Abstract
A carbonic anhydrase (CA, EC 4.2.1.1) from red blood cells of pigeons (Columba livia var. domestica), clCA, was purified to homogeneity. Its kinetic parameters for the CO2 hydration reaction were measured. With a kcat/Km of 1.1 × 108 M−1 s−1, and a kcat of 1.3 × 106 s−1, clCA has a high activity, similar to that of the human isoform hCA II. A group of 25 aromatic/heterocyclic sulfonamides incorporating the sulfanilamide, homosulfanilamide, benzene-1,3-disulfonamide, and acetazolamide scaffolds showed variable inhibitory activity against the pigeon enzyme, with KIs in the range of 1.9–3460 nM. Red blood cells of pigeons, like those of ostriches, contain thus just one CA isoform, unlike the blood of mammals, which normally contain two isoforms, one of low (CA I-like) and one of very high activity (CA II-like). However, from the sulfonamide inhibition viewpoint, the pigeon enzyme was more similar to hCA II than to the ostrich enzyme.
Introduction
The metalloenzyme carbonic anhydrase (CA, EC 4.2.1.1) is involved in pH buffering of extra and intracellular spaces by catalyzing the interconversion of CO2 to HCO3− with generation of protonsCitation1–4. The equilibration between these chemical species is constantly maintained by the catalytic activity of these enzymes, across the whole phylogenetic tree, in prokaryotes and eukaryotesCitation5–9. CAs are in fact encoded by five distinct, evolutionarily unrelated gene families: the α-, β-, γ-, δ- and ζ-CAsCitation1,Citation5–9. The α-CA gene family is present in many organisms, starting with bacteria and plants, protozoa, or invertebrates and ending with higher vertebrates, including humansCitation1–4. The ubiquity of these enzymes in all these organisms is clearly due to their involvement in basic physiological processes, in which the three chemical species mentioned above (CO2, HCO3−, and the H+ ions) are involvedCitation1–4.
It is thus not surprising that in mammals 16 different α-CA isozymes have been described, with very different catalytic activity, subcellular localization, tissue distribution, physiological/pathological roles, and susceptibility to inhibitorsCitation1–4,Citation8,Citation9. Among them, are five cytosolic catalytically-active forms (CA I–III, CA VII, and CA XIII), five membrane-bound isozymes (CA IV, CA IX, CA XII, CA XIV, and CA XV), two mitochondrial ones (CA VA and CA VB), a secreted CA isozyme, CA VI (in the saliva and milk), as well as three acatalytic proteins, CA VIII, X and XI, and CA-related proteinsCitation1–4. CA XV is not present in primates but is found in other vertebrates, such as rodents, birds, and fishCitation9. These different isoforms are involved in physiological processes connected with the respiration and transport of CO2/HCO3− between metabolizing tissues and lungs, pH and CO2 homeostasis, electrolyte secretion in a variety of tissues/organs, biosynthetic reactions (e.g., gluconeogenesis, lipogenesis, and ureagenesis), bone resorption, calcification, tumourigenicity, epileptogenesis, tumourigenesis, etcCitation1–9.
Vertebrate genomes encode only for α-CAs (which are zinc enzymes), but generally a rather high (and variable) number of isoforms is present in these organisms, and their precise number is not known for most ordersCitation1–4,Citation8. With the exception of humans and rodents (for which 15 and respectively 16 CAs have been described and characterized in detail, as outlined above)Citation1–4, few other vertebrates have been thoroughly investigated regarding the number of isoforms, their catalytic activity, and inhibition susceptibility with various classes of inhibitors Citation1,Citation10,Citation11. Indeed, some literature data are available for several fish speciesCitation10 (such as the model animal zebra fish, Danio rerioCitation11, the rainbow trout Oncorhyncus mykissCitation12, the sea bass Dicentrarchus labraxCitation13 or the haemoglobinless Antarctic icefish Chionodraco hamatusCitation14). Among birds, the red blood cell enzyme from the ratid Struthio camelus (ostrich)Citation15 has been investigated in some detail, but as outlined above, these data for enzymes which are of non-human or non-rodent origin, are rather scarce. In the case of birds (Aves), there are several reports on CAs from chicken (Gallus gallus) and ostrich (S. camelus)Citation16–20 but no attempts were made to isolate, characterize, and study the inhibition of these enzymes were done, except for the ostrich enzyme which has been investigated in detail in this studyCitation15. It is interesting to note that ThomasCitation17 reported previously in a preliminary work about some CA activity of pigeon erythrocyte.
In a previous work, we have reported on the kinetic parameters for the CO2 hydrase activity of the red blood cell CA from S. camelus (scCA) and its inhibition with a series of aromatic and heterocyclic sulfonamides, one of the main class of CA inhibitors (CAIs)Citation15. Here, we extend this type of investigation to another enzyme of the avian origin, and report on the purification, characterization, and inhibition susceptibility to sulfonamides of a CA isolated from red blood cells of the pigeon, Columba livia var. domestica.
Materials and methods
Sulfonamide inhibitors
Acetazolamide (AZA), ethoxzolamide (EZA), and sulfanilamide (SA) were from Sigma–Aldrich (Milan, Italy). Compounds 1–5 used in the assay were previously reported by one of our groupsCitation21–27.
Preparation of the pigeon blood haemolysate
Blood samples from C. livia erythrocytes were anticoagulated with acid-citrate-dextrose and centrifuged at 1848g for 20 min at 4°C. The supernatant was removed. The packed red cells were washed with NaCl (0.9%) three times and the erythrocytes were haemolysed with three times the volume of cold water for 1 h. The ghost and intact cells were removed by centrifugation at 18,924g for 25 min at 4°C, and the pH of the haemolysate was adjusted to 8.5 with solid Tris buffer. The haemolysate was applied to an affinity column containing sepharose-4B-l-tyrosine-sulfonamide and equilibrated with 25 mM Tris–HCl/0.1 M Na2SO4 (pH 8.5). The affinity gel was washed with a solution of 25 mM Tris–HCl/22 mM Na2SO4 (pH 8.5). The CA was eluted with a solution of 0.1 M CH3COONa/0.5 M NaClO4 (pH 5.6). The enzyme was dialyzed extensively for 3 days against 20 mM Tris buffer (pH 7.5) until all the acetate and perchlorate were removedCitation15.
Determination of protein content
After scanning at 280 nm, the tubes with the significant absorbance were pooled and a quantitative protein determination was done by the Coomassie brilliant blue G-250 methodCitation28.
CA assay
The change in absorbance of a pH indicator was measured by an SX.18MV-R (Applied Photophysics, Leatherhead, Surrey, UK) stopped-flow instrument in order to determine the initial velocities of the CO2 hydration reaction which is catalysed by different CA isozymes, including the newly purified clCACitation29. Phenol red (at a concentration of 0.2 mM) was used as an indicator, working at the absorbance maximum of 557 nm, with 10 mM HEPES( 4-(2-hydroxyethyl)-1-piperazineethanesulfonic acid; pH 7.5) as buffer, 0.1 M Na2SO4 (for maintaining constant the ionic strength), following the CA-catalyzed CO2 hydration reaction for a period of 10–100 s. Saturated CO2 solutions in water at 20°C were used as substrate. The CO2 concentrations ranged from 1.7–17 mM for the determination of the catalytic and inhibition constants. For each inhibitor, at least six traces of the initial 5–10% of the reaction have been used for determining the initial velocity. The uncatalyzed rates were determined in the same manner and subtracted from the total observed rates. Stock solutions of inhibitors were prepared at a concentration of 1–3 mM (in DMSO-water 1:1, v/v) and dilutions up to 0.01 nM were done with the assay buffer mentioned above. The kinetic kcat and kcat/Km were obtained by non-least square methods using PRISM3 (La Jolla, CA) whereas the inhibition constants were obtained by non-linear least-squares methods using PRISM 3, from Lineweaver-Burk plots, as reported earlierCitation15, and represent the mean from at least three different determinations.
Results and discussion
Among the higher vertebrate CAs, the human and mouse isozymes have generally been thoroughly characterized both with regard to their kinetic properties for the CO2 hydration reaction, as well as for their interaction with sulfonamide inhibitorsCitation1–9. However, few isozymes of other origin have been investigated in detail. This is particularly true for birds (Aves), for which different reports mention the role of CAs in the eggshell formation for chicken (Gallus gallus) and ostrich (S. camelus), but few attempts have been undertaken to isolate, characterize, and investigate the inhibition of these enzymes were doneCitation15–20. Here, we report on the purification of a CA from pigeon red blood cells as well as on the first inhibition study of this enzyme with a series of aromatic and heterocylic sulfonamides. This study is of interest in order to understand potential differences between diverse CAs from higher vertebrates such as mammals and birds, which diverged evolutionarily more than 350 million years ago.
As the sulfonamides represent a major class of CAIs, we also investigated the interaction of clCA with three sulfonamide drugs known to possess relevant CA inhibitory propertiesCitation1–3, AZA, EZA, and SA, as well as with a library of sulfonamides of types 1–5, reported on earlier by one of our groupsCitation21–27.
Blood from domestic pigeons has been used for the isolation and purification of the enzyme, as described in detail in the Materials and Methods section. Only one CA isoform, with a molecular weight of around 30 kDa (the same as those of the human (h) CA isoforms hCA I and II1–3) was isolated and purified from pigeon blood by sulfonamide affinity chromatography (). The catalytic activity of this preparation was measured by using a stopped-flow spectrophotometric methodCitation29, monitoring the physiologic reaction catalyzed by these enzymes, i.e., CO2 hydration to HCO3− and protons. The data in show the kinetic parameters (kcat and Km) for the physiologic reaction catalyzed by various vertebrate CA isoforms, such as the primate ones hCA I-hCA III, and the bird enzymes from ostrich (scCA)Citation15 and pigeon, denominated here clCA (see Experiment for details).
Table 1. Kinetic parameters for CO2 hydration reaction catalysed by the cytosolic α-CA isozymes of mammalian and avian, origin and their susceptibility to acetazolamide (AZA) inhibition.
Figure 1. SDS PAGE (sodium dodecyl sulfate polyacrylamide gel electrophoresis) for clCA purified from red blood cells (lane 4) compared to ladder (lane 1). hCA I (lane 2) and hCA II (lane 3). The hCAs were from Sigma–Aldrich.
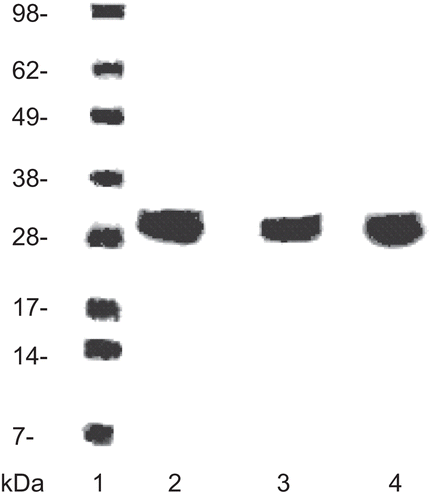
Data of show the pigeon enzyme clCA to possess a very high catalytic activity as CO2 hydrase, with a kcat/Km of 1.1 × 108 M−1 s−1, similar to that of the most active human isoform hCA II (the physiologically dominant one)Citation1–4), which is also one of the most effective catalysts known in nature(with a kcat/Km of 1.5 × 108 M−1 s−1)Citation1,Citation10. The pigeon enzyme has a first order rate constant of 1.3 × 106 s−1, slightly lower than hCA II (kcat of 1.4 × 106 s−1), whereas the Michaelis–Menten constants (Km) of the two enzymes are also rather similar, of 11.8 mM for the pigeon enzyme and 9.3 mM for hCA II. It should be observed that clCA is catalytically much more active than other vertebrate enzymes investigated earlier, such as the human ones hCA I, III, and IV or the ratid one scCA, which showed turnover numbers in the range of 2.5 × 105–5.1 × 107 M−1 s−1 (). The pigeon enzyme, similar to all other CAs explored to date with the exception of CA IIICitation30, was also significantly inhibited by the clinically used sulfonamide CAI AZA (1,3,4-thiadiazole-2-sulfonamide) with an inhibition constant of 71 nM ().
Sulfonamides constitute one the most important class of CAIs, with many representatives used as human or veterinary drugs, as diuretics, antiglaucoma, antiobesity or antiepileptic agentsCitation1–3. It appeared thus of interest to investigate inhibition of the pigeon enzyme isolated here, clCA, with some of these compounds, many of which are widely used drugsCitation1–3. We have included in this study, three clinically used derivatives, AZA, EZA, and SA, as well as a group of 22 other derivativesCitation21–27, of types 1–5, some of which are aromatic (1–3) and heterocyclic (4 and 5) sulfonamides, obtained by the tail approachCitation31,Citation32, considering SA and AZA as lead molecules. These compounds have been investigated earlier as inhibitors of the human isoforms hCA I and IICitation21–27 and more recently for their interaction with the ostrich enzyme scCACitation15. In , the inhibition data of three vertebrate CAs with these 25 sulfonamides are shown: hCA II and ostrich enzyme scCA inhibition data are shown for comparison reasons, together with the new data of the pigeon enzyme isolated here for the first time.
Table 2. hCA II, scCA, and clCA inhibition data with sulfonamides 1–5, acetazolamide (AZA), ethoxzolamide (EZA) and sulfanilamide (SA). Data of hCA II and scCA are from refs.Citation15,Citation21–27.
Several interesting structure-activity relationship features were observed for the inhibition of clCA with the set of compounds investigated here: (i) SA and two other derivatives, the thioureas 1n and 1p, showed weak inhibitory action against clCA, with inhibition constants of 621–3460 nM. It is interesting to note that the two thioureas incorporate the same scaffold but have one or respectively two CH2 moieties between the benzenesulfonamide and phenylthiourea fragments of the molecule. These two compounds also act as very effective, low nanomolar hCA II inhibitors (KIs of 12–53 nM), whereas they are weak inhibitors for the ostrich enzyme (KIs of 2970–2980 nM). Thus, the two avian enzymes are more similar with each other with regard to their interaction with these two inhibitors. However, SA acts as a much more potent scCA inhibitor (KI of 570 nM) compared to its inhibition of the pigeon enzyme; (ii) A rather large group of derivatives, i.e., 1a–1g, 1i, 1q and 2, showed medium inhibitory action against the pigeon enzyme, with KIs in the range of 137–467 nM. They include, the acylated SAs 1a–1g incorporating aliphatic moieties, the disulfonamide 1i, as well as the ureas 1q and 2. Thus, the introduction of acyl or alkylsulfonyl moieties in the SA scaffold, dramatically enhances the clCA inhibitory activity with these compounds, compared to the lead. Furthermore, an increase in the alkyl chain in compounds 1a–1g from one to four carbon atoms, leads to an increase of the inhibitory activity against the pigeon enzyme. It should be noted that most of these compounds are rather weak ostrich CAIs, whereas their affinity for the human enzyme hCA II is better, in the same range as for the pigeon one (). (iii) Potent clCA inhibition, with KIs in the range of 1.9–95 nM, has been observed with the following compounds investigated here: 1h, 1j–1l, 1m, 1r, 1s, 3, 4, 5, AZA, and EZA. They include the benzoylated SA 1h, as well as the structurally related sulfonylated SAs 1i–1m, the bis-sulfonamides 1r and 1s, the urea incorporating the benzene-1,3-disulfonamide scaffold 3, the bromo-nitro-substituted benzolamides 4a and 4b, the furanyl analogue of methazolamide 5 as well as AZA and EZA. Several inhibitors with KIs < 5 nM for the pigeon enzyme have been identified, such as 4a, 4b, and 5. It should be mentioned that most of these compounds are much weaker inhibitors for the ostrich enzyme, whereas their affinity for hCA II is most of the time in the low nanomolar range.
In conclusion, we report here that the red blood cells of the pigeon contain just one CA isoform, unlike the blood of mammals, which usually contain two such isoforms, one of low activity (CA I-like) and one of high or very high activity (CA II-like). Pigeons, similar to ostriches, have thus a high activity, CA II-like isoform in the blood. clCA purified here showed kinetic parameters for the CO2 hydration reaction typical of a highly effective catalyst, with a kcat/Km of 1.1 × 108 M−1 s−1, and a kcat of 1.3 × 106 s−1. A group of 25 aromatic/heterocyclic sulfonamides incorporating the SA, homosulfanilamide, benzene-1,3-disulfonamide, and AZA scaffolds, showed variable inhibitory activity against the pigeon enzyme, with KIs in the range of 1.9–3460 nM. The least effective inhibitor was SA, whereas the highly effective ones incorporated benzolamide- and AZA-like scaffolds. The pigeon enzyme showed inhibitory properties more similar to the human than the ostrich enzyme for this class of sulfonamides.
Declaration of interest
During the course of this study, the Scientific and Technical Research Council of Turkey (TUBITAK) provided a scholarship (NATO-A2) to Ozen Ozensoy, which is gratefully acknowledged. The authors also thank to Balikesir University, Research Center of Applied Sciences (BURCAS/Balikesir, Turkey) for providing research facilities and an EU 7th FP research grant to CTDS (Metoxia project).
References
- Pastorekova S, Parkkila S, Pastorek J, Supuran CT. Carbonic anhydrases: current state of the art, therapeutic applications and future prospects. J Enzyme Inhib Med Chem 2004;19:199–229.
- Supuran CT, Scozzafava A, Casini A. Carbonic anhydrase inhibitors. Med Res Rev 2003;23:146–189.
- Supuran CT. Carbonic anhydrases: novel therapeutic applications for inhibitors and activators. Nat Rev Drug Discov 2008;7:168–181.
- Supuran CT. Carbonic anhydrase inhibition/activation: trip of a scientist around the world in the search of novel chemotypes and drug targets. Curr Pharm Des 2010;16:3233–3245.
- Ferry JG. The gamma class of carbonic anhydrases. Biochim Biophys Acta 2010;1804:374–381.
- Supuran CT. Carbonic anhydrase inhibitors. Bioorg Med Chem Lett 2010;20:3467–3474.
- Hall RA, Mühlschlegel FA. Fungal and nematode carbonic anhydrases: their inhibition in drug design. In: Supuran CT, Winum JY, eds. Drug Design of Zinc-Enzyme Inhibitors: Functional, Structural, and Disease Applications. Hoboken: John Wiley & Sons 2009, 301–322.
- Swietach P, Wigfield S, Cobden P, Supuran CT, Harris AL, Vaughan-Jones RD. Tumor-associated carbonic anhydrase 9 spatially coordinates intracellular pH in three-dimensional multicellular growths. J Biol Chem 2008;283:20473–20483.
- Hilvo M, Tolvanen M, Clark A, Shen B, Shah GN, Waheed A et al. Characterization of CA XV, a new GPI-anchored form of carbonic anhydrase. Biochem J 2005;392:83–92.
- Gilmour KM. Perspectives on carbonic anhydrase. Comp Biochem Physiol, Part a Mol Integr Physiol 2010;157:193–197.
- Lin TY, Liao BK, Horng JL, Yan JJ, Hsiao CD, Hwang PP. Carbonic anhydrase 2-like a and 15a are involved in acid-base regulation and Na+ uptake in zebrafish H+-ATPase-rich cells. Am J Physiol, Cell Physiol 2008;294:C1250–C1260.
- Hisar O, Beydemir S, Gülçin I, Küfrevioglu OI, Supuran CT. Effects of low molecular weight plasma inhibitors of rainbow trout (Oncorhynchus mykiss) on human erythrocyte carbonic anhydrase-II isozyme activity in vitro and rat erythrocytes in vivo. J Enzyme Inhib Med Chem 2005;20:35–39.
- Ekinci D, Ceyhun SB, Sentürk M, Erdem D, Küfrevioglu OI, Supuran CT. Characterization and anions inhibition studies of an a-carbonic anhydrase from the teleost fish Dicentrarchus labrax. Bioorg Med Chem 2011;19:744–748.
- Rizzello A, Ciardiello MA, Acierno R, Carratore V, Verri T, di Prisco G et al. Biochemical characterization of a S-glutathionylated carbonic anhydrase isolated from gills of the Antarctic icefish Chionodraco hamatus. Protein J 2007;26:335–348.
- Ozensoy O, Isik S, Arslan O, Arslan M, Scozzafava A, Supuran CT. Carbonic anhydrase inhibitors. Inhibition of red blood cell ostrich (Struthio camelus) carbonic anhydrase with a series of aromatic and heterocyclic sulfonamides. J Enzyme Inhib Med Chem 2005;20:383–387.
- Palatroni P, Gabrielli MG, Grappasonni I. Comparative study on carbonic anhydrase activity in the retina of different birds during development. Anat Anz 1987;163:5–18.
- Thomas PC. Carbonic anhydrase activity of pigeon erythrocytes. Indian J Exp Biol 1970;8:338–339.
- Quélo I, Machuca I, Jurdic P. Identification of a vitamin D response element in the proximal promoter of the chicken carbonic anhydrase II gene. J Biol Chem 1998;273:10638–10646.
- Gabrielli MG. Carbonic anhydrases in chick extra-embryonic structures: a role for CA in bicarbonate reabsorption through the chorioallantoic membrane. J Enzyme Inhib Med Chem 2004;19:283–286.
- Berg C, Blomqvist A, Holm L, Brandt I, Brunström B, Ridderstråle Y. Embryonic exposure to oestrogen causes eggshell thinning and altered shell gland carbonic anhydrase expression in the domestic hen. Reproduction 2004;128:455–461.
- Vullo D, Franchi M, Gallori E, Antel J, Scozzafava A, Supuran CT. Carbonic anhydrase inhibitors. Inhibition of mitochondrial isozyme V with aromatic and heterocyclic sulfonamides. J Med Chem 2004;47:1272–1279.
- Clare BW, Supuran CT. Carbonic anhydrase inhibitors. Part 61. Quantum chemical QSAR of a group of benzenedisulfonamides. Eur J Med Chem 1999;34:463–474.
- Supuran CT, Scozzafava A, Jurca BC, Ilies MA. Carbonic anhydrase inhibitors. Part 49. Synthesis of substituted ureido- and thioureido derivatives of aromatic/heterocyclic sulfonamides with increased affinities for isozyme I. Eur J Med Chem 1998;33:83–93.
- Casini A, Scozzafava A, Mincione F, Menabuoni L, Supuran CT. Carbonic anhydrase inhibitors: synthesis of water soluble sulfonamides incorporating a 4-sulfamoylphenylmethylthiourea scaffold. J Enz Inhib Med Chem 2002;17:333–343.
- Supuran CT, Ilies MA, Scozzafava A. Carbonic anhydrase inhibitors. Part 29. Interaction of isozymes I, II and IV with benzolamide-like derivatives. Eur J Med Chem 1998;33:739–752.
- Ilies M, Supuran CT, Scozzafava A, Casini A, Mincione F, Menabuoni L et al. Carbonic anhydrase inhibitors: sulfonamides incorporating furan-, thiophene- and pyrrole-carboxamido groups possess strong topical intraocular pressure lowering properties as aqueous suspensions. Bioorg Med Chem 2000;8:2145–2155.
- Mincione F, Starnotti M, Menabuoni L, Scozzafava A, Casini A, Supuran CT. Carbonic anhydrase inhibitors: 4-sulfamoyl-benzenecarboxamides and 4-chloro-3-sulfamoyl-benzenecarboxamides with strong topical antiglaucoma properties. Bioorg Med Chem Lett 2001;11:1787–1791.
- Cakir U, Ugras HI, Ozensoy O, Sinan S, Arslan O. In vitro inhibition effects of some new sulfonamide inhibitors on human carbonic anhydrase I and II. J Enzyme Inhib Med Chem 2004;19:257–261.
- Khalifah RG. The carbon dioxide hydration activity of carbonic anhydrase. I. Stop-flow kinetic studies on the native human isoenzymes B and C. J Biol Chem 1971;246:2561–2573.
- Nishimori I, Minakuchi T, Onishi S, Vullo D, Cecchi A, Scozzafava A et al. Carbonic anhydrase inhibitors: cloning, characterization, and inhibition studies of the cytosolic isozyme III with sulfonamides. Bioorg Med Chem 2007;15:7229–7236.
- Menabuoni L, Scozzafava A, Mincione F, Briganti F, Mincione G, Supuran CT. Carbonic anhydrase inhibitors. Water-soluble, topically effective intraocular pressure lowering agents derived from isonicotinic acid and aromatic/heterocyclic sulfonamides: is the tail more important than the ring? J Enzyme Inhib 1999;14:457–474.
- Scozzafava A, Menabuoni L, Mincione F, Briganti F, Mincione G, Supuran CT. Carbonic anhydrase inhibitors. Synthesis of water-soluble, topically effective, intraocular pressure-lowering aromatic/heterocyclic sulfonamides containing cationic or anionic moieties: is the tail more important than the ring? J Med Chem 1999;42:2641–2650.