ||
F 6-P, | = | fructose 6-phosphate; |
F 1,6-BP, | = | fructose 1,6-bisphosphate; |
RPFK-1, | = | BPFK-1, 1-phosphofructokinase from rabbit muscle and beef muscle, respectively; |
RMAK, | = | rabbit muscle adenylate kinase. |
Introduction
Comparisons of inhibitions of rabbit muscle 1-phosphofructokinase (RPFK-1) by ammonium salts, by ascorbate, and by monovalent salts of ammonium, potassium, and sodium were reported previouslyCitation1. We initiated studies on the effect of lithium salts on RPFK-1 activity because these salts are used therapeutically for manic–depressive disorderCitation2–4 with no clear evidence why they are effective. We reasoned that if lithium salts inhibited RPFK-1, and therefore glycolysis, the major energy source for the brain, perhaps some inhibition characteristic would serve as a clue to the therapeutic mechanisms. Our previous reportsCitation1 on the effects of monovalent salts on RPFK-1 activity tacitly implied that monovalent cations caused the inhibitions. Preliminary studies not given here suggested that while lithium, potassium, and sodium salts did inhibit RPFK-1, the inhibitions could not be dissociated from the contributing effects of anions. Emphasis in this study was placed on the effect of lithium chloride and lithium carbonate on RPFK-1 activity; the low solubility of lithium phosphate precluded it from this study. The effects of chloride, phosphate, and carbonate of potassium and sodium salts were also studied for comparisons with RPFK-1 inhibitions by lithium salts and by ascorbateCitation5–9.
Materials
Biologicals and chemicals
Sigma-Aldrich Co. was the source of chemicals and enzymes used in experiments and assay systems unless stated otherwise. Rabbit muscle G-actin (A 2522) had no aldolase (EC 4.1.2.13), lactic dehydrogenase (LDH, EC 1.1.1.28), or adenylate kinase (AK, EC 2.7.4.3) activity. Rabbit muscle aldolase (A 8811) was free of AK and LDH activity under our conditions.
Phosphofructokinase-1 preparation
Rabbit muscle (RPFK-1) and beef (BPFK-1) 1-phosphofructokinase (PFK-1, EC 2.7.1.56) was prepared by our laboratory from frozen tissue according to Kemp and WoodCitation10.
Criteria of purity
Purities of RPFK-1 and BPFK-1 were determined by sodium dodecyl sulfate-polyacrylamide gel electrophoresis (SDS PAGE) (not shown) that was run with 12% cross-linked gels and silver stained for proteins according to MorrisseyCitation11. All PFK-1 preparations used in these studies were devoid of AK, LDH, and aldolase activities and showed a single band in SDS PAGE.
Methods
All operations were at 25°C unless otherwise stated. Buffer used in these studies was 0.01 M Tris-phosphate, pH 8, for PFK-1 solutions unless stated otherwise.
Standard RMAK assay
We measured rabbit muscle adenylate kinase (RMAK) activity, AMP + ATP-Mg = ADP + ADP-Mg, according to AdamCitation12. A 1.0 mL assay mixture contained the final concentrations 0.3 mM phosphoenolpyruvate; 0.4 mM NADH (reduced nicotinamide adenine dinucleotide); 8.0 mM ATP (adenosine triphosphate) and 8 mM AMP (adenosine monophosphate); 8.1 mM MgCl2; and 20 mM potassium phosphate buffer, pH 8, unless stated otherwise.
30 nM RMAK assay
When AK solutions at low concentrations had rates below 0.05 absorbancy units/min in 100 µL of sample, the standard RMAK assay components above were concentrated to 10 times the final assay concentrations into 0.1 mLCitation9. This allowed buffered AK samples up to 0.9 mL for more accurate rate measurements. A molar absorptivity value of 6220 was used to convert NADH absorbance changes to µmol of product formed. One AK enzyme unit (eu) of activity is defined as 1 µmol of NAD+ formed per minute.
Standard RPFK-1 assay
We measured RPFK-1 activity, F 6-P + ATP = F 1,6-BP, with a modification of the method by Anderson et al.Citation13. A 1.0 mL assay mixture contained 2 mM fructose 6-phosphate; 1 mM ATP (A 7699); 3 mM MgCl2; 0.13 mM NADH (N 1161); 1.7 eu/mL glyceraldehyde 3-phosphate dehydrogenase (G 0763); 18 eu/mL triose phosphate isomerase (G 1881); 1.3 eu/mL aldolase (A 8811); and 10 mM Tris-phosphate buffer, pH 8.0, as final concentrations.
30 nM RPFK-1 assay
Assay components from the standard 1-phosphofructokinase assay above were concentrated to 10 times the final assay concentrations into 0.1 mL9. Other conditions were the same as in the 30 nM RMAK assay above. A molar absorptivity value of 6220 was used to convert NADH absorbance changes to µmol of product formed. One PFK-1 enzyme unit (eu) of activity is defined as 1 µmol of NAD+ formed per minute.
Aldolase assay
Reagents for the measurement of aldolase activity were the same as in the standard RPFK-1 assay above, except that 2 mM fructose 6-phosphate and 1 mM ATP were omitted and replaced by 2 mM fructose 1,6-bisphosphate. One enzyme unit (eu) of aldolase activity is defined as 1 µmol of NAD+ formed per minute.
Dilutions of RPFK-1 and RMAK to 30 nmol
Standard procedures for preparing all low concentrations of all RPFK-1 and RMAK preparations were as follows unless stated otherwise. Stock solutions of purifiedCitation5 3 µM RPFK-1 (1.7 eu/mL) in 0.01 M Tris-phosphate, pH 8, and commercial 3 µM RMAK (15.6 eu/mL) in 0.01 M potassium phosphate, pH 8, were diluted with their respective buffers to final concentrations, usually 30 nM, 70 nM, 140 nM, or 200 nM PFK-1, and allowed to stand at 25°C for at least 0.5 h to allow activity losses due to dilution to stabilize; activities remained constant for more than 2 h under these conditionsCitation3,Citation4. Additions to RPFK-1 diluted samples were usually accomplished by adding 1/20 volume of test sample at a 20-fold concentration of desired final concentration. Test samples were then incubated at 25°C for 1 h and RPFK-1 activity was then determined. The losses of RPFK-1 and RMAK activity due to dilution under these conditions were previously reportedCitation1.
Time course of inhibitions by lithium salt
PFK-1 was mixed with additions as described above and activities measured at indicated times. Under our conditions, it was determined that no significant changes in inhibition levels occurred after 1 h of the additions and they were stable beyond 2 h.
Lithium salt titrations against constant RPFK-1
Fixed RPFK-1 concentrations, with varied final lithium salt concentrations as indicated, were incubated 0.5 h before activities were determined.
RPFK-1 titrations against constant lithium salt concentration
Fixed lithium salt concentrations with varying PFK-1 were incubated 1 h before activities were determined.
Measurements of protein concentrations
PFK-1 protein concentrations during purification procedures were determined using the following formula: mg protein/mL = 1.55 A280 – 0.76 A260, where A280 and A260 are absorbencies at 280 nm and 260 nm, respectivelyCitation14. The spectrophotometric protein determinations were comparable to the Bradford methodCitation15.
Results
Effect of lithium salts on 30 nM RMAK and rabbit muscle LDH
shows that chloride, sulfate, and carbonate salts of lithium did not inhibit 30 nM RMAK but instead showed increased activity above the losses due to dilution, similar to that previously shown for ammonium, potassium, and sodium sulfatesCitation1. The same lithium salt concentrations had little or no effect, neither stimulatory nor inhibitory, on 30 nM rabbit muscle LDH activity (not shown).
Figure 1. Effect of lithium salts on 30 nM RMAK. The 30 nM RMAK was diluted from a 3 µM RMAK stock in 0.01 M potassium phosphate, pH 8 buffer. The activity of RMAK was control, 0.016 eu/mL. The diluted preparations were incubated for 1 h at 25°C and the activities were then determined (see “Methods”).
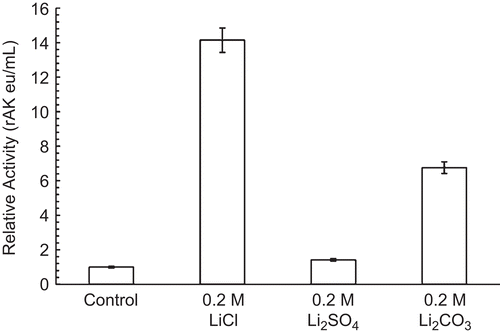
Effect of lithium chloride, sulfate, and carbonate on 30 nM RPFK-1 activity
shows that both inhibitions and levels depended upon the anion. LiCl did not inhibit 30 nM RPFK-1 at the concentrations and conditions given, while Li2SO4 and Li2CO3 did inhibit; Li2CO3 inhibited considerably more than Li2SO4.
Figure 2. Effect of lithium anions on 30 nM RPFK-1 activity. A 30 nM RPFK-1 was prepared as given in the “Methods” section containing the quantities of lithium salts shown. The preparations were incubated for 1 h at 25°C and the activities were then determined. The RPFK-1 activity of the control was 0.018 ± 0.022 eu/mL.
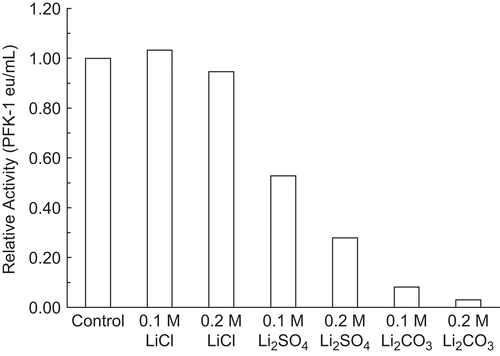
Effect of substrates on Li2CO3 inhibitions of 30 nM RPFK-1
Given that ATP-Mg offers a protective effect during the heat step of the RPFK-1 purificationCitation10, the possibility that substrates protected against Li2CO3 inhibition was investigated. shows that each substrate at 100 mmol offered no protective effect and slightly augmented Li2CO3 inhibitions. Under similar conditions, the presence of 2 mM ATP-Mg or 2 mM F 6-P was indistinguishable from the control.
Figure 3. Effect of substrates on 30 nM RPFK-1 inhibition by Li2CO3. A 30 nM RPFK-1 control (▪) with no additions was prepared as given in the “Methods” section containing the quantities of lithium salts shown. The final additions to the control shown are 100 mM F 6-P (•), and 100 mM ATP-Mg (○). In the absence of Li2CO3 average activities were as follows: control, 0.020 eu/mL; plus F 6-P, 0.018 eu/mL; and plus ATP-Mg, 0.015 eu/mL.
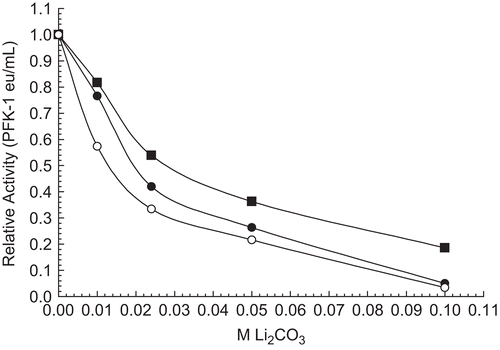
Effect of 5 µM aldolase on PFK-1 inhibition by Li2CO3
PreviouslyCitation8, rabbit muscle aldolase was shown to protect 30 nM RPFK-1 from activity losses due to dilution and activity losses due to inhibition by ascorbate. shows that an initial presence of 5 µM aldolase completely protected 30 nM RPFK-1 from 25% inhibition by 20 mM Li2CO3 and from 60% inhibition by 40 mM RPFK-1. Similar results can be shown with purified beef muscle PFK-1.
Figure 4. Effect of 5 µM aldolase on PFK-1 inhibition by Li2CO3. A 30 nM RPFK-1 was prepared as given in the “Methods” section containing the quantities of lithium salts shown. The preparations were incubated for 1 h at 25°C and the activities were then determined. The RPFK-1 activity of the control was 0.020 ± 0.002 eu/mL.
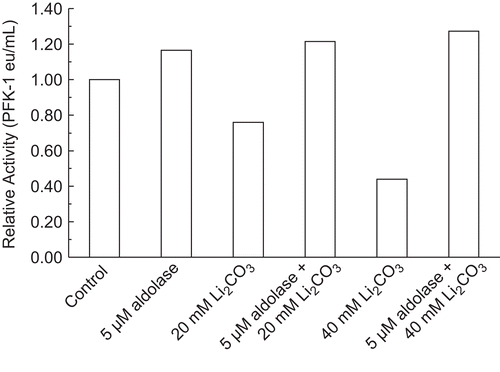
Effect of RPFK-1 concentration on inhibition by 0.075 M Li2CO3
shows that inhibitory effects of 0.075 M Li2CO3 depended upon RPFK-1 concentrations. With respect to the differential effects of Li2CO3 and based on previous studiesCitation1, we believe that inhibition of 30 nM PFK-1 reflects a characteristic of dimeric RPFK-1 and that stimulation of 200 nM RPFK-1 reflects a characteristic of tetrameric PFK-1. Similar results can be shown for purified beef muscle PFK-1.
Figure 5. Effect of RPFK-1 concentration on inhibition by 0.075 M Li2CO3. Concentrations of RPFK-1 were prepared by dilutions of a 3 µmol stock as given in the “Methods” containing 0.075 M LiCO3 or not (controls) as shown. Preparations were incubated for 1 h at 25°C and the activities were then determined. The RPFK-1 activity of the control was 0.020 ± 0.002 eu/mL. The RPRK-1 activities of stock controls were as follows: 30 nmol, 0.017 eu/mL; 100 nmol, 0.044 eu/mL; and 200 nmol, 0.29 eu/mL.
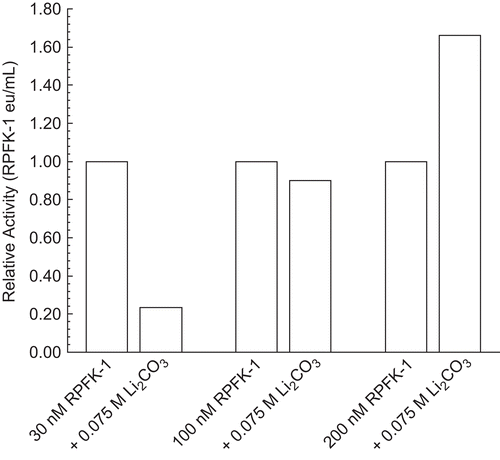
Effect of potassium and sodium salts on 30 nM RPFK-1 activity
The amount of RPFK-1 inhibition by monovalent salts depended on the combination of both the cation and the anion and not on one or the other. The inhibitions of 30 nM RPFK-1 by potassium or sodium salts with chloride, carbonate, and phosphate shown in illustrate these points. Potassium chloride (□) and potassium phosphate (Δ) inhibit more than the corresponding sodium salts, but the potassium carbonate (○) and sodium carbonate (•) inhibit equivalently, suggesting that anions have an influence on the inhibitions. Again, both potassium chloride (□) and potassium phosphate (Δ) inhibit equivalently, even though the potassium ion concentration in phosphate is twice that in chloride, suggesting that anions influence inhibitions; a similar situation prevails for sodium chloride (▪) and sodium phosphate (▴).
Figure 6. Effect of potassium and sodium salts on 30 nM RPFK-1 activity. The figure is a composite of several experiments using the average of 0.017 eu/mL as the control value for relative activities of 1.00. The 30 nM RPFK-1 solutions were made as given in the “Methods” section, incubated for 1 h; solutions were then made to salt concentrations shown, with an additional 1 h incubation, when RPFK-1 activities were determined. All solutions were maintained at pH 8. The symbols for the salts are as follows: KCl, □; K2CO3, ○; K2HPO4, Δ; NaCl, ▪; Na2CO3, •; and Na2HPO4, ▴.
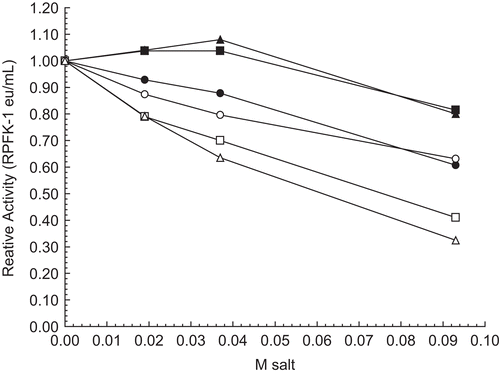
Effects of acetate salts on 30 nM RPFK-1 activity
The effects of acetate salts of sodium (▪), lithium (•), and potassium (○) in show how varied the effects by monovalent cations can be when compared with the effects of sodium, lithium, and potassium carbonate, chloride, phosphate, and sulfate salts illustrated in this article () and elsewhereCitation1. Lithium acetate slightly stimulates 30 nM RPFK-1, while sodium acetate inhibits slightly and potassium acetate has no effect.
Figure 7. Effect of acetate salts on 30 nM RPFK-1 activity. The figure is a composite of several experiments using the average of 0.010 eu/mL as the control value for relative activities of 1.00. The 30 nM RPFK-1 solutions were made as given in the “Methods” section, incubated for 1 h; solutions were then made to salt concentrations shown, with an additional 1 h incubation, when RPFK-1 activities were determined. All solutions were maintained at pH 8. The symbols for the salts are as follows: sodium acetate, ▪; lithium acetate, •; and potassium acetate, ○.
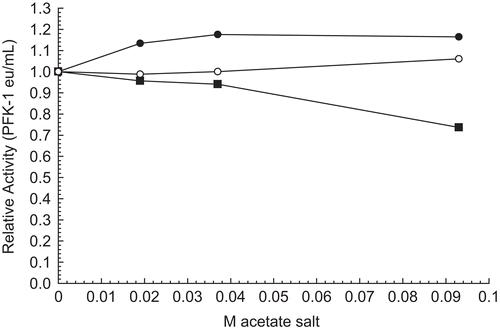
Table 1. Percent inhibition of 30 nM RPFK-1 by 0.1 M monovalent salt.
summarizes the effects of lithium, potassium, and sodium salts on 30 nM RPFK-1. The feature that stands out is the variation in effects on RPFK-1 activity both horizontally by cations and vertically by anions.
Discussion
Initiation of these lithium salt studies was prompted by inhibitions of RPKF-1 by monovalent salts, ammonium, potassium, and sodium sulfateCitation1. Lithium salts were of interest because of their use in manic–depressive disorder therapyCitation16,Citation17. Lithium salts and their effect on RPFK-1, the putative controlling enzyme for the major energy pathway for the brain, became a focus of this study. The wide variations of inhibitions of 30 nM RPFK-1 by salts of lithium, potassium, and sodium were unexpected. Potassium is the prevalent intracellular cation, so it was also unexpected that the potassium salts were more potent inhibitors of 30 nM RPFK-1 than the corresponding sodium saltsCitation1,Citation8,Citation9 (). shows that K2HPO4 and KCl are more potent inhibitors of 30 nM RPFK-1 than Na2HPO4 and NaCl, suggesting that the potassium ion is a more potent inhibitor than the sodium ion under similar conditions. , , and show that the extent of RPFK-1 inhibitions by lithium salts varied with the anion. For example, shows that LiCl is not an inhibitor, ostensibly ruling out the lithium ion and chloride ion as inhibitors. shows also that Li2CO3 is the more potent inhibitor of 30 nM RPFK-1 compared with Li2SO4. However, K2CO3 and Na2CO3 inhibit 30 nM RPFK-1 equivalently, suggesting that the carbonate anion mitigates inhibition by K+ and/or boosts inhibition by Na+. Our results suggest that the extent of inhibitions was due to an inhibiting contribution by carbonate. shows the modifying effects of anions on the extent of inhibition by the lithium ion. In this instance, lithium acetate •) slightly stimulated, potassium acetate (○) had no inhibitory effect, and sodium acetate (▪) was slightly inhibitory. From our results, summarized in , it appears that both cations and anions need to be considered before an inhibition can be credited to one or the other ion. For example, as shown in , that potassium chloride is an inhibitor of RPFK-1 might indicate that the potassium cation is an inhibitor, but the results with potassium acetate would suggest that potassium is not an inhibitor. Similarly, lithium chloride is not an inhibitor, suggesting that neither lithium nor chloride is an inhibitor. Carbonate and sulfate anions appear to be consistent inhibitors but inhibitions vary widely, depending on the cation. The literature is replete with references to “lithium” with little or no apparent references to the anion; if a lithium salt inhibits, then the tacit assumption is that the lithium cation is the inhibitor.
Assumptions that the lithium cation is the inhibitor probably draw from experiments carried out between pH 7 and pH 8, where the net charge of most proteins is negative, but proteins are amphoteric, possessing both positive and negative sites. It is possible that different anions interact with positively charged sites and different cations with the negatively charged sites on RPFK-1 with varying degrees of affinity, altering conformation or facilitating addition of the lithium cation, either or both of which could alter enzyme activity. It is proposed, as an example, that positively and negatively charged protein sites with differing affinities for different anions and cations, respectively, account then for the different effects on enzyme activities in the presence of the same cation. It follows then from our studies that lithium per se would not be the inhibitor; the inhibitor would be the lithium salt—both the lithium cation and its anion. shows that RPFK-1 concentrationCitation1 also alters its reaction to lithium carbonate: the dimeric form of 30 nM RPFK-1 was inhibited while the tetrameric form of 200 nM RPFK-1 was stimulated. Discussion of the possibility of low concentrations of PFK-1 in the cytosol and sequestration of PFK-1 by cellular infrastructures was presented previouslyCitation1.
In summary, we showed that the inhibition of 30 nM RPFK-1 by monovalent salts of lithium, potassium, and sodium differed widely, depending upon the associated anion. In our opinion, in vitroCitation18–26 studies of enzyme inhibitions by salts, including lithium, should not assign the cation as the sole inhibitor without examination of the effects of several other associated anions. For example, drawn from PFK-1 kinetic studies with Li2CO3, it was reportedCitation18 that lithium (ion) was competitive with the ATP-Mg complex and non-competitive with F 6-P. Our report allows the possibility that the carbonate anion was the inhibitor, since lithium acetate () and lithium chloride () were not inhibitors. Studies with cell cultures, whole organs, or whole animalsCitation17,Citation19,Citation27–41 offer a more difficult order of control and interpretation of results, but given the influence of the anion on the effect of lithium salts to inhibit or stimulate ( and ), we believe that more than one anion of lithium salts should be explored.
Acknowledgments
The project/study described was partially supported by Grant Number P60 MD00220 from the San Diego EXPORT, NCMHD, NIH; by Grant Number 1 R25 GM73590 from the UCSD CURE Program, National Cancer Institute, NIH; and by Grant Number R25 GM083275 UCSD IMSD Program National Institute of General Medical Sciences, NIH. The contents are solely the responsibility of the authors and do not necessarily represent the official views of the National Institutes of Health.
References
- Russell P, Williams A, Marquez K, Hua T, Ehya F, Hardamon C, et al. Effect of ammonium, sodium, and potassium ions on rabbit muscle phosphofructokinase-1 and adenylate kinase activities, J Enzyme Inhib Med Chem 2009;24;1–7.
- Schou M, Juel-Nielsen N, Stromgren E, Voldby H. The treatment of manic psychoses by the administration of lithium salts. J Neurol Neurosurg Psychiatry 1954;17:250–60.
- Cade J. Lithium salts in the treatment of psychotic excitement. Med J Aust 1949;2:349–52.
- Johnson FN, Cade JFJ. The Historical Background to Lithium Research and Therapy. London: Academic Press, 1975:9–22.
- Russell PJ, Williams A, Gapuz D. Inhibition of rabbit muscle adenylate kinase by vitamin C. Biochem Biophys Res Commun 1997;233:386–8.
- Russell PJ, Williams A, Austin TA. Inhibition of rabbit muscle isozymes by vitamin C. J Enzyme Inhib 2000;15:283–96.
- Russell PJ, Williams A, Amador X, Vargas R. Aldolase and actin protect rabbit muscle lactate dehydrogenase from ascorbate inhibition. J Enzyme Inhib 2004;19:91–8.
- Russell PJ, Williams A, Abbott A, DeRosales B, Vargas R. Characteristics of rabbit muscle adenylate kinase inhibition by ascorbate. J Enzyme Inhib Med Chem 2006;21:61–7.
- Russell P, Williams A, Marquez K, Tahir Z, Hosseinian B, Lam K. Some characteristics of rabbit muscle phosphofructokinase-1 inhibition by ascorbate. J Enzyme Inhib Med Chem 2008;23:411–17.
- Kemp RG, Wood W, eds. Phosphofructokinase from rabbit skeletal muscle. Methods Enzymol 1975;42C:71–7.
- Morrissey JH. Silver stain for proteins in polyacrylamide gels: a modified procedure with enhanced uniform sensitivity. Anal Biochem 1981;117:307–10.
- Adam H. Methods of Enzymatic Analysis. New York:Academic Press, 1963:145–52.
- Anderson RL, Hanson TE, Sapico VL. D-Fructose-1-phosphate kinase A. aerogenes. J Biol Chem 1969;244:6280–8.
- Wilson K, Walker J. Principles and Techniques of Practical Biochemistry. Cambridge: Cambridge University Press, 2001:230.
- Bradford MM. Determination of protein concentration in the manufacture and characterization of biopharmaceuticals. Anal Biochem 1976;72:248–54.
- Struneck A, Patočka J, Sarek M. How does lithium mediate its therapeutic effects? J Appl Biomed 2005;3:25–35.
- Goldberg H, Clayman P, Skorecki K. Mechanism of Li inhibition of vasopressin-sensitive adenylate cyclase in cultured renal epithelial cells. J Physiol 1988;255:F995–1002.
- Kajda PK, Birch NJ. Lithium inhibition of phosphofructokinase. J Inorgan Chem 1981;14:275–8.
- Nordenberg J, Kaplansky M, Beery E, Klein S, Beitner R. Effects of lithium on the activities of phosphofructokinase and phosphoglucomutase and on glucose-1,6-diphosphate levels in rate muscles and liver. Biochem Pharm 1982;31:1025–31.
- Mann L, Heldman E, Shabltiel G, Belmaker RH, Agam G. Lithium preferentially inhibits adenylyl cyclase V and VII isoforms. Int J Neuropsychopharmacol 2008;11:533–9.
- López-Coronado JM, Bellés JM, Lesage Dagger F, Serrano R, Rodríguez PL. A novel mammalian lithium-sensitive enzyme with a dual enzymatic activity, 3’-phosphoadenosine 5’-phosphate phosphatase and inositol-polyphosphate 1-phosphatase. J Biol Chem 1999;274:16034–9.
- Hallcher LM, Sherman WR. The effects of lithium ion and other agents on the activity of myo-inositol-1-phosphatase from bovine brain. J Biol Chem 1980;255:10896–901.
- Jope RS. Lithium and GSK-3: one inhibitor, two inhibitory actions, multiple outcomes. Trends Pharmacol Sci 2003;24:441–3.
- Beyaert R, Vanhaesebroeck B, Suffys P. Van Roy F, Fiers W. Lithium chloride potentiates tumor necrosis factor-mediated cytotoxicity in vitro and in vivo. Proc Natl Acad Sci USA 1989;86:9494–8.
- King TD, Bijur GN, Jope RS. Caspase-3 activation induced by inhibition of mitochondrial complex I is facilitated by glycogen synthase kinase-3β and attenuated by lithium. Brain Res 2001;919:106–14.
- Zhang F, Phiel CJ, Spece L, Gurvich N, Klein PS. Inhibitory phosphorylation of glycogen synthase kinase-3 (GSK-3) in response to lithium. Evidence for autoregulation of GSK-3. J Biol Chem 2003;278:33067–77.
- Rodriguez-Gil JE, Fernandez JM, Barbera A, Guiovart JJ. Lithium’s effects on rat liver glucose metabolism in vivo. Arch Biochem Biophys 2000;375:377–84.
- Klein PS, Melton DA. A molecular mechanism for the effect of lithium on development. Proc Natl Acad Sci USA 1996;93:8455–9.
- Centeno F, Mora A, Fuentes JM, Soler G, Claro E. Partial lithium-associated protection against apoptosis induced by C2-ceramide in cerebellar granule neurons. Neuroreport 1998;9:4199–203.
- Schotte P, Van Loo G, Carpentier I, Vandenabeele P, Beyaert R. Lithium sensitizes tumor cells in an NF-κB-independent way to caspase activation and apoptosis induced by tumor necrosis factor (TNF). Evidence for a role of the TNF receptor-associated death domain protein. J Biol Chem 2001;276:25939–45.
- Hongisto V, Smeds N, Brecht S, Herdegen T, Courtney MJ, Coffey ET. Lithium blocks the c-Jun stress response and protects neurons via its action on glycogen synthase kinase 3. Mol Cell Biol 2003;23:6027–36.
- De Sarno P, Li X, Jope RS. Regulation of Akt and glycogen synthase kinase-3β phosphorylation by sodium valproate and lithium. Neuropharmacology 2002;43:1158–64.
- Bijur GN, De Sarno P, Jope RS. Glycogen synthase kinase-3β facilitates staurosporine- and heat shock-induced apoptosis. Protection by lithium. J Biol Chem 2000;275:7583–90.
- Jope RS, Bijur GN. Mood stabilizers, glycogen synthase kinase-3β and cell survival. Mol Psychiatry 2002;7(Suppl. 1):S35–45.
- Chuang DM, Chen RW, Chalecka-Franaszek E, Ren M, Hashimoto R, Senatorov V, et al. Neuroprotective effects of lithium in cultured cells and animal models of diseases. Bipolar Disord 2002;4:129–36.
- Alvarez G, Munoz-Montano JR, Satrustegui J, Avila J, Bogonez E, Diaz-Nido J. Lithium protects cultured neurons against β-amyloid-induced neurodegeneration. FEBS Lett 1999;453:260–4.
- Wei H, Leeds PR, Qian Y, Wei W, Chen R, Chuang D. β-Amyloid peptide-induced death of PC12 cells and cerebellar granule cell neurons is inhibited by long-term lithium treatment. Eur J Pharmacol 2000;392:117–23.
- Nonaka S, Chuang DM. Neuroprotective effects of chronic lithium on focal cerebral ischemia in rats. Neuroreport 1998;9:2081–4.
- Ren M, Senatorov VV, Chen RW, Chuang DM. Postinsult treatment with lithium reduces brain damage and facilitates neurological recovery in a rat ischemia/reperfusion model. Proc Natl Acad Sci USA 2003;100:6210–15.
- Klein PS, Melton DA. A molecular mechanism for the effect of lithium on development. Proc Natl Acad Sci USA 1996;93:8455–9.
- Beyaert R, Heyninck K, De Valck D, Boeykens F, van Roy F, Fiers W. Enhancement of tumor necrosis factor cytotoxicity by lithium chloride is associated with increased inositol phosphate accumulation. J Immunol 1993;151:291–300.