Abstract
A novel method has been developed for the synthesis of α-oxycarbanilino phosphonates through a reaction of α-hydroxyphosphonates with isocyanate under microwave irradiation. The synthesized compounds were evaluated for their acetylcholinesterase (AChE) inhibition potency through IC50determination. Molecular modelling studies suggest that the most potent inhibitor (compound 4h, IC50 = 6.36 µM) is bound to the peripheral site of AChE, which suggests that it decreases the catalytic activity not through binding to the active site but through blocking the entrance of the active site gorge. This puts forward the potential of compound 4h and its derivatives to be used in the design of dual inhibitors: inhibition of the catalytic activity of AChE and of amyloid β aggregation.
Introduction
Phosphonic acids are of growing importance in understanding and modulating biological processesCitation1–3. In recent years, the synthesis of α-substituted phosphoryl derivatives (phosphonic and phosphinic acids) have attracted significant attentions, due to their biological activities with broad application as enzyme inhibitors, antimetabolites and antibioticsCitation2. Among α-functionalized phosphonic acids, α-hydroxyphosphonic derivatives have potential biological activities such as anti-bacterialCitation4, herbicidalCitation5 and fungicidalCitation6.
Acetylcholinesterase (AChE) hydrolyzes the neurotransmitter acetylcholine (ACh) and is the most widely used target enzyme in studies with the purpose to synthesize new and more effective therapeutic agents to treat patients with diseases such as Alzheimer’s disease (AD) or myasthenia gravisCitation7–Citation9. Reversible inhibition of brain AChE by anti-AD drugs such as tacrineCitation10, donepezilCitation11, and huperzineCitation12 increases the ACh levels and improves neurotransmission in cholinergic synapsesCitation13. AChE is also used widely as a target in the studies of compounds with insecticidal activities, as all commercially available organophosphate compounds are believed to exert their effects through potent reversible or irreversible inhibition of this enzymeCitation14. There are two major binding sites in AChE, a narrow active site gorge, at the base of which is located the catalytic triad or acylation site (A-site), consisting of H447, E334, and S203 (Electrophorus electricus AChE numbering). The other binding site is peripheral anionic site (PAS) which spans from residue W286, at the mouth of the gorge, to D74, at the boundary between PAS and A-SiteCitation15Citation,16. Inhibitors of AChE bind to A-site (organophosphatesCitation14, rivastigmineCitation17, tacrineCitation10) or PAS (propidiumCitation18) or to both of them (donepezilCitation11) in AChE.
The carbamates have attracted lots of attention due to applications such as elastase and cholinesterase inhibitionCitation19. They are derived from carbamic acid and are insecticidal in a similar fashion as organophosphates and are widely used in homes, gardens and agriculture. They inhibit cholinesterase enzymes (acetyl- and butyrylcholinesterases), and terminate nerve impulse transmission. Due to relatively low mammalian oral and dermal toxicity, they have found wide application in lawn and garden settings.
Besides the above applications, recent studies have shown that a number of organophosphates could also be useful as potential chemotherapeutic agentsCitation20,Citation21 and inhibitors of different enzymesCitation22. Although, because of these applications and potential usages, extensive studies on the synthesis of 1-hydroxyphosphonates and carbamate derivatives have been undertaken, relatively few papers have been published on the synthesis of the compounds containing two functionalities in the same molecule, i.e. organophosphates and carbamatesCitation23. The conjunction of two functionalities would presumably afford more versatile biological activity and specifically, less powerful inhibition of AChE, which would make them beneficial in designing new drugs to be used in the treatment of diseases such as AD or myasthenia gravis ().
Despite wide range of biological activities of 1-hydroxyphosphonates and carbamate derivatives, the synthesis and study of anticholinesterase activities of novel α-oxycarbanilinophosphonates have received little attention. To the best of our knowledge, only one synthetic route to α-oxycarbanilinophosphonates has been reported. The method involves prolonged heating (3 days) of α-hydroxyphosphonates with phenylisocyanate at 50°C in the presence of tin octanoate as a catalystCitation23. However, the method has problems including harsh reaction conditions, low yields, long reaction times, using of Lewis acid and side reactions. On the other hand without using tin octanoate as catalyst, N-phenyl isocyanurates has been obtained as the major product. Recently we have reported a novel method using ultrasound energy for the one-pot synthesis of α-oxycarbanilinophosphonatesCitation2Citation4.
The application of microwave energy to accelerate organic reactions is of increasing interest and offers several advantages over conventional techniquesCitation25. Syntheses that normally require lengthy periods can be achieved conveniently and very rapidly in a microwave oven. As part of our efforts to explore the microwave-assisted methodology for the synthesis of organic compoundsCitation26–28, hereby we report a new method for the synthesis of α-oxycarbanilinophosphonates from the reaction of 1-hydroxyphosphonate with isocyanate under microwave irradiation, producing good to high yields of α-oxycarbanilinophosphonates.
In the continuation of our studies on the synthesis and biological activities of novel organophosphatesCitation29,Citation30, we decided to investigate to what extent the inhibitory effect of the synthesized α-oxycarbanilinophosphonates toward electric eel AChE would be affected upon the inclusion of different substitutions in the R and R’ groups. We have shown, through molecular modelling, the most potent synthesized α-oxycarbanilinophosphonates inhibits AChE through binding to the peripheral site and not to the catalytic site of the enzyme.
Materials and methods
General
Chemicals were either prepared in our laboratories or purchased from Merck, Fluka, and Aldrich Chemical Companies. All yields refer to isolated products. NMR spectra were taken by a 250 Brucker with the chemical shifts being reported as δ ppm and couplings expressed in Hertz. Silica gel column chromatography was carried out with Silica gel 100 (Merck No. 10184). UV spectra were determined on a Varian Cary 100 instrument. A microwave reactor (Milestone, Micro SYNTH Microwave Labstation for Synthesis) was used for all experiments with a continuous power source (1000 W max).
General procedure for the synthesis of diethyl (2-4-dichlorophenyl) hydroxymethylphosphonate (2h)
This compound was obtained according to the method of our previously published articleCitation31. Magnesium oxide (2 g) was added to a stirred mixture of diethyl phosphite (20 mol) and 2,4-dichlhorobenzaldehyde (20 mol) at room temperature. After 2 h, the mixture was washed with dichloromethane (4 × 50 mL), dried with CaCl2, and solvent evaporated to give crude product. Product was crystallized from EtOAc/n-hexane.
mp 92–94°C (n-hexane-EtOAc) (Lit. report: 90–91°C)Citation31. 1H NMR (CDCl3, 250 MHz): δ = 1.16 (3H, t, J = 7.0 Hz), 1.36 (3H, t, J = 7.0 Hz), 3.90–4.28 (5H, m), 5.83 (1H, d, JHP = 19.5 Hz), 7.15-7.34 (3H, m); 13C NMR (CDCl3, 62.9 MHz): δ = 16.2, 16.4, 63.1 (d, JPC = 6.9 Hz), 63.2 (d, JPC = 6.9 Hz), 68.8 (d, JPC = 158.1), 128.5, 129.6, 129.7, 132.32; 31P NMR (CDCl3/H3PO4, 101 MHz): δ = 20.80.
General procedure for the synthesis of diethyl[(3-chloro-4-methylanilinocarbonyl)oxy](2,4-dichloro phenyl) methyl phosphonate (4h)
Diethyl (2-4-dichlorophenyl) hydroxymethylphosphonate 2h (2 mmol) was added to dry toluene (8 mL) under argon. 3-Chloro-4-methylisocyanate (3 mmol) was added stepwise for 45 min to the reaction mixture at 50°C at ambient pressure in a microwave reactor. The reaction mixture was stirred for 45 min under microwave irradiation. The solvent was evaporated and the crude product was chromatographed on silica gel (n-hexane:EtOAc = 40/60) to give the pure product in 80% isolated yield.
mp 146–148°C (n-hexane-EtOAc). 1H NMR (CD3SOCD3, 250 MHz): δ = 1.09–1.25 (6H, m), 2.21 (3H, S), 3.96-4.15 (4H, m), 6.67 (1H, d, JHP = 18.8 Hz), 7.20–7.56 (6H, m), 10.17 (1H, br, NH); 13C NMR (CD3SOCD3, 62.9 MHz): δ = 16.4, 16.6, 19.2, 63.7 (d, JPC = 6.9 Hz), 63.9 (d, JPC = 6.9 Hz), 68.5 (d, JPC = 173.6 Hz), 117.6, 118.7, 129.5, 130.4, 131.5, 131.7, 133.6, 137.6, 152.3; 31P NMR (CDCl3/H3PO4, 101 MHz): δ = 16.47. Anal. Calcd for C19H21NCl3O5P. C, 47.60; H, 4.42; N, 2.92%. Found: C, 47.48; H, 4.28; N, 2.81%.
Biochemical methods: AChE inhibition assay
Inhibitory activities of synthesized α-oxycarbanilinophosphonates were determined at 25°C by the colorimetric method of Ellman et al.Citation32. The assay solution contained 0.1 M phosphate buffer, pH 8, 0.3 mM 5,5′-dithiobis(2-nitrobenzoic acid) (DTNB), 0.125 units of AChE (Sigma Chemical Co. from electric eel (Electrophorous electricus)), and 0.2 mM acetylthiocholine iodide as the substrate in a total volume of 2.5 mL. In order to provide sufficient time for interaction with either PAS and/or active site of the enzyme, particularly in competition with the substrate, the synthesized compounds were added to the assay solution and preincubated with the enzyme for 10 min at 25°C. In order to solubilize the synthesized compounds, the reaction mixture contained 2% ethanol (50 µL in 2.5 mL reaction mixture volume). Control experiments were run and it was seen that no inhibitory effect was exerted on the enzyme by ethanol. The changes in absorbance at 412 nm were recorded for 5 min with a Camspec 501 Single Beam Scanning UV/Vis Spectrophotometer. Percent inhibitions were calculated and the concentration of the compounds that produced 50% inhibition of AChE (IC50) was determined through the use of Excel-Solver program. The IC50’s are the result of three independent experiments and reported as the average value ±SEM. The dose response curves were fit by nonlinear regression.
Computer-aided molecular modelling
Molecular Docking was carried out using AutoDock 4.2 and AutoDockTools version 1.5.4 with standard parametersCitation33. Crystal structure of EeAChE was obtained from protein data bank (pdb code 1C2O) and ligand structure was constructed and energy minimized using PRODRG online serverCitation34. All calculations were performed with the use of a PC with Intel Core 2 Quad 2.66 GHz Processor. Images were generated with PMVCitation33.
Results and discussion
We have reported synthesis of α-hydroxyphosphonate by the reaction of aldehyde with diethylphosphite in the presence of magnesia ()Citation31.
Initially, we carried out the reaction of benzaldehyde 1a with diethylphosphite in the presence of magnesia to afford diethyl 1-hydroxyphenylmethylphosphonate 2a in 85% isolated yield after 2 h. When the phenylisocyanate was added to diethyl 1-hydroxyphenylmethylphosphonate 2a in toluene, it afforded the corresponding α-oxycarbanilinophosphonate 4a in 88% isolated yield under microwave irradiation for 45 min. These results prompted us to extend this process to other 1-hydroxyphosphonates and isocyanates. Interestingly, 1-hydroxyphosphonates reacted smoothly with isocyanates under microwave irradiation to produce the corresponding α-oxycarbanilinophosphonates in good yields ( and ). As shown in , treatment of diethyl 1-hydroxyphenylmethylphosphonate (2a) with phenylisocyanate afforded diethyl[(anilinocarbonyl)oxy](phenyl)methylphosphonate (4a) in 88 % isolated yield. Other different substituted 1-hydroxyarylmethylphosphonates also reacted with phenylisocyanate to give the desired compounds (4b–4e) in good yields. Treatment of diethyl 1-hydroxy-3-(2-nitrophenyl)allyl phosphonate (2f), as an α,β-unsaturated 1-hydroxyphosphonate, also reacted with phenylisocyanate under ultrasonic irradiation to give the desired compound 4f in 71% yield. The reaction of diethyl (2-thiophenyl) hydroxymethylphosphonate (2g), as a heterocyclic 1-hydroxyphosphonate, with phenyl isocyanate gave 78% desired product 4g. The reaction of substituted isocyanates with 1-hydroxyphosphonate gave corresponding α-oxycarbanilinophosphonate 4h and 4i in good yields. The reaction of cyclohexylisocyanate, as an aliphatic isocyanate with diethyl 1-hydroxyphenylmethylphosphonate 2a gave the desired product 4j in 80% isolated yield.Diethyl 1-hydroxy-1-naphthylmethyl phosphonate (2k), as polynuclear 1-hydroxyarylmethylphosphonate, also reacted with benzylisocyanate under microwave irradiation, gave the desired compound 4l in 86 % isolated yield.
Table 1. Synthesis of novel α-oxycarbanilinophosphonates (4) and their anticholinesterase activities.
AChE inhibitory activity
Newly synthesized α-oxycarbanilinophosphonates were assayed for AChE (Electrophorus electricus) inhibition potency by the Ellman methodCitation32. The results are summarized in .
As it can be seen in , oxycarbanilinophosphonates have generally shown three kinds of effects on the target enzyme AChE: (1) no inhibition toward AChE (compounds 4a–4c, 4e, 4g, and 4j–4k), (2) moderate inhibitory effects (compounds 4d and 4l with IC50’s of 161.42 and 418.29 µM, respectively) and (3) the most potent inhibitory effects (compounds 4f, 4h, and 4i with IC50’s of 10.35, 6.36, and 7.47 µM, respectively).
In compound 4f there is no chemical group in the para position of the aromatic ring and instead there is a nitro group in the ortho position. The IC50 of the compound is 10.35 µM which is much lower than compound 4d and shows considerably higher inhibitory activity. Nitro group has caused the aromatic ring to become highly electron-deficient which makes it prepared to interact through π–π interaction with another aromatic ring system in the enzyme. Also the nitro group itself can make H-bond with potential side chains in the enzyme. The fulfillment of these two potentials has presumably made the IC50 of compound 4f that was much lower than compound 4d.
The highest inhibitory activity was obtained with compound 4h with an IC50 of 6.36 µM. Although we have not tried out to determine the inhibition mechanism, considering the fact that it can be deduced from molecular modelling results that the mode of inhibition is of mixed type inhibition, in the case of pure noncompetitive inhibition, Ki would be equal to IC50, that is 6.36 µMCitation35. This deduced Ki of compound 4h is comparable to and in a number of cases more potent than reported Ki’s for synthetic anticholinesterases in the literatureCitation36–38. The presence of two chlorine atoms at ortho and para positions of the aromatic ring has made the ring very electron-deficient and consequently well prepared for potential π–π interactions with aromatic side chains in the PAS. Also the acyl oxygen of the carbamate group can potentially make strong H-bond with an acceptor group in the enzyme because of its orientation and also because of the presence of a chlorine atom in the meta position of the aromatic ring close to carbamate group (in the Molecular modelling section we will explain more about the specific interactions of this compound with the enzyme).
Almost the same explanation as that provided for compound 4h can also be provided for compound 2i. Here, there is a nitro group at para position of the aromatic ring instead of two chlorine atoms at para and ortho positions in compound 4h. The nitro group has also caused the ring to become electron-deficient. The acylic oxygen of the carbamate group is also ready for a strong H-bond because of the presence of a chlorine atom on the proximal aromatic ring. These two changes are presumably the reasons for its very low IC50 of 7.47 µM.
Molecular modelling studies
In order to understand better the mode of interaction between the synthesized α-oxycarbanilinophosphonates and AChE, we studied the interactions between compound 4h and AChE through molecular modelling. Compound 4h was the most potent inhibitor, and could be considered as a representative member.
Our molecular docking calculations showed that compound 4h could only interact with peripheral site of AChE. The binding site was defined as a sphere around the Trp 286 with an arbitrary distance that included Ser 203 in the active site, together with the fact that a number of interacting residues around the drug and the drug itself were fully flexible. After 100 runs we observed that Trp 286 and Tyr 341 could interact through π–π interactions with both of the phenyl rings attached to phosphonate and carbamate moieties (). As it is clear from , there are also other residues whose interactions with the drug would be important in its proper orientation in the PAS. Asp 74 can interact electrostatically with the partially positive charge of the phosphorous atom and Tyr 72 may participate in another π–π interaction. We can also observe from that the compound 4h prefers to enter the gorge structure from its phenyl ring proximal to the carbamate group. And the other phenyl group proximal to the phosphonate group has been remained in the outside of the gorge structure. is a surface polarizability map of AChE that shows tri-dimensionally the drug and enzyme during binding interactions, together with the degree of the polarizability of the enzyme’s surface. The binding energies showed that the Asp 74 could have a determining role in making it favourable energetically the orientation of the drug shown in , where the Asp 74 is visible at the upper right hand corner of the gorge structure as a dark red spot, which shows its highly negative charge in comparison with other negatively-charged regions.
Figure 1. Stereo image presentation of peripheral site residues of the enzyme and Ser 203. The interacting residues are Trp 286 and Tyr 341. As it can be seen both of the phenyl rings of the compound 4h are interacting with the above-mentioned amino acid residues of the PAS through π–π interactions. The interactions of other residues such as Asp 74 and Tyr 72 are important in the appropriate orientation of the drug.
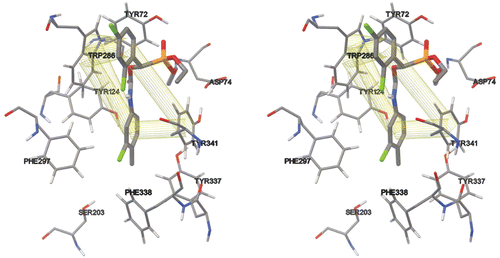
Figure 2. Top view of the surface palatability map of AChE that shows the tri-dimensional position of compound 4h at the peripheral site of the enzyme and its position respect to Ser 203. As it can be seen the orientation of the drug is such that its aromatic ring proximal to the carbamate group has been entered first and the other aromatic ring proximal to the phosphonate group is still outside. It seems that electrostatic interactions between the partially positive phosphorous atom and the negatively charged Asp 74 (shown in dark red colour at the upper right hand corner of the gorge structure) could be of importance in orienting the π–π interactions between the aromatic rings in the compound 4h and aromatic residues in the PAS (i.e. Trp 286 and Tyr 341).
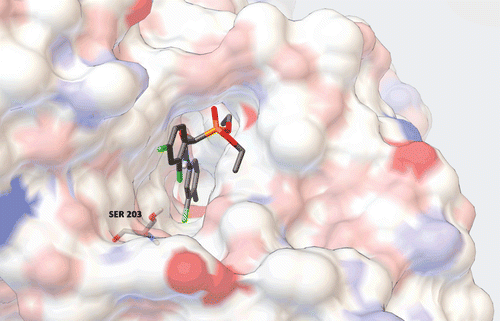
Conclusions
We have synthesized a family of α-oxycarbanilinophosphonates through a new synthetic methodology and have examined their inhibitory activities against electric eel AChE. These compounds have been synthesized by a reaction of α-hydroxyophosphonates with isocyanate under microwave irradiation. Variations in the aromatic rings proximal to phosphonate and carbamate moieties together with altering the distance between the aromatic ring and phosphonate group led us to identify that presence of an electron withdrawing group and atom such as nitro and chlorine, respectively, in the aromatic rings enhanced the AChE inhibitory potency. Molecular modelling studies showed that compound 4h interacts only with peripheral site of EeAChE, with the nature of protein ligand interactions being mainly hydrophobic. Our inhibition studies and also our molecular modelling simulations with the use of compound 4h showed that this group of compounds could be considered as a drug with two kinds of activities: First they can inhibit the catalytic activities of AChE with different potencies and second since our modelling studies showed that compound 4h interacts only with the PAS and can not enter the gorge structure, it may also inhibit the non-hydrolytic activity of AChE which is the promotion of amyloid aggregation. In this respect although these compounds could not be considered as dual binding inhibitors they may act as dual binding inhibitors.
Acknowledgments
The authors gratefully acknowledge support by the Institute for Advanced Studies in Basic Sciences (IASBS) Research Council under grant No. G20109IASBS120.
Declaration of interest
The authors report no conflicts of interest.
References
- Hilderbrand RL. The Role of Phosphonates in Living Systems. Boca Raton: CRC Press, F1 1982.
- Kaboudin B. Novel methods for the synthesis of phosphonate esters on the solid surface. Phosphorus, Sulfur, Silicon Relat Elem 2002;177:1749–1751.
- Redmore D. Topics in Phosphorus Chemistry. Griffith EJ, Grayson M, Eds.; Vol. In: 8; New YorkWiley, 1976.
- Kuntz L, Tritsch D, Grosdemange-Billiard C, Hemmerlin A, Willem A, Bach TJ et al. Isoprenoid biosynthesis as a target for antibacterial and antiparasitic drugs: phosphonohydroxamic acids as inhibitors of deoxyxylulose phosphate reducto-isomerase. Biochem J 2005;386:127–135.
- Kafarski P, Lejczak B, Tyka R, Koba L, Pliszczak E, Wieczorek P. Herbicidal activity of phosphonic, phosphinic, and phosphonous acid analogues of phenylglycine and phenylalanine. J Plant Growth Regul 1995;14:199–203.
- Vayron P, Renard PY, Taran F, Créminon C, Frobert Y, Grassi J et al. Toward antibody-catalyzed hydrolysis of organophosphorus poisons. Proc Natl Acad Sci USA 2000;97:7058–7063.
- Enz A, Amstutz R, Boddeke H, Gmelin G, Malanowski J. Brain selective inhibition of acetylcholinesterase: a novel approach to therapy for Alzheimer’s disease. Prog Brain Res 1993;98:431–438.
- Millard CB, Broomfield CA. Anticholinesterases: medical applications of neurochemical principles. J Neurochem 1995;64:1909–1918.
- Lindner A, Schalke B, Toyka KV. Outcome in juvenile-onset myasthenia gravis: a retrospective study with long-term follow-up of 79 patients. J Neurol 1997;244:515–520.
- Proctor GR, Harvey AL. Synthesis of tacrine analogues and their structure-activity relationships. Curr Med Chem 2000;7:295–302.
- Sugimoto H, Iimura Y, Yamanishi Y, Yamatsu K. Synthesis and structure-activity relationships of acetylcholinesterase inhibitors: 1-benzyl-4-[(5,6-dimethoxy-1-oxoindan-2-yl)methyl]piperidine hydrochloride and related compounds. J Med Chem 1995;38:4821–4829.
- Bai DL, Tang XC, He XC. Huperzine A, a potential therapeutic agent for treatment of Alzheimer’s disease. Curr Med Chem 2000;7:355–374.
- Winkler J, Thal LJ, Gage FH, Fisher LJ. Cholinergic strategies for Alzheimer’s disease. J Mol Med 1998;76:555–567.
- Mileson BE, Chambers JE, Chen WL, Dettbarn W, Ehrich M, Eldefrawi AT et al. Common mechanism of toxicity: a case study of organophosphorus pesticides. Toxicol Sci 1998;41:8–20.
- Sussman JL, Harel M, Frolow F, Oefner C, Goldman A, Toker L et al. Atomic structure of acetylcholinesterase from Torpedo californica: a prototypic acetylcholine-binding protein. Science 1991;253:872–879.
- Harel M, Quinn DM, Nair HK, Silman I, Sussman JL. The X-ray structure of a transition state analog complex reveals the molecular origins of the catalytic power and substrate specificity of acetylcholinesterase. J Am Chem Soc 1996;118:2340–2346.
- Bar-On P, Millard CB, Harel M, Dvir H, Enz A, Sussman JL et al. Kinetic and structural studies on the interaction of cholinesterases with the anti-Alzheimer drug rivastigmine. Biochemistry 2002;41:3555–3564.
- Taylor P, Lappi S. Interaction of fluorescence probes with acetylcholinesterase. The site and specificity of propidium binding. Biochemistry 1975;14:1989–1997.
- Stone PJ, Lucey EC, Noskova D, Digenis GA, Snider GL. Covalently linking a peptidyl carbamate elastase inhibitor to a hydrophilic polymer increases its effectiveness in preventing emphysema and secretory cell metaplasia in the hamster. Am Rev Respir Dis 1992;146:457–461.
- Uhlman E, Peyman A. Antisense oligonucleotides: a new therapeutic principle. Chem Rev 1990;90:543.
- Stein CA, Cheng YC. Antisense oligonucleotides as therapeutic agents–is the bullet really magical? Science 1993;261:1004–1012.
- Guo JX, Wu JJ, Wright JB, Lushington GH. Mechanistic insight into acetylcholinesterase inhibition and acute toxicity of organophosphorus compounds: a molecular modeling study. Chem Res Toxicol 2006;19:209–216.
- Failla S, Finochiaro P. Oxycarbanilinio derivatives of aralkyloxy-1 methylarylphosphonates: synthesis and characterization. Phosphorus, Sulfur, and Silicon 1996;1:285–293.
- Kaboudin B, Fallahi M. Ultrasound-assisted one-pot synthesis of α-oxycarbanilinophosphonates via a three component condensation of an aldehyde, diethyl phosphite and an isocyanate under solvent-free conditions. Tetrahedron Lett 2011;52: 4346–4348.
- Kappe CO. Controlled Microwave heating in modern organic synthesis. Angew Chem Int Ed 2004;43:6250–6284.
- Kaboudin B, Farjadian F. Synthesis of phosphorothioates using thiophosphate salts. Beilstein J Org Chem 2006;2:4.
- Kaboudin B, Norouzi H. A new, efficient, and simple method for the synthesis of thiiranes from epoxides under solvent-free conditions. Tetrahedron Lett 2004;45:1283–1285.
- Kaboudin B. A simple and new method for the synthesis of thiophosphates. Tetrahedron Lett 2002;43:8713–8714.
- Kaboudin B, Emadi S, Hadizadeh A. Synthesis of novel phosphorothioates and phosphorodithioates and their differential inhibition of cholinesterases. Bioorg Chem 2009;37:101–105.
- Kaboudin B, Emadi S, Norouzi H. A convenient method for the synthesis of phosphorothioates and their anticholinesterease activities. Phosphorus, Sulfur, Silicon Relat Elem 2004;179:585–590.
- Sardarain AR, Kaboudin B. Surface-mediated solid phase reactions: preparation of diethyl 1-hydroxyarylmethylphosphonates on the surface of magnesia. Synth Commun 1997;27:543–551.
- Ellman GL, Courtney KD, Andres V Jr, Feather-Stone RM. A new and rapid colorimetric determination of acetylcholinesterase activity. Biochem Pharmacol 1961;7:88–95.
- Sanner MF. Python: a programming language for software integration and development. J Mol Graph Model 1999;17:57–61.
- Schüttelkopf AW, van Aalten DMF. Biological crystallography. Acta Crystallogr 2004;D60:1355–1363.
- Copeland RA. Evaluation of Enzyme Inhibitors in Drug Discovery. Hoboken, NJWiley Interscience: , 2005, p. 131
- Leader H, Wolfe AD, Chiang PK, Gordon RK. Pyridophens: binary pyridostigmine-aprophen prodrugs with differential inhibition of acetylcholinesterase, butyrylcholinesterase, and muscarinic receptors. J Med Chem 2002;45:902–910.
- Gemma S, Gabellieri E, Huleatt P, Fattorusso C, Borriello M, Catalanotti B et al. Discovery of huperzine A-tacrine hybrids as potent inhibitors of human cholinesterases targeting their midgorge recognition sites. J Med Chem 2006;49:3421–3425.
- Baici A, Schenker P, Wächter M, Rüedi P. 3-Fluoro-2,4-dioxa-3-phosphadecalins as inhibitors of acetylcholinesterase. A reappraisal of kinetic mechanisms and diagnostic methods. Chem Biodivers 2009;6:261–282.