Abstract
Glutathione transferase P1-1 is over expressed in some cancer cells and contributes to detoxification of anticancer drugs, leading to drug-resistant tumors. The inhibition of human recombinant GSTP1-1 by natural plant products was investigated using 10 compounds isolated from plants indigenous to Southern and Central Africa. Monochlorobimane and 1-chloro-2,4-dinitrobenzene were used to determine GST activity. Each test compound was screened at 33 and 100 µM. Isofuranonapthoquinone (1) (from Bulbine frutescens) showed 68% inhibition at 33 µM, and sesquiterpene lactone (2) (from Dicoma anomala) showed 75% inhibition at 33 μM. The IC50 value of 1 was 6.8 μM. The mode of inhibition was mixed, partial (G site) and noncompetitive (H site) with Ki values of 8.8 and 0.21 µM, respectively. Sesquiterpene 2 did not inhibit the CDNB reaction. Therefore, isofuranonapthoquinone 1 needs further investigations in vivo because of its potent inhibition of GSTP1-1 in vitro.
Abbreviations | ||
CDNB | = | 1-chloro-2,4-dinitrobenzene |
DTT | = | dithiothreitol; GSH, glutathione |
GST | = | Glutathione transferase, isopropylthiogalactoside |
JNK | = | c-jun N-terminal kinase-1; PM |
Introduction
Natural products have been in use since ancient times as medicines and spices, and the use of herbal remedies and dietary supplements is ever increasingCitation1,Citation2. With this resurgence of natural products, the focus on the interaction of the latter with xenobiotic metabolizing enzymes has received increased attention. For instance, cytochrome P450 enzymes have been found to interact with commonly used herbsCitation3, and flavonoids have been shown to inhibit glutathione S-transferases in blood plateletsCitation4. These characteristics suggest that phytochemicals may have important pharmacological and toxicological consequencesCitation5.
During treatment of many cancers, there is often a development of drug resistance in a tumor that was originally sensitive to treatment resulting in a phenomenon known as multidrug resistance (MDR)Citation6. Many mechanisms are involved in MDR, and these include alterations in drug transport resulting in impaired entry or enhanced efflux of the drug from the tumor cell, enhanced DNA repair, alterations in target proteins, and alterations in drug metabolismCitation7. The glutathione transferases (EC 2.5.1.18: GST) are a unique family of detoxification enzymes comprising a large group of cytosolic, mitochondrial, and microsomal proteins which are capable of multiple reactions with a multitude of substrates, both endogenous and xenobioticCitation8. These enzymes can constitute up to 10% of cytosolic protein in some mammalian organs and play an important role in the detoxification of electrophilic xenobiotics such as, drugs, toxins, and carcinogens allowing the products to be exported from the cell through the GS-X pump in an ATP-dependent mannerCitation9. Besides catalysing the inactivation of various electrophile-producing anticancer agents via conjugation to the tripeptide glutathione, some cytosolic proteins belonging to the glutathione transferase superfamily are emerging as negative modulators of stress/ drug-induced cell apoptosis through the interaction with specific signalling kinasesCitation10. GST P1 is over expressed in cancer cells; hence, it is regarded as a prognostic factor in cancer treatmentCitation11. Cancer cells and normal cells are known to respond differently to nutrients and drugs that affect glutathione status. Numerous studies have shown that tumor cells have elevated levels of glutathione levels, which confers resistance to chemotherapeutic drugsCitation12. The genes coding for GST Pi are up regulated during early stage oncogenesis and significantly over expressed in human tumors. The high levels of GST Pi that result are associated with anti-cancer drug resistance and poor cancer patient survivalCitation13.
This study focuses on the interaction of glutathione transferase Pi (GST Pi) and natural products isolated from Dicoma anomala (Asteraceae), Bulbine frutescens (Asphodelaceae), Plumeria rubra (Apocynaceae), Dorstenia elliptica, Treculia africana (Moraceae), Garcinia smeathmannii, and Mammea africana ((Clusiaceae or Guttiferae). Dicoma anomala is a small shrub whose common English names are fever bush and stomach bush. It is widely distributed in sub-Saharan Africa. Root decoctions are administered orally to children believed to be suffering from blood disordersCitation14. The aerial parts of this plant collected from Namibia have been shown to contain several sesquiterpene lactonesCitation15. G. smeathmannii is an evergreen tree commonly found in southern and central Africa. It is used to treat eye inflammation, scabies, wounds, and stomach painCitation16. The isolation of xanthones and poly-prenylated benzophenone derivatives from G. smeathmannii as well as the antimicrobial and antioxidant properties of these compounds have been reported recentlyCitation17,Citation18. Bulbine frutescens is an ornamental herb that grows widely in Africa. It is also used medicinally to enhance the healing of wounds. The roots of B. frutescens are good sources of phenylanthraquinones and isofuranonaphthoquiones which are reported to possess anti-plasmodial and antimicrobial agentsCitation19. T. africana, commonly known as African bread fruit, is used in folk medicine against skin diseases and dental allergies. Two flavonol derivatives were isolated from the leaves of T. Africana. One of these (6) is shown to possess antimicrobial propertiesCitation20. Dorstenia elliptica Bureau, an undergrowth perennial plant, is used in the treatment of many diseases, especially, for eye infections. Phytochemical investigation of the twigs of D. ellipitca resulted in the isolation of several coumarins including compound 4. The crude extracts as well as compound 4 are reported to have antimicrobial propertiesCitation21. P. rubra, commonly known as Red Frangipani, is a spreading shrub or small tree to a height of 7–8 m and wide flushed with fragrant flowers. It is widely cultivated in subtropical and tropical climates worldwide. It is reported to contain triterpenesCitation22 alkaloidsCitation23, and other cytotoxic compoundsCitation24.
Mammea africana is a large forest tree commonly known as the African apple, African apricot, and African mammey apple. Extracts from this plant consist mainly of coumarin derivatives which are known to exhibit a number of bioactivities such as insecticidal, antioxidant, anticancer, antibacterial, antimicrobial, and antibiotic activitiesCitation25.
Multidrug resistance is often associated with decreased intracellular drug accumulation in a patient’s tumor cells due to enhanced drug efflux or enhanced metabolism via GSTsCitation26. Therefore, there is an urgent need to find replacement for drugs previously used or to find suitable chemo-modulators in order to reverse drug resistanceCitation27. Several natural products have been identified as possible anticancer agents that exhibit antimutagenic and antiproliferative characteristicsCitation28. Seventy percent of all present antileukemia drugs have been derived from natural products or their derivativesCitation29. Doxorubicin, vinblastine, and vincristine represent some of the current standard chemotherapeutic drugs that have been isolated from plants and used in the treatment of solid and blood cancersCitation30. The use of GST inhibitors as therapeutic agents has been proved to be useful in endeavours to modulate anticancer drug resistanceCitation31. Natural products that are potent GST P1-1 inhibitors may have possible uses in chemomodulation and cancer therapy given the role that the elevated GST P1-1 levels play in cancer proliferation and progression. The aim of this study was to evaluate novel natural products as inhibitors of glutathione transferase P1-1 from the plants Dicoma anomala, B. frutescens, P. rubra, Dorstenia elliptica, T. africana, G. smeathmannii, and M. africana. This evaluation was aimed at searching for potential effective inhibitors of GSTs that could augment the cytotoxic effects of alkylating anticancer drugs in the case where these enzymes are involved in alkylating anticancer drug resistance.
Materials and methods
Reagents and chemicals
The substrates 1-chloro-2,4 dinitrobenzene (CDNB), monochlorobimane (MCB), and other chemicals and reagents were obtained from Sigma Chemical Company and Aldrich Chemical Company (St Louis, MO, USA). The natural product compounds used in this study were obtained from Professor Berhanu Abegaz (University of Botswana, Botswana). The compounds and the plants from which they are isolated are as follows. The isofuranonaphthoquinone (1) was extracted from B. frutescensCitation32, the sesquiterpene lactone 2 was extracted from Dicoma anomalaCitation33, iridoid 3 was extracted from P. rubraCitation34, furocoumarin (4) was obtained from Dorstenia ellipticaCitation35, and benzophenone derivative (5) was extracted from G. smeathmanniiCitation36. Flavanol 6 was isolated from T. africanaCitation37. The xanthones (7 and 9) and coumarin (8) were obtained from M. africanaCitation38–40. The natural products were extracted from the above-mentioned plants using the following general protocol. The sun-dried plant material (ca 1 kg) was soaked in a mixture of dichloromethane–methanol (1:1) and pure methanol for 24 h and 2 h, respectively, at room temperature. Concentration of the combined organic extract gave a residue (ca 50–65 g). Part of this residue was chromatographed on a silica gel column eluting with hexane–ethyl acetate mixtures, to give fractions of 250 ml each. The fractions were concentrated and monitored by TLC and 1H NMR, and similar fractions were combined. The first fractions examined by TLC (hexane–ethyl acetate; 9:1) contained mainly mixtures of hydrocarbons and phytosterols, which were not investigated further. More polar fractions were passed through Sephadex LH-20 column (CHCl3–methanol, 2:1). The post-chlorophyll fractions were subjected to repeated silica gel CC and PTLC to yield the various metabolites. Pure metabolites’ molecular structures were established by spectroscopic techniques such as NMR, MS, and IR. The structures of the chemicals used are shown in . All the other chemicals used were of the highest purity obtained from different sources. The structures of the compounds used are shown in . Escherichia coli cells with the gene for human GST P1-1 were obtained from Professor Bengt Mannervik (Department of Biochemistry, Uppsala University, Sweden).
Expression and purification of recombinant glutathione S-transferases Pi
Recombinant human P1-1 were expressed in E. coli and purified as described by Mukanganyama et al. (35). A 100 ml portion of 2TYA medium (54 g tryptone, 40.5 g yeast extract, 13.5 g NaC1 and 27 g glycerol in 2 700 ml water) containing 13.5 μl ampicillin (1 M stock) was inoculated with 20 μl of the E. coli cells. The culture was incubated in a shaking incubator (Labcon, Labotec, South Africa) operating at 170 rpm and 37°C for 20 h. Three 2000-ml conical flasks containing 500-ml 2TYA medium and 67.5 μl of 1-M ampicillin were inoculated with 5 ml of the culture and incubated in a shaking incubator at the same settings for 22 h. The bacteria were sedimented, lysed and GSTs purified, affinity chromatography on an S-hexylglutathione Sepharose 6B (Pharmacia, Uppsala, Sweden) affinity gel. The activity of the enzyme was determined using CDNB as substrateCitation40 and protein content was determined using the Lowry procedureCitation41.
The purity of the enzyme purification fractions was determined by sodium dodecyl sulphate polyacrylamide gel electrophoresis (SDS–PAGE), carried out on 15% slab gels using a Hoeffer SE Mighty Small II electrophoresis system (Hoeffer Scientific Instruments, CA, USA). Protein bands were stained with Coomasie Blue-G.
Screening for Inhibition by Natural Products
Compounds 1–10 () were screened for inhibition of the major human cytosolic P1-1. First, the effects of NPs were determined on GSTs using monochlorobimane as a substrate for GST. A Shimadzu UV-1501 spectrophotofluorometer (Shimadzu Corporation, Kyoto, Japan) in the kinetics mode was used for the assay. The excitation wavelength was set at 390 nm and the emission wavelength was 478 nm. For all the readings, a concentration of 0.24 µg/ml of GST P1-1 was used and the final concentrations of MCB and GSH were 4 µM and 0.5 mM, respectively. Inhibition by the compounds was tested at 100 μM and 33.3 μM final concentrations from stock concentrations of 2.5 mM and 0.833 mM prepared in dimethyl sulfoxide (DMSO). Compounds that were found to be potent inhibitors using the MCB assay were also tested for inhibitory activity using CDNB as a substrate (). The assay with CDNB was adapted for measurement of absorbance with a SpectraMax 340 microplate spectrophotometer equipped with a kinetics mode (Molecular Devices, Sunnyvale, CA, USA). All compounds were used in the concentration range of 0–100 μM and were dissolved in 95% ethanol or dimethylsulfoxide. The final concentration of each solvent in the inhibition assays was 2.5%, and this concentration had no effect on activity of the GSTs (data not shown). The concentration of natural product required to bring about 50% inhibition of GST activity, the IC50 value, was determined by plotting sigmoidal dose–response curves of enzyme activity vs. log natural product concentration using GraphPad Prism version 4.00 for Windows (GraphPad™ Software Inc., San Diego, CA, USA).
Figure 2. The chemical reactions of CDNB and MCB catalysed by GST P1-1.The monochlorobimane fluorescence assay for GST activity was used to measure the product of conjugation at the excitation wavelength 390 nm and the emission wavelength of 478 nm. The CDNB spectrophotometric assay for GST activity was used to determine the conjugation product 2, at a wavelength of 340 nm.
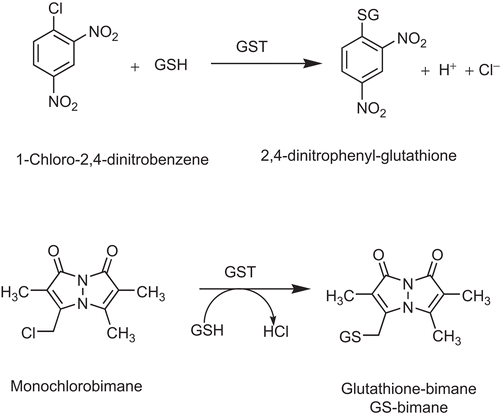
The effects of isofurano-napthoquinone 1 and sesquiterpene 2 on the kinetics of GSTs were determined as described by Mukanganyama et al.,Citation35. The Km(app) and Vmax(app)were determined using GraphPad Prism™ version 4.00 for Windows. The Ki values with respect to GSH and CDNB, as well as the type of inhibition, were determined. The type of inhibition was deduced by determination of trends of Km and Vmax values with an increase in natural product concentration. To determine the trend, the means of the Km (or Vmax) values with increase in inhibitor concentration were compared by performing a one-way ANOVA with Dunnett’s post-test using GraphPad InStat™ version 3.00 for Windows 95 (GraphPad™ Software, Inc.). The inhibition constant, Ki, was determined by means of re-plotsCitation35. The type of re-plot depends on the type of inhibition, for example, plotting 1/Vmax versus inhibitor concentration for noncompetitive inhibition, will give Ki as the intercept on the baselineCitation43.
Results
Purification of heterologously expressed GSTs
Human GST P1-1 was expressed heterologously in E. coli and was purified by affinity chromatography. The GSTs were purified to homogeneity and a single band was obtained on SDS–PAGE analyses (data not shown) and the specific activity was 160 μmol/min/mg protein. This value is comparable to the value of 129 μmol/min/mg obtained by Hayeshi et al.Citation44
Effects of the natural products on GST activity
The effects of compounds 1–10 on the activity of human recombinant GST activity were assessed by measuring the conjugation activity with MCB and CDNB. The inhibition profile when using MCB as a substrate is shown in . Isofuranonaphthoquinone 1, sesquiterpene lactone 2, and xanthone 7 were potent inhibitors of the enzyme. The other compounds, the monoterpene-substituted furocoumarin 4, the benzophenone derivative 5, the 4-phenyl coumarin derivative 8, and xanthone 9 inhibited the enzyme but to a lesser extent even at the highest concentration of 100 μM. Iridoid 3 was, however, shown to activate the enzyme at 100 µM. Thus, the compounds 1 and 2 were found to be potent GST P1-1 inhibitors. For this reason, their potential inhibitory activity was also assessed using CDNB as a substrate to confirm if there was any substrate-dependent inhibition. The results showed that 2 did not inhibit the CDNB reaction, as it did for the MCB conjugation but activation at 19 and 5% at 33 and 100 μM, respectively was shown (). Isofuranophthoquinone 1 displayed a high level of potency with both CDNB and with MCB as substrates. The reaction was inhibited by 87% and 100% at 33 and 100 μM, respectively. The IC50 for 1 (6.8 μM) was determined photometrically using CDNB as the substrate. shows the sigmoidal dose–response curve for the determination of the IC50 for 1 using CDNB as a substrate.
Table 1. Percentage inhibition of GST P1-1 using natural plant compounds at 33 and 100 µM concentration.
Effect of the natural products on GST kinetics
On the basis of the results for the inhibitory effects of isofuranonaphthoquinone 1, its effects on the kinetics of the GSTs were determined. The trend in changes of KmGSH/CDNB and VmaxGSH/CDNB values with increase in natural product concentration was used to determine the type of inhibition. The predominant type of inhibitions with respect to the G site (GSH) and H site (CDNB) was noncompetitive and mixed type of inhibition. shows the secondary plot for determination of Ki values for 1 on GST P1-1. The data for 1 are summarized in .
Table 2. The effects of 5,8-dihydroxy-1-hydroxymethylnaphtho[2,3-c]furan-4,9-dione (1) on the kinetic properties of GST P1-1 with 1-chloro-2,4 dinitrobenzene as electrophilic substrate.
Discussion
During cancer development, synthesis of the enzyme GST P1-1 is greatly increased so that tumors over express this enzymeCitation45. GST P1-1 detoxifies drugs, particularly anti-cancer drugs, and, therefore, the elevated GST P1-1 levels could greatly inhibit the effectiveness of chemotherapy due to the inactivation of drugs. Therefore, increased levels of GST P1-1 found in tumor cells promote the growth and spread of cancerCitation13. These observations have led for the need of compounds that can inhibit GST P1-1 as this inhibition could be a useful cancer treatment strategy.
The mono- and di-hydroxyxanthones 7 and 9 have almost the same level of potency, with the slight difference in favour of the mono-hydroxy xanthone probably attributed to the preferred point of attachment specified by the only hydroxyl group at the second position. The coumarins 8 and 10 are both pentasubstituted and belong to a small group of unusual coumarins that are also alkylated at position 4. The 5-hydroxygroup is hydrogen-bonded to the carbonyl of the side chain carbonyl in both compounds. Furthermore 8 is substituted with the bulky phenyl group at the fourth position. However, the 7-hydroxy group is relatively less hindered in 8 than in 10. This may explain the greater potency of 8 in inhibiting GST Pi than that of 10. Xanthone 9 appears to be slightly weaker than either of the coumarins at the low concentration of 33 µM, but has a comparable activity to 8 at higher concentration.
The iridoid 3 seemed to inhibit GST P1-1 at low concentration but activate the enzyme at high concentration. These observations indicate that there could be other molecular interactions occurring between 3 and either the substrates or the enzyme. The compounds 1 and 2 were the two inhibitors that were found to be potent since they displayed more than 60% inhibition of the enzyme at the lower concentration of 33 μM.
Isofuranonaphthoquinone 1 displayed potent inhibition properties when using both CDNB and MCB as substrates. However, 2 was not effective as an inhibitor of GSTP1-1 when CDNB was used a substrate although inhibition was observed when MCB was used a substrate. The differences in the results obtained in the interaction of 2 with MCB and CDNB can be explained by the different interactions that some enzymes display with different substrates. For example, in the study of the effect of the antimalarial drugs on GST activity, it was noticed that artemisinin inhibited GSTs when the substrate CDNB was used but when the substrate ethacrynic acid was used instead, the drug showed no inhibitionCitation35. It has also been suggested that GSTs may have two other substrate-binding sites that are distinct from the H siteCitation46. These are the benzyl isothiocyanate (BITC) and monobromobimane (MBB) sites. MBB is an analogue of MCB where the chlorine atom in the latter is replaced by bromine, and it may be reasonable to suppose that both MBB and MCB would likely bind to the same site. The MBB site has been found in pig GST Pi and rat GST Mu classesCitation46. A study on these sites found an MBB derivative to be a competitive inhibitor of rat GST M1-1 and pig GST Pi using MBB as a substrate but not CDNBCitation46. These findings imply that MBB and CDNB have separate binding sites. An earlier study using affinity labelled MBB showed that the MBB- and CDNB-binding sites were independentCitation47. It may, thus, be that human GST P1-1 contains a site similar to the MBB site that can bind MCB, and that 2 is an inhibitor at that MBB site and not the CDNB site.
The IC50 value for 1 of 6.8 µM is only slightly higher than those of other potent GST inhibitors, such as the natural plant phenolic compound curcumin whose IC50 value was found to be 5 µM using GST P1-1 and ellagic acid which has a 1.6 µM IC50 value using GST A2-2Citation44. Cibacron blue, a known GST inhibitor, has an IC50 value of approximately 2.0 µM. A study by Van Haaften et al.Citation43 showed that α-tocopherol can inhibit GST P1-1 activity. α-Tocopherol was found to have an IC50 value 0.5 μM and had a Km and Vmax of 1.11 mM and 18.83 μmol/min/mg protein, respectively, at the H site and 1.0 mM and 18.11 μmol/min/mg protein, respectively, at the G siteCitation48. These findings show that natural products can modify the activity of drug metabolizing enzymes and, thus, have potential use as chemomodulatorsCitation48. Low IC50 values are desirable if inhibition is to occur in cells since very high levels of exogenous compounds can be toxic to a cell because these xenobiotics may interfere with certain biological pathways. Also, these high levels can be difficult to achieve in vivo since cells actively efflux xenobiotics, and so the desired inhibitions might not occurCitation6. However, since 1 is potent even at low concentrations, it may possibly inhibit GSTs in vivo.
Isofuranonaphthoquinone 1 was found to display noncompetitive inhibition at the H site. In this type of inhibition, the inhibitor binds to both the enzyme and the enzyme substrate complex. This type of inhibition cannot be overcome by large amounts of substrateCitation49. However, 1 showed mixed inhibition at the G site. Mixed inhibition is similar to noncompetitive inhibition in that the inhibitor binds to both the free enzyme and the enzyme–ubstrate complex. Mixed inhibition may come up as a result of reversible binding of the inhibitor at a site other than the active site or reversible binding to the enzyme–substrate complexCitation49.
The results indicate that 1 is a more potent inhibitor of the H site than the G site. The structure of GSH and 1 is very different: the former being a linear flexible aliphatic chain which may engage in inter- or intra-molecular attractions due to hydrogen bonds between carbonyl oxygen and the N–H and O–H bonds. On the other hand, isofuranonaphthoquinone is a tricyclic aromatic molecule, which can easily form an alternative quinone–quinol structure through tautomerism. It is, therefore, possible to conclude that 1 and GSH are not likely to bind to the same site. Kcat/Km, the catalytic efficiency of the reaction, would decrease as inhibitor concentration increased due to the reduced activity of the enzyme. This trend was noted for 1 in the inhibition of both the G- and H sites, and these findings were consistent with those found in the literatureCitation35. The Ki value of 1 at the H site was low, and this shows that the inhibitor had a high affinity for the H site. The Ki value is comparable to that of other natural compounds that inhibit GST P1-1. Curcumin has a much higher Ki of 9.6 and ellagic acid has a Ki of 11Citation44. Thus, 1 is more potent as an inhibitor than these compounds.
Flavonoids and isoflavonoids, such as eriodictyol, quercetin, and genistein, found in dietary agents such as soy foods have been found to reduce the risk of cancer through many mechanisms, including the inhibition of drug-metabolizing enzymesCitation45. Other inhibitors of GSTs include other phenolic compounds such as epigallocatechin galateCitation50, ellagic acid, and curcuminCitation44. Ethacrynic acid modulates the cytotoxicity of doxorubicin, an anti-cancer agent, by inhibiting GSTs and reducing efflux of the drug from the cell, thereby, increasing the therapeutic efficiency of the drugCitation51. It is postulated that 1 may inhibit GST P1-1 via two ways. It may react with GSH via its quinone moiety. The conjugate formed then inhibits GSTP1-1 as glutathione analogue. Alternatively 1 may react directly with the protein. The major target in proteins is the thiol group of cysteine residues. GST P1-1 has a cysteine at the active site and this may be susceptible to reaction with the quinone. Both proposed schemes are shown in . Compounds with quinone groups have been shown to react with glutathione in vitroCitation10. The activity of 2 may be different from all the above compounds which contain at least one aromatic ring and phenolic hydroxyl groups. The compound 2 is a sesquiterpene lactone with a characteristic α,β-unsaturated double bond, which may be responsible for the observed high level of GSTP1-1 inhibition. Indeed, van Iersel et al.Citation52 have found that naturally occurring α and β-unsaturated aldehydes and ketones, such as acrolein and cinnamaldehyde, can inhibit GST P1-1.
Figure 5. Reaction of proteins or GSH with quinones. In A, the reaction is with any nucleophile, whilst in B, the reaction is with reduced glutathione (GSH).
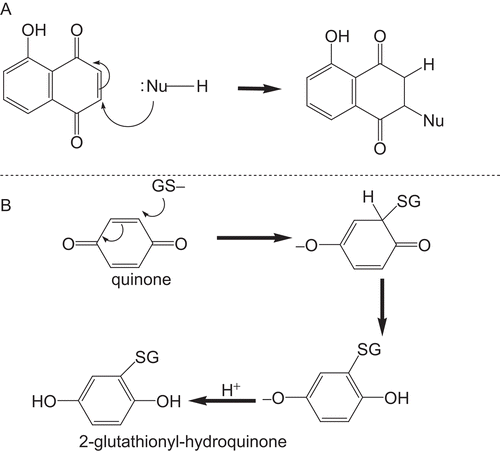
In conclusion, The isofuranonapthoquinone 1 from Bulbine frutescens is a potent inhibitor of human recombinant glutathione transferase P1-1 in vitro using both the fluorescent substrate monochlorobimane and the photometric substrate CDNB. The compound may inhibit GST P1-1 in vivo and could, therefore, be of importance in its potential use as a chemomodulator in situations where GST P1-1 is over expressed and is involved in alkylating anticancer drug resistance. However, care should be taken in interpolating data from in vitro to in vivo situations, as one needs to know about the metabolism of this compound as well as its bioavailability.
Declaration of Interest
The authors report no conflicts of interest. The authors alone are responsible for the content and writing of the paper.
References
- Polasa K, Naidu AN, Ravindranath I, Krishnaswamy K. Inhibition of B(a)P induced strand breaks in presence of curcumin. Mutat Res 2004;557:203–213.
- Cowan MM. Plant products as antimicrobial agents. Clin Microbiol Rev 1999;12:564–582.
- Sevior DK, Hokkanen J, Tolonen A, Abass K, Tursas L, Pelkonen O et al. Rapid screening of commercially available herbal products for the inhibition of major human hepatic cytochrome P450 enzymes using the N-in-one cocktail. Xenobiotica 2010;40:245–254.
- Ghazali R, Waring, RH. Effects of flavonoids on glutathione-S-transferase in human blood platelets, rat liver, rat kidney, and HT-29 colon adenocarcinoma cell-lines: potential in drug metabolism and chemoprevention. Med Sci Res 1999;27:449–451.
- Middleton E Jr, Kandaswami C, Theoharides TC. The effects of plant flavonoids on mammalian cells: implications for inflammation, heart disease and cancer. Pharmacol Rev 2000;52:673–751.
- Gottesman MM, Pastan I. Biochemistry of multidrug resistance mediated by the multidrug transporter. Annu Rev Biochem 1993;62:385–427.
- Volm M. Multidrug resistance and its reversal. Anticancer Res 1998;18:2905–2917.
- Eklund BI, EdalatM Stenberg, G, Mannervik, B. Screening for recombinant glutathione transferases active with monochlorobimane. Analytical Biochem 2002;309:102–108.
- Duvoix A, Morceau F, Delhalle S, Schmitz M, Schnekenburger M, Galteau MM et al. Induction of apoptosis by curcumin: mediation by glutathione S-transferase P1-1 inhibition. Biochem Pharmacol 2003;66:1475–1483.
- Josephy PD, Mannervik B. Molecular Toxicology. Oxford University Press, New York, USA. 2006:372–373.
- Eaton DL, Bammler TK. Concise review of the glutathione S-transferases and their significance to toxicology. Toxicol Sci 1999;49:156–164.
- Townsend DM, Tew KD. The role of glutathione-S-transferase in anti-cancer drug resistance. Oncogene 2003;22:7369–7375.
- McLellan LI, Wolf CR. Glutathione and glutathione-dependent enzymes in cancer drug resistance. Drug Resist Updat 1999;2:153–164.
- Leistner OA. Seed Plants of Southern Africa: Families and Genera, Strelitzia, National Botanical Institute, Pretoria. 2000;10.
- Zdero C, Bohlmann F. Sesquiterpene lactones from Dicoma species. Phytochemistry 1990;29:183–187.
- Neuwinger HD. African traditional medicine: A dictionary of plant use and applications. Medpharm Scientific Publishers, Stuttgart, 2000.
- Komguem J, Meli AL, Manfouo RN, Lontsi D, Ngounou FN, Kuete V et al. Xanthones from Garcinia smeathmannii (Oliver) and their antimicrobial activity. Phytochemistry 2005;66:1713–1717.
- Lannang AM, Komguem J, Ngninzeko FN, Tangmouo JG, Lontsi D, Ajaz A, Choudhary MI, Sondengam BL, Atta-Ur-Rahman. Antioxidant benzophenones and xanthones from the root bark of Garcinia smeathmannii. Bulletin of the Chemical Society of Ethiopia 2006;20:247–252.
- Abegaz BM, Bezabih M, Msuta T, Brun R, Menche D, Mühlbacher J et al. Gaboroquinones A and B and 4′-O-demethylknipholone-4′-O-beta-D-glucopyranoside, phenylanthraquinones from the roots of Bulbine frutescens. j Nat Prod 2002;65:1117–1121.
- Kuete V, Metuno R, Ngameni B, Mbaveng AT, Ngandeu F, Bezabih M, Etoa F-X Ngadjui, BT, Abegaz BM, Beng VP. Antimicrobial activity of the methanolic extracts and compounds from Treculia africana and Treculia acuminata (Moraceae), South African Journal of Botany 2008;74:111–115.
- Kuete V, Ngameni Mbaveng AT, Ambassa P, Simo IK, Bezabih M, Etoa F-X Ngadjui, BT, Abegaz BM, Beng VP. Antimicrobial activity of the extract from the twigs of Dorstenia elliptica (Moraceae). Pharmacologyonline 2007;1:573–580.
- Akhtar N, Malik A. Oleanene type triterpenes from Plumeria rubra. Phytochemistry 1993;32:1523–1525.
- Kazmi SN, Ahmed Z, Ahmed W, Malik A. Plumerinine ― A novel lupin alkaloid from Plumeria rubra. Heterocycles 1989;29:1901–1906.
- Kardono LB, Tsauri S, Padmawinata K, Pezzuto JM, Kinghorn AD. Cytotoxic constituents of the bark of Plumeria rubra collected in Indonesia. J Nat Prod 1990;53:1447–1455.
- Ouahouo BM, Azebaze AG, Meyer M, Bodo B, Fomum ZT, Nkengfack AE. Cytotoxic and antimicrobial coumarins from Mammea africana. Ann Trop Med Parasitol 2004;98:733–739.
- Volm M, Zintl F, Edler L, Sauerbrey A. Prognostic value of protein kinase C, proto-oncogene products and resistance-related proteins in newly diagnosed childhood acute lymphoblastic leukemia. Med Pediatr Oncol 1997;28:117–126.
- Wellems TE. Plasmodium chloroquine resistance and the search for a replacement antimalarial drug. Science 2002;298:124–126.
- Zhao G, Liu C, Wang R, Song D, Wang X, Lou H, Jing Y. The synthesis of unsaturated carbonyl derivatives with the ability to inhibit both glutathione S-transferase P1-1 activity and the proliferation of leukaemia cells. J Bioorg Med Chem 2007;15:2701–2707.
- Demirtas I, Sahin A, Ayhan B, Tekin S, Telc I. Antiproliferative Effects of the Methanolic Extracts of Sideritis libanotica Labill. subsp. Linearis. Rec. Nat. Prod 2009;3:104–109.
- Rosario LA, O’Brien ML, Henderson CJ, Wolf CR, Tew KD. Cellular response to a glutathione S-transferase P1-1 activated prodrug. Mol Pharmacol 2000;58:167–174.
- Tew KD, Dutta S, Schultz M. Inhibitors of glutathione S-transferases as therapeutic agents. Adv Drug Deliv Rev 1997;26:91–104.
- Bezabih M, Abegaz BM, Dufall K, Croft K, Skinner-Adams T, Davis TM. Antiplasmodial and antioxidant isofuranonaphthoquinones from the roots of Bulbine capitata. Planta Med 2001;67:340–344.
- Zdero C, Bohlmann F. Sesquiterpene lactones from Dicoma species. Phytochemistry 1990;29:183–187.
- Abegaz BM, Ngadjui BT, Folefoc GN, Fotso S, Ambassa P, Bezabih M et al. Prenylated flavonoids, monoterpenoid furanocoumarins and other constituents from the twigs of Dorstenia elliptica (Moraceae). Phytochemistry 2004;65:221–226.
- Mukanganyama S, Widersten M, Naik YS, Mannervik B, Hasler JA. Inhibition of glutathione S-transferases by antimalarial drugs possible implications for circumventing anticancer drug resistance. Int J Cancer 2002;97:700–705.
- Magadula JJ, Kapinug M, Bezabih M, Abegaz BM. Polyisoprenylated benzophenones from Garcinia semseii (Clusiaceae). Phytochemistry letters 2008;1:215–218.
- Methuno R, Ngandeu F, Tchinda AT, Ngameni B, Kapche GDWF, Djemgou PC, Ngadjui BT, Bezabih M, Abegaz BM. Chemical constituents of Treculia acuminata and Treculia Africana (Moraceae). Biochem Syst Ecol 2008;36:148–152.
- Gottleib OR, Mesquita AAL, de Oliveria GG, de Melo MT. Xanthones from Kielmeyera speciosa. Phytochemistry 1970;9:2537–2544.
- Crichton EG, Waterman PG. Dihydromammea C/OB: A new coumarin from the seed of Mammea Africana, Phytochemistry 1978;17:1783–1785.
- Yang XD, Xu LZ, Yang SL. Xanthones from the stems of Securidaca inappendiculata. Phytochemistry 2001;58:1245–1249.
- Habig WH, Pabst MJ, Jakoby WB. Glutathione S-transferases. The first enzymatic step in mercapturic acid formation. J Biol Chem 1974;249:7130–7139.
- Lowry OH, Rosebrough NJ, Farr AL, Randall RJ. Protein measurement with the Folin phenol reagent. J Biol Chem 1951;193:265–275.
- Segel, IH. Enzyme Kinetics. John Wiley and Sons: Toronto, USA. 1975.
- Hayeshi R, Mutingwende I, Mavengere W, Masiyanise V, Mukanganyama S. The inhibition of human glutathione S-transferases activity by plant polyphenolic compounds ellagic acid and curcumin. Food Chem Toxicol 2007;45:286–295.
- Birt DF, Hendrich S, Wang W. Dietary agents in cancer prevention: flavonoids and isoflavonoids. Pharmacol Ther 2001;90:157–177.
- Hearne JL, Colman RF. Delineation of xenobiotic substrate sites in rat glutathione S-transferase M1-1. Protein Sci 2005;14:2526–2536.
- Hu L, Borleske BL, Colman RF. Probing the active site of alpha-class rat liver glutathione S-transferases using affinity labeling by monobromobimane. Protein Sci 1997;6:43–52.
- van Haaften RI, Haenen GR, van Bladeren PJ, Bogaards JJ, Evelo CT, Bast A. Inhibition of various glutathione S-transferase isoenzymes by RRR-alpha-tocopherol. Toxicol in Vitro 2003;17:245–251.
- Kuby KA. A study of enzymes Volume 1: Enzyme catalysis, kinetics and substrate binding. CRC Press, Inc. 2000 Corporate Blvd, Boca Raton, Florida 33431, USA.
- Koo JY, Kim HJ, Jung KO, Park KY. Curcumin inhibits the growth of AGS human gastric carcinoma cells in vitro and shows synergism with 5-fluorouracil. J Med Food 2004;7:117–121.
- Awasthi S, Singhal SS, He N, Chaubey M, Zimniak P, Srivastava SK et al. Modulation of doxorubicin cytotoxicity by ethacrynic acid. Int J Cancer 1996;68:333–339.
- van Iersel ML, Ploemen JP, Lo Bello M, Federici G, van Bladeren PJ. Interactions of alpha, beta-unsaturated aldehydes and ketones with human glutathione S-transferase P1-1. Chem Biol Interact 1997;108:67–78.