Abstract
We recently reported that synthetic derivatives of rutaecarpine alkaloid exhibited high acetyl cholinesterase (AChE) inhibitory activity and high selectivity for AChE over butyrylcholinesterases (BuChE). To explore novel effective drugs for the treatment of Alzheimer’s disease (AD), in this paper, further research results were presented. Starting from a structure-based drug design, a series of novel 2-(2-indolyl-)-4(3H)-quinazolines derivates were designed and synthesized as the ring-opened analogues of rutaecarpine alkaloid and subjected to pharmacological evaluation as AChE inhibitors. Among them, derivates 3a–c and 3g–h exhibited strong inhibitory activity for AChE and high selectivity for AChE over BuChE. The structure–activity relationships were discussed and their binding conformation and simultaneous interactions mode were further clarified by kinetic characterization and the molecular docking studies.
Abbreviations: | ||
AD, | = | Alzheimer’s disease; |
AChE, | = | acetylcholinesterase; |
BuChE, | = | butyrylcholinesterases; |
Aβ, | = | amyloid β-peptide; |
PAS, | = | peripheral anionic site; |
SAR, | = | structure–activity relationship; |
Introduction
Alzheimer’s disease (AD), the most common form of dementia affecting elderly people, is a complex irreversible neurological affection clinically characterized by a progressive loss of memory and impairment of cognitive functionsCitation1,Citation2. Unfortunately, the causes of AD have not been completely elucidated and no disease-modifying treatment is available yet. However, the cholinergic hypothesis of AD has provided the rationale for the current major therapeutic approach to the disease, which is aimed to correct the cognitive decline by enhancing cholinergic neurotransmissionCitation3,Citation4. Among them, cholinesterase (ChE) inhibition has been so for the most extensively used approach for the treatment of AD. To date, ChE inhibitors are the most effective drugs for the treatment of cognitive deficits and associated behavioural abnormalities in individuals with AD, such as donepezilCitation5, rivastigmineCitation6 and galantamineCitation7 ().
Two types of ChE were found in the central nervous system, including AChE and butyrylcholinesterase (BuChE)Citation8. Control of the AChE enzyme activity can be used for the treatment of diseases associated with acetylcholine depletionCitation9. X-ray crystallography of the AChE/inhibitor complexes indicated that AChE consists of a narrow gorge with two separate ligand-binding sites: an acylation site (CAS) at the bottom of the gorge and a peripheral anionic site (PAS) located at the gorge rimCitation10,Citation11. The CAS of AChE is located at the bottom of a narrow gorge, which consists of two sub-sites, an anionic and esteratic site. The second ‘anionic’ site, known as the PAS, lies around 14 Å from the active site. Recent studies have identified that the PAS might be somehow related with aggregation and deposition of amyloid-β (Aβ) peptide in AD patientsCitation12,Citation13. However, the functions of BuChE are still not clear. There is some evidence to suggest that BuChE activity may be involved in the pathogenesis of ADCitation14, although clinical experience shows that inhibition of ChEs is a palliative treatment, which does not address AD’s etiologyCitation15. But several reports have been published where it has been clearly shown that the design of central selective AChE inhibitors free from adverse peripheral effects remains a major challenging therapeutic goalCitation16–18.
The search for inhibitors of AChE that can simultaneously bind to its catalytic- and peripheral-binding sites has become an area of very active research. The success of the dual-binding site strategy is evidenced by the large increase in AChE inhibitory potency of these dimers or hybrids relative to the parent compounds from which they have been designedCitation19. Further interest comes from the fact that some of these dual-binding site AChE inhibitors have been shown to inhibit the aggregation of AβCitation20, which is a key event in the neurotoxic cascade of ADCitation21.
Based on this strategy, the authors have recently reported the synthesis, in vitro pharmacology and molecular modelling of a series of 3-aminoalkanamido-substituted rutaecarpine derivatives as AChE inhibitors of potential interest for the treatment of ADCitation22. The synthetic compounds exhibited strong inhibitory activity for AChE and high selectivity for AChE over BuChE. With the aim of gaining insights into the structure–activity relationships (SARs) and possibly identifying new lead/s in AChE inhibitors, the authors became interested in developing new rutaecarpine analogues based on rational drug design theory. In the present work, some new 2-(2-indolyl-)-4(3H)-quinazoline (2IQ) derivates were designed and synthesized by removal of the methylene groups in positions 7, 8 of rutaecarpine C-ring and introduction of a side chain with terminal amino group at the 3 or 5 position (). Meanwhile, biological evaluation, including AChE/BuChE inhibition, kinetic analysis and molecular modelling of the AChE inhibition for the novel rutaecarpine analogues were reported. The SARs were then discussed.
Experimental methods
Materials
All chemicals were obtained from commercial sources unless otherwise specified. Melting points were determined using an X-6 microscope melting point instrument and are uncorrected. Nuclear magnetic resonance (NMR) was recorded on a Bruker AvanceIII 400 spectrometer, whereas tetramethylsilane (TMS) (δ 0 ppm) was used as an internal standard for spectra recorded. The MS spectra were recorded on a Shimadzu LCMS-2010A instrument with an electrospray ionization (ESI) or atmospheric pressure chemical ionization (ACPI) mass selective detector. Elemental analysis was carried out on an Elementar Vario EL CHNS Elemental Analyzer.
The AChE (E.C. 3.1.1.7, from electric eel), BuChE (E.C. 3.1.1.8, from equine serum), 5,5′-dithiobis-(2-nitrobenzoic acid) (Ellman’s reagent, DTNB), butylthiocholine chloride (BTC), acetylthiocholine chloride (ATC) and tarcine hydrochloride were purchased from Sigma-Aldrich.
Chemistry
The preparation of a series of 2-(1H-indol-2-yl)quinazolin-4(3H)-one derivatives (3a–j) structurally related to rutaecarpine was accomplished using the general method outlined in . The intermediate 6-amino-2-(1H-indol-2-yl)quinazolin-4(3H)-one (1) was initially synthesized, according to the method described in the literatureCitation23. When the 2-ethyl-7-nitroquinazolin- 4(3H)-one (4) was not commercially available, it was prepared by treatment of the corresponding 2-amino-5-nitrobenzamide and orthoesters under classical heating. The 2-(1H-indol-2-yl)-6- nitroquinazolin-4(3H)-one (7) was prepared through the Fischer indolization of 7-nitro-2-(1-[2-phenylhydrazono]ethyl)-quinazolin-4(3H)-one (6) by heating in polyparabanic acid (PPA). Treatment of the 7 with hydrogen in the presence of 10% Palladium on carbon (Pd/C) provided the intermediate 1. The acylation of compound 1 with the appropriate acid halide gave the haloalkanamides 2a and 2b. Finally, the target compounds 3a–l were obtained by aminolysis of the compounds 2a and 2b, respectively, under reflux by the treatment with the appropriate secondary amines.
Scheme 1. Synthesis of 6-aminoalkanamido-substituted 2-(1H-indol-2-yl)quinazolin-4(3H)-one derivatives (3a–j). Reagents and conditions: (i) orthoesters, reflux, 3 h; (ii) tryptamine, 115°C, 3 h; (iii) HCI, AcOH, reflux, 1 h; (iv) (v) 10% Pd/C, H2, MeOH, 8 h; (vi) ClCO(CH2)nCl, CH2Cl2, reflux and (vii) HNR2, EtOH, KI, reflux (‘R-’ are shown in ).
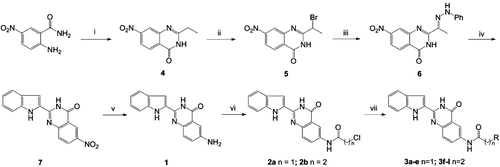
The synthetic method employed for the preparation of 9a and 9b is outlined in . Treatment of 2IQ with POCl3 in the presence of N,N-diethylaniline afforded the corresponding 4-chloro-2-(1H-indol-2-yl)quinazoline (8). These were subjected to the nucleophilic substitution of the 4-chloro group with N1,N1-dimethylpropane-1,3-diamine or N1,N1-diethylpropane-1,3-diamine in boiling benzene under reflux to give the corresponding target compounds 9a and 9b, respectively.
Scheme 2. Synthesis of 4-substituted 2-(1H-indol-2-yl)quinazolin-4(3H)-one derivatives (9a and 9b). Reagents and conditions: (i) POCl3, PhN(CH2CH3)2/Ph, reflux, 6 h and (ii) H2N(CH2)3NR2, EtOH, KI, reflux; (‘R-’ are shown in ).

2-ethyl-7-nitroquinazolin-4(3H)-one (4)
A stirred mixture of 2-amino-5-nitrobenzamide (20 mmol) and the triethyl orthopropionate (40 mmol) was heated under reflux for 1.5–3 h. The reaction was monitored by thin layer chromatography (TLC). The reaction mixture was cooled to 0°C and the pallide-flavens precipitate thus obtained was filtered off and recrystallized from ethanol to give pallide-flavens needles of 4 in 78% yield. 1H NMR (400 MHz, DMSO) δ 12.62 (s, 1H), 8.78 (d, J = 2.6 Hz, 1H), 8.50 (dd, J = 9.0, 2.7 Hz, 1H), 7.78 (d, J = 9.0 Hz, 1H), 2.69 (q, J = 7.5 Hz, 2H), 1.27 (t, J = 7.5 Hz, 3H). ESI-MS m/z: 220 [M+H]+.
2-(1-bromoethyl)-7-nitroquinazolin-4(3H)-one (5)
To a solution of 4 (10 mmol) and sodium acetate (8.2 g, 10 mmol) in acetic acid (120 mL) a solution of bromine (16.0 g, 10 mmol) in acetic acid (40 mL) was added drop-wise at 60°C. The reaction mixture was stirred while the colour of bromine disappeared. Then the reaction mixture was gradually poured into cold water. The precipitated crystals were filtered off, washed with water and dried to give 5 in 92% yield. 1H NMR (400 MHz, DMSO) δ 12.98 (s, 1H), 8.79 (d, J = 2.6 Hz, 1H), 8.55 (dd, J = 9.0, 2.7 Hz, 1H), 7.88 (d, J = 9.0 Hz, 1H), 5.12 (q, J = 6.8 Hz, 1H), 2.02 (d, J = 6.8 Hz, 3H). ESI-MS m/z: 297 [M+H]+.
7-nitro-2-(1-(2-phenylhydrazono)ethyl)quinazolin-4(3H)-one (6)
A solution of 5 (2.52 g, 10 mmol) and phenylhydrazine (4.32 g, 40 mmol) in ethanol (80 ml) was refluxed for 10 h. After cooling, the precipitated crystals were filtered off, washed with ethanol, dried and recrystallized from ethanol to give aurantia solid of 6 in 63% yield. 1H-NMR (400 MHz, DMSO-d6) δ 12.09 (s, 1H), 10.09 (s, 1H), 8.81 (d, J = 2.7, 1H), 8.52 (dd, J = 9.0, 2.7, 1H), 7.83 (d, J = 9.0, 1H), 7.66 (d, J = 8.0, 2H), 7.30 (t, J = 7.8, 2H), 2.35 (s, 3H). ESI-MS m/z: 324 [M+H]+.
2-(1H-indol-2-yl)-6-nitroquinazolin-4(3H)-one (7)
Hydrazone 6 (1 g, 3.59 mmol) was stirred in PPA (5 mL) at 120°C for 30 min. The reaction mixture was poured into water (50 mL) and the precipitated crystals were filtered off, washed with ethanol, dried and recrystallized from aqueous dimethylformamide (DMF) to give brown solid of 7 in 32% yield. 1H-NMR (400 MHz, DMSO-d6) δ 12.25 (s, 1H), 11.64 (s, 1H), 11.04 (s, 1H), 9.74 (s, 1H), 8.67 (d, J = 1.8, 1H), 8.06 (d, J = 8.7, 1H), 7.51 (d, J = 7.9, 2H), 7.27 (s, 1H), 7.03 (t, J = 7.5, 1H). ESI-MS m/z: 307 [M+H]+.
6-amino-2-(1H-indol-2-yl)quinazolin-4(3H)-one (1)
To a solution of 7 (1.00 g, 3.62 mmol) in MeOH (200 mL) Pd/C (10%) was added prior to purging with H2 (g), and the mixture was stirred at room temperature until reaction was complete. The catalyst was removed by filtration and the solvent was removed in vacuo to give a brown solid of 1 in 72% yield. 1H-NMR (400 MHz, DMSO-d6) δ 12.25 (s, 1H), 11.64 (s, 1H), 11.04 (s, 1H), 9.74 (s, 1H), 7.60 (d, J = 7.9, 1H), 7.51 (d, J = 7.9, 2H), 7.27–7.23 (m, 2H), 7.04 (t, J = 7.5, 1H), 5.70 (s, 2H). ESI-MS m/z: 277 [M+H]+.
General acylation procedure for the synthesis of compounds 2a–b
A solution of the appropriate acid halide (4.8 mmol) in CH2Cl2 (5 mL) was added during to a well-stirred mixture of the amino-substituted compounds 1 (4 mmol) and K2CO3 (0.8 g) in CH2Cl2 (75 mL) at room temperature. After completed addition, the solution was finally refluxed for 4–6 h. After cooling to 0°C–5°C, the precipitate formed was filtered off and was further purified by flash column chromatography with petroleum ether/EtOAc (10:1) elution to give the compounds 2a–b.
N-(2-(1H-indol-2-yl)-4-oxo-3,4-dihydroquinazolin-6-yl)-2-chloroacetamide (2a)
The compound 1 was treated with chloroacetyl chloride according to general acylation procedure to afford 2a as brown solid in 75% yield; 1H-NMR (400 MHz, DMSO-d6) δ 12.62 (s, 1H), 11.83 (s, 1H), 11.12 (s, 1H), 8.57 (d, J = 1.8, 1H), 8.02 (dd, J = 8.7, 2.0, 1H), 7.73 (d, J = 8.8, 1H), 7.65–7.60 (m, 2H), 7.52 (d, J = 20.3, 2H), 7.25 (t, J = 7.4, 1H), 7.04 (s, 1H), 4.37 (d, J = 8.5, 2H). ESI-MS m/z: 353 [M+H]+.
N-(2-(1H-indol-2-yl)-4-oxo-3,4-dihydroquinazolin-6-yl)-3-chloropropanamide (2b)
The compound 1 was treated with 3-chloropropionyl chloride according to general acylation procedure to afford 2b as brown solid in 82% yield; 1H-NMR (400 MHz, DMSO-d6) δ 12.56 (s, 1H), 11.79 (s, 1H), 10.65 (s, 1H), 8.57 (d, J = 2.2, 1H), 8.01 (dd, J = 8.8, 2.4, 1H), 7.72 (dd, J = 8.8, 4.4, 1H), 7.66–7.61 (m, 2H), 7.53 (d, J = 8.2, 2H), 7.25–7.20 (m, 1H), 7.06 (t, J = 7.5, 1H), 3.93 (t, J = 6.2, 2H), 2.92 (t, J = 6.2, 2H). ESI-MS m/z: 367 [M+H]+.
General aminolysis procedure for the synthesis of compounds 3a–j
To a stirred refluxing suspension of the haloalkanamido-substituted compounds 2a–b (1.5 mmol) and NaI (0.15 g) in EtOH (40 mL) appropriate secondary amine (1.0 mL) in EtOH (10 mL) was added drop-wise. The mixture was stirred at reflux for 3 h, cooled to 0°C, filtered, and washed with ether and water, then evaporated under vacuum. The crude solid was purified by chromatography with petroleum ether/EtOAc (20:1) elution or crystallization from EtOH/DMF (5:1 v/v) to afford 3a–j.
N-(2-(1H-indol-2-yl)-4-oxo-3,4-dihydroquinazolin-6-yl)-2-(diethylamino)acetamide (3a)
The compound 2a was treated with excess diethylamine according to general acylation procedure to afford 3a, after column chromatography with petroleum ether/EtOAc (10:1) elution, as grey solid in 87% yield; m.p. 248°C–249°C; 1H-NMR (400 MHz, DMSO-d6) δ 12.54 (s, 1H), 11.75 (s, 1H), 10.02 (s, 1H), 8.55 (s, 1H), 8.06 (d, J = 7.1, 1H), 7.70 (d, J = 8.8, 1H), 7.63 (d, J = 5.3, 2H), 7.52 (d, J = 8.2, 1H), 7.24 (t, J = 7.5, 1H), 7.08 (t, J = 7.4, 1H), 3.24 (s, 2H), 2.67–2.61 (m, 4H), 1.05 (d, J = 7.0, 6H). 13C NMR (101 MHz, DMSO-d6) δ 170.10, 161.61, 145.25, 144.66, 137.56, 136.64, 130.14, 127.44 (d, J = 4.2), 126.69, 123.87, 121.37 (d, J = 5.5), 119.87, 115.03, 112.30, 104.50, 57.26, 47.79, 11.81. HRMS (ESI): calcd for (M+H)+ (C22H23N5O2) requires m/z 390.1930, found 390.1928. Anal calcd for C22H23N5O2·1/2H2O: C, 66.31; H, 6.07; N, 17.58; O, 10.04. Found: C, 66.35; H, 6.08; N, 17.52; O, 10.05.
N-(2-(1H-indol-2-yl)-4-oxo-3,4-dihydroquinazolin-6-yl)-2-(pyrrolidin-1-yl)acetamide (3b)
The compound 2a was treated with excess pyrrolidine according to general acylation procedure to afford 3b after column chromatography with petroleum ether/EtOAc (10:1) elution, as beige solid in 78% yield; m.p. 257°C–258°C; 1H-NMR (400 MHz, DMSO-d6) δ 12.30 (s, 1H), 11.75 (s, 1H), 10.10 (s, 1H), 8.57 (s, 1H), 8.04 (t, J = 10.2, 1H), 7.70 (t, J = 7.5, 1H), 7.63 (d, J = 6.3, 2H), 7.53 (d, J = 8.1, 1H), 7.22 (t, J = 7.3, 1H), 7.06 (t, J = 7.2, 1H), 3.29 (s, 2H), 2.62 (s, 4H), 1.77 (s, 4H). 13C NMR (101 MHz, DMSO-d6) δ 169.10, 161.66, 145.29, 144.61, 137.56, 136.93, 130.16, 127.47, 126.70, 123.85, 121.34 (d, J = 8.2, 4H), 119.86, 115.07, 112.30, 104.48, 59.49, 53.63, 23.45. HRMS (ESI): calcd for (M+H)+ (C22H21N5O2) requires m/z 388.1774, found 388.1782. Anal calcd for C22H21N5O2·H2O: C, 65.17; H, 5.72; N, 17.27; O, 11.84. Found: C, 65.20; H, 5.69; N, 17.25; O, 11.86.
N-(2-(1H-indol-2-yl)-4-oxo-3,4-dihydroquinazolin-6-yl)-2-(piperidin-1-yl)acetamide (3c)
The compound 2a was treated with excess piperidine according to general acylation procedure to afford 3c after column chromatography with petroleum ether/EtOAc (10:1) elution, as grey solid in 65% yield; m.p. 261°C–262°C; 1H-NMR (400 MHz, DMSO-d6) δ 12.27 (s, 1H), 11.76 (s, 1H), 10.04 (s, 1H), 8.55 (d, J = 2.1, 1H), 8.05 (dd, J = 8.8, 2.2, 1H), 7.71 (d, J = 8.8, 1H), 7.64 (d, J = 6.4, 2H), 7.53 (d, J = 8.2, 1H), 7.22 (t, J = 7.5, 1H), 7.06 (t, J = 7.4, 1H), 3.13 (s, 2H), 2.50–2.47 (m, 4H), 1.62–1.40 (m, 6H). 13C NMR (101 MHz, DMSO) δ 168.92, 161.66, 145.29, 144.61, 137.57, 136.76, 130.17, 127.42 (d, J = 12.2), 126.69, 123.85, 121.35 (d, J = 6.1), 119.86, 115.05, 112.30, 104.50, 79.12, 62.66, 54.04, 25.39, 23.53. HRMS (ESI): calcd for (M+H)+ (C23H23N5O2) requires m/z 402.1930, found 402.1944. Anal calcd for C23H23N5O2·H2O: C, 65.85; H, 6.01; N, 16.70; O, 11.44. Found: C, 65.81; H, 6.04; N, 16.68; O, 11.47.
N-(2-(1H-indol-2-yl)-4-oxo-3,4-dihydroquinazolin-6-yl)-2-morpholinoacetamide (3d)
The compound 2a was treated with excess morpholine according to general acylation procedure to afford 3d after column chromatography with petroleum ether/EtOAc (10:1) elution, as off-white solid in 72% yield; m.p. 241°C–242°C; 1H-NMR (400 MHz, DMSO-d6) δ 12.44 (s, 1H), 11.76 (s, 1H), 10.07 (s, 1H), 8.54 (s, 1H), 8.04 (d, J = 8.2, 1H), 7.71 (d, J = 8.7, 1H), 7.63 (s, 2H), 7.53 (d, J = 8.0, 1H), 7.22 (t, J = 7.3, 1H), 7.06 (t, J = 7.2, 1H), 3.26 (d, J = 65.5, 4H), 2.51 (s, 2H), 2.19 (s, 4H). 13C NMR (101 MHz, DMSO-d6) δ168.59, 161.64, 145.29, 144.63, 137.57, 136.76, 130.16, 127.47, 126.66, 123.86, 121.35, 119.87, 115.04, 112.30, 104.51, 61.77, 54.47, 52.63, 45.68. HRMS (ESI): calcd for (M+H)+ (C22H21N5O3) requires m/z 404.1723, found 404.1732. Anal calcd for C22H21N5O3·3/2H2O: C, 61.38; H, 5.62; N, 16.27; O, 16.73. Found: C, 61.40; H, 5.65; N, 16.26; O, 16.70.
N-(2-(1H-indol-2-yl)-4-oxo-3,4-dihydroquinazolin-6-yl)-2-(4-methylpiperazin-1-yl)acetamide (3e)
The compound 2a was treated with excess methylpiperazine according to general acylation procedure to afford 3e after column chromatography with petroleum ether/EtOAc (10:1) elution, as yellow-white solid in 78% yield; m.p. 269°C–270°C; 1H-NMR (400 MHz, DMSO-d6) δ 12.24 (s, 1H), 11.75 (s, 1H), 10.11 (s, 1H), 8.54 (d, J = 2.2, 1H), 8.05 (dd, J = 8.8, 2.3, 1H), 7.71 (d, J = 8.8, 1H), 7.64 (d, J = 7.0, 2H), 7.53 (d, J = 8.2, 1H), 7.22 (t, J = 7.5, 1H), 7.06 (t, J = 7.5, 1H), 3.71–3.60 (m, 4H), 3.20 (s, 2H), 2.59–2.52 (m, 4H), 2.50 (s, 3H). 13C NMR (101 MHz, DMSO-d6) δ 168.40, 161.61, 145.29, 144.67, 137.57, 136.75, 130.14, 127.47, 126.74, 123.87, 121.35 (d, J = 8.0), 119.87, 115.17, 112.30, 104.52, 79.24, 78.91, 78.58, 66.03, 62.03, 53.13. HRMS (ESI): calcd for (M+H)+ (C23H24N6O2) requires m/z 417.2389, found 417.2376. Anal calcd for C23H24N6O2·H2O: C, 63.58; H, 6.03; N, 19.34; O, 11.05. Found: C, 63.55; H, 6.05; N, 19.32; O, 11.08.
N-(2-(1H-indol-2-yl)-4-oxo-3,4-dihydroquinazolin-6-yl)-3-(diethylamino)propanamide (3f)
The compound 2b was treated with excess diethylamine according to general acylation procedure to afford 3f, after column chromatography with petroleum ether/EtOAc (10:1) elution, as grey solid in 67% yield; m.p. 226°C–227°C; 1H-NMR (400 MHz, DMSO-d6) δ 12.45 (s, 1H), 11.78 (s, 1H), 10.64 (s, 1H), 8.54 (d, J = 1.5, 1H), 7.99 (dd, J = 8.8, 2.0, 1H), 7.71 (d, J = 8.8, 1H), 7.63 (d, J = 4.1, 2H), 7.54 (d, J = 8.2, 1H), 7.22 (t, J = 7.6, 1H), 7.06 (t, J = 7.5, 1H), 2.85 (s, 2H), 2.60–2.55 (m, 4H), 2.51 (s, 2H), 1.02 (t, J = 7.0, 6H). 13C NMR (101 MHz, DMSO-d6) δ 169.46, 160.58, 144.08, 143.33, 136.51, 136.40, 129.73, 129.15, 126.43, 125.16, 122.77, 120.32, 120.02, 118.80, 113.40, 111.25, 103.43, 47.08, 45.06, 32.80, 29.57, 10.38. HRMS (ESI): calcd for (M+H)+ (C23H25N5O2) requires m/z 404.2087, found 404.2101. Anal calcd for C23H25N5O2·1/2H2O: C, 66.97; H, 6.35; N, 16.98; O, 9.70. Found: C, 66.99; H, 6.35; N, 16.97; O, 9.69.
N-(2-(1H-indol-2-yl)-4-oxo-3,4-dihydroquinazolin-6-yl)-3-(pyrrolidin-1-yl)propanamide (3g)
The compound 2b was treated with excess pyrrolidine according to general acylation procedure to afford 3g after column chromatography with petroleum ether/EtOAc (10:1) elution, as beige solid in 78% yield; m.p. 233°C–234°C; 1H-NMR (400 MHz, DMSO-d6) δ 12.25 (s, 1H), 11.76 (s, 1H), 10.51 (s, 1H), 8.51 (s, 1H), 7.93 (d, J = 7.8, 1H), 7.70 (d, J = 8.7, 1H), 7.53 (d, J = 7.9, 1H), 7.23 (d, J = 6.6, 1H), 7.07 (d, J = 7.0, 1H), 3.34 (s, 2H), 2.74 (d, J = 6.7, 2H), 2.55–2.50 (m, 4H), 1.69 (s, 4H). 13C NMR (101 MHz, DMSO-d6) δ 170.61, 161.70, 145.20, 144.67, 137.45 (d, J = 17.8), 130.31, 127.49, 126.17, 125.28, 123.80, 121.37, 119.84, 118.88, 114.48, 112.29, 104.41, 53.37, 51.46, 36.17, 24.43, 23.14. HRMS (ESI): calcd for (M+H)+ (C23H23N5O2) requires m/z 402.1930, found 402.1948. Anal calcd for C23H23N5O2·H2O: 65.85; H, 6.01; N, 16.70; O, 11.44. Found: C, 65.88; H, 5.98; N, 16.72; O, 11.46.
N-(2-(1H-indol-2-yl)-4-oxo-3,4-dihydroquinazolin-6-yl)-3-(piperidin-1-yl)propanamide (3h)
The compound 2b was treated with excess piperidine according to general acylation procedure to afford 3h after column chromatography with petroleum ether/EtOAc (10:1) elution, as grey solid in 51% yield; m.p. 239°C–240°C; 1H-NMR (400 MHz, DMSO-d6) δ 12.25 (s, 1H), 11.76 (s, 1H), 10.51 (s, 1H), 8.51 (s, 1H), 7.93 (d, J = 7.8, 1H), 7.63 (d, J = 6.1, 2H), 7.53 (d, J = 7.9, 1H), 7.30–7.17 (m, 1H), 7.13–7.02 (m, 1H), 2.62 (d, J = 5.6, 2H), 2.51 (s, 2H), 2.40 (s, 4H), 1.45 (d, J = 47.5, 6H). 13C NMR (101 MHz, DMSO-d6) δ 170.61, 161.70, 145.20, 144.67, 137.46 (d, J = 17.7), 130.20, 127.48, 126.16, 123.85, 121.38, 119.87, 114.42, 112.29, 104.45, 54.34, 53.61, 34.11, 30.63, 25.58, 23.95. HRMS (ESI): calcd for (M+H)+ (C24H25N5O2) requires m/z 416.2087, found 416.2089. Anal calcd for C24H25N5O2·H2O: C, 66.49; H, 6.28; N, 16.16; O, 11.07. Found: C, 66.48; H, 6.26; N, 16.17; O, 11.09.
N-(2-(1H-indol-2-yl)-4-oxo-3,4-dihydroquinazolin-6-yl)-3-morpholinopropanamide (3i)
The compound 2b was treated with excess morpholine according to general acylation procedure to afford 3i after column chromatography with petroleum ether/EtOAc (10:1) elution, as off-white solid in 38% yield; m.p. 235°C–236°C;Citation1H-NMR (400 MHz, DMSO-d6) δ 12.38 (s, 1H), 11.75 (s, 1H), 10.42 (s, 1H), 8.51 (s, 1H), 7.94 (dd, J = 8.8, 2.0, 1H), 7.70 (d, J = 8.8, 1H), 7.63 (d, J = 7.2, 2H), 7.53 (d, J = 8.3, 1H), 7.22 (t, J = 7.5, 1H), 7.06 (t, J = 7.5, 1H), 2.66 (t, J = 6.8, 2H), 2.51 (d, J = 7.7, 4H), 2.33 (s, 2H), 2.16 (s, 4H). 13C NMR (101 MHz, DMSO-d6) δ 170.45, 161.60, 145.14, 144.40, 137.46 (d, J = 18.9), 130.16, 127.47, 126.18, 123.85, 121.38, 119.87, 114.46, 112.29, 104.47, 54.66, 53.61, 52.25, 45.59, 34.18, 30.63. HRMS (ESI): calcd for (M+H)+ (C23H23N5O3) requires m/z 418.1879, found 418.1880. Anal calcd for C23H23N5O3·3/2H2O: C, 62.15; H, 5.90; N, 15.76; O, 16.20. Found: C, 62.17; H, 5.93; N, 15.73; O, 16.17.
N-(2-(1H-indol-2-yl)-4-oxo-3,4-dihydroquinazolin-6-yl)-3-(4-methylpiperazin-1-yl)propanamide (3j)
The compound 2b was treated with excess methylpiperazine according to general acylation procedure to afford 3j after column chromatography with petroleum ether/EtOAc (10:1) elution, as yellow solid in 41% yield; m.p. 245°C–246°C; 1H-NMR (400 MHz, DMSO-d6) δ 12.53 (s, 1H), 11.76 (s, 1H), 10.39 (s, 1H), 8.53 (s, 1H), 7.96 (d, J = 7.9, 1H), 7.71 (d, J = 8.7, 1H), 7.64 (s, 2H), 7.54 (d, J = 8.0, 1H), 7.23 (t, J = 7.3, 1H), 7.06 (t, J = 7.2, 1H), 3.59 (s, 4H), 3.35 (s, 2H), 2.67 (d, J = 6.3, 2H), 2.59–2.46 (m, 4H), 2.43 (s, 3H). 13C NMR (101 MHz, DMSO-d6) δ 170.34, 161.61, 145.13, 144.41, 137.45 (d, J = 19.2), 130.15, 127.50 (d, J = 7.8), 126.20, 123.85, 121.38, 119.87, 114.46, 112.29, 104.46, 66.11, 54.04, 52.97, 33.90. HRMS (ESI): calcd for (M+H)+ (C24H26N6O2) requires m/z 431.2195, found 431.2183. Anal calcd for C24H26N6O2·H2O: C, 64.27; H, 6.29; N, 18.74; O, 10.70. Found: C, 64.30; H, 6.25; N, 18.71; O, 10.65.
4-chloro-2-(1H-indol-2-yl)quinazoline (8)
A solution of 2-(1H-indol-2-yl)quinazolin-4(3H)-one (2.61 g, 10.0 mmol), N,N-diethylaniline (4.75 mL, 15.0 mmol) and dry benzene (100 mL) was refluxed for 5 min. Phosphorus oxychoride (0.9 mL, 8.0 mmol) was added by syringe, and the resultant mixture was refluxed for 6 h maintaining anhydrous conditions by use of a CaCl2 drying tube attached to the condenser. The solution was cooled to room temperature and filtered. The insoluble precipitate was washed with dry benzene (40 mL). The combined filtrate was rapidly washed sequentially with ice water (100 mL), ice-cooled 20% sodium hydroxide (100 mL). The organic layer was immediately washed with a HCl solution (1 M, 100 mL) and water (100 mL) and dried over sodium sulphate. The solvent was removed in vacuo to give as light yellow solid. Purified by column chromatography (CHCl3 / MeOH 10:1) elution to afford 8, as white solid in 21% yield; 1H NMR (400 MHz, CDCl3) δ 9.53 (s, 1H), 8.23 (dd, J = 8.3, 0.7, 1H), 8.03 (d, J = 8.4, 1H), 7.92 (ddd, J = 8.4, 6.9, 1.3, 1H), 7.72 (dd, J = 8.0, 0.5, 1H), 7.66− 7.59 (m, 2H), 7.47 (dd, J = 8.3, 0.7, 1H), 7.32–7.27 (m, 1H), 7.17–7.12 (m, 1H). ESI-MS m/z: 280 [M+H]+.
N1-(2-(1H-indol-2-yl)quinazolin-4-yl)-N3,N3-dimethylpropane-1,3-diamine (9a)
A solution of 8 (0.28 g, 1.0 mmol), triethylamine (0.1–0.2 mL) and dry benzene (10 mL) was refluxed for 5 min. Then N1,N1-dimethylpropane-1,3-diamine (130 μL, 1.0 mmol) was added drop-wise, and the resultant mixture was refluxed for 3 h. The solution was cooled to room temperature and rapidly washed sequentially with ice water (50 mL). The organic layer was dried over sodium sulphate, then the solvent was removed in vacuo. Purified by column chromatography with chloroform/MeOH (10:1) elution to afford 9a, as grey solid in 47% yield; m.p. 215°C–216°C; 1H-NMR (400 MHz, DMSO-d6) δ 11.50 (s, 1H), 8.40 (t, J = 5.0, 1H), 8.28–8.11 (m, 2H), 7.95 (d, J = 3.6, 1H), 7.77 (s, 1H), 7.55 (d, J = 7.7, 1H), 7.29 (s, 1H), 7.19–7.12 (m, 1H), 7.06–7.01 (m, 1H), 4.31 (s, 1H), 3.76 (dd, J = 12.2, 6.4, 2H), 2.41 (t, J = 6.9, 2H), 2.20 (s, 6H), 1.89 (dt, J = 13.7, 6.8, 2H). 13C NMR (101 MHz, DMSO-d6) δ 159.42, 155.23, 149.69, 137.66 (d, J = 3.6), 137.18, 134.61, 132.65, 128.01, 127.18, 126.04, 124.88, 122.60 (d, J = 15.2), 121.48, 120.87, 119.28, 113.94, 112.66, 104.87 (d, J = 8.3), 103.39, 57.12, 54.34, 45.18, 26.54. HRMS (ESI): calcd for (M+H)+ (C21H23N5) requires m/z 346.2023, found 346.2020. Anal calcd for C21H23N5: C, 73.02; H, 6.71; N, 20.27. Found: C, 73.05; H, 6.70; N, 20.25.
N1-(2-(1H-indol-2-yl)quinazolin-4-yl)-N3,N3-diethylpropane-1,3-diamine (9b)
A solution of 8 (0.28 g, 1.0 mmol), triethylamine (0.1–0.2 mL) and dry benzene (10 mL) was refluxed for 5 min. Then N1,N1-diethylpropane-1,3-diamine (160 μL, 1.0 mmol) was added drop-wise, and the resultant mixture was refluxed for 3 h. The solution was cooled to room temperature and rapidly washed sequentially with ice water (50 mL). The organic layer was dried over sodium sulphate, then the solvent was removed in vacuo. Purified by column chromatography with chloroform/MeOH (10:1) elution to afford 9b, as off-white solid in 47% yield; m.p. 208°C–209°C; 1H-NMR (400 MHz, DMSO-d6) δ 11.62 (s, 1H), 8.21 (d, J = 8.1, 1H), 7.76–7.71 (m, 2H), 7.60 (d, J = 7.9, 1H), 7.46–7.41 (m, 1H), 7.28 (s, 1H), 7.15 (t, J = 7.5, 1H), 7.01 (t, J = 7.4, 1H), 3.63 (s, 1H), 3.33 (s, 2H), 3.15 (d, J = 7.1, 4H), 2.05 (s, 2H), 1.05 (t, J = 6.9, 6H). 13C NMR (101 MHz, DMSO-d60) δ 159.35, 155.30, 149.73, 137.71, 137.15, 132.54, 128.04, 127.06, 124.79, 122.98, 122.43, 120.81, 119.23, 114.19, 112.19, 103.15, 61.93, 59.20, 46.27, 22.03, 11.56, 8.09. HRMS (ESI): calcd for (M+H)+ (C23H27N5) requires m/z 374.2345, found 374.2351. Anal calcd for C23H27N5: C, 73.96; H, 7.29; N, 18.75. Found: C, 73.99; H, 7.28; N, 18.73.
In vitro inhibition studies on AChE and BuChE
The method of Ellman et al.Citation24 was followed for the in vitro inhibition studies on AChE and BuChE. All the assays were under 0.1 M KH2PO4/K2HPO4 buffer, pH 8.0, using a Shimadzu 2450 Spectrophotometer. Enzyme solutions were prepared to give 2 units/mL in 2 mL aliquots. The assay medium contained phosphate buffer, pH 8.0 (1 mL), 50 μL of 0.01 M DTNB, 10 μL of enzyme and 50 μL of 0.01 M substrate (acetylthiocholine chloride). The substrate was added to the assay medium containing enzyme, buffer and DTNB with inhibitor after 15 min of incubation time. The activity was determined by measuring the increase in absorbance at 412 nm at 1 min intervals at 37°C. The reaction rates were compared, and the percentage inhibition due to the presence of test compounds was calculated. Each concentration was analysed in triplicate, and IC50 values were determined graphically from log concentration–inhibition curves.
Kinetic characterization of AChE and BuChE was performed using a reported method. Test compound was added into the assay solution and pre-incubated with the enzyme at 37°C for 15 min, followed by the addition of substrate. Kinetic characterization of the hydrolysis of ATC catalysed by AChE was done spectrometrically at 412 nm. A parallel control with no inhibitor in the mixture allowed adjusting activities to be measured at various times. The plots were assessed by a weighted least square analysis that assumed the variance of V to be a constant percentage of V for the entire data set. Slopes of these reciprocal plots were then plotted against the concentration of the inhibitors in a weighted analysis and Ki was determined as the ratio of the replot intercept to the replot slope. Kinetic characterization of BuChE assay uses the similar method described above.
Molecular modelling
The initial model of AChE (code ID:1EVE) and BuChE (code ID: 1POI) for docking studies were built based on the X-ray crystal structure of the E2020–AChE complex and Echothiophate–BuChE complex, respectively, which were obtained from the Protein Data Bank after eliminating the inhibitor and water molecules. The 3D structures of the compounds 3h and 9a were generated and optimized by Discovery studio 2.1 package (Accelrys Inc., San Diego, CA, USA). AUTODOCK 4.0 package was used to perform docking simulations, which adopts the hybrid Lamarckian Genetic Algorithm as searching algorithm and allows full flexibility of the ligand. Solvation parameters and Kollman charges for all atoms in AChE and BuChE were assigned by using AUTODOCK Tools. The grid for energy evaluation was centred at the bottom of the active site gorge (AChE [2.781 64.383 67.971]; BuChE [112.0 20.0 40.0]) with grid points in the x-, y-, z-axes set to 50 × 46 × 46 Å and separated by 0.375 Å. Flexible ligand docking was performed for the compounds. The initial population size and maximum number of energy evaluations were set to 100 and 2,500,000, respectively. The docked results within an RMSD of 1.5 Å were clustered, and the final results of each ligand were selected considering both the embedded empirical binding free energy evaluation and the clustering analysis.
Results and discussion
Enzyme inhibition assays
The biological profiles of the novel 2-(2-indolyl-)-4(3H)-quinazolines derivates towards AChE and BuChE in vitro were determined and expressed as IC50 values, and the inhibitory activities were reported in . The ability of compounds 3a–j and 9a–b to inhibit AChE and BuChE activities was assessed employing the modified Ellman’s method and using tacrine as reference compound. The AChECitation24 from electric eel and BuChE from equine serum were purchased from Sigma Corporation.
Table 1. In vitro inhibition IC50a and selectivity index of derivatives 3a–j and 9a–b on AChE and BuChE.
Among the molecules screened for the AChE inhibitory activity, most of these compounds exhibited strong inhibitory activity for AChE and high selectivity for AChE over BuChE. With the same basic terminus, compounds 3a–d with side chains of the same length (n = 2) displayed higher inhibitory activity and selectivity towards AChE than compounds 3f–i with shorter side chains (n = 1). Compound 3h was the most potent for AChE inhibition, which presented an IC50 value of 20.97 nM. These results showed a similar trend with the reported rutaecarpine derivatives as Wang et al.Citation22, which was also consistent with the literature that the introduction of the aminoalkanamido-substituted group at the 6-position of 2IQ could significantly increase the inhibitory activity of derivatives, and the higher inhibitory potency was associated with piperidine at the end of side chain. However, the compounds (3e, 3j) with piperazine did not show any inhibitory activity. In addition, the compounds (9a, 9b), introduced side chains of terminal groups−NR into 3-position of 2IQ, also did not exhibit any inhibitory activity. Most of the active derivatives proved to be selective for AChE with respect to BChE. Particularly, compound 3h gave the highest inhibitory activity and the highest selectivity index (349-fold). Moreover, the selectivity of the 2IQ derivatives also showed a similar trend with the reported rutaecarpine derivatives.
It may be observed from the SAR studies that after comparing inhibitory activity of 2IQ derivatives with the reported rutaecarpine derivatives, it was found that the compounds exhibited strong ChE-inhibiting properties. It has a similar trend with the reported rutaecarpine derivatives by Wang et al.Citation22, and a reversed-binding profile compared with the rutaecarpine derivatives without side chainsCitation25. It may be observed that the aromatization and flexibility of molecules increases after removal of the methylene groups at 6, 7 position of rutaecarpine C-ring. These results further demonstrated that the aromatic planar moiety of compounds could be in favour for interacting to the enzyme-binding site, while the flexibility of molecules might be a disadvantageous factor for binding affinity of compounds with enzyme, which was pointed out in our previous study. On the other hand, through studying the crystal structure and related literature of AChE, it was known that the AChE has a catalytic site at the bottom of deep and narrow gorge and a peripheral site at the entrance. Based on the preceding considerations and on the hypothesis that the rather wide shape of molecules, such as compounds 9a, 9b, might impair the entrance into the AChE gorge, it can be seen that the terminal groups of side chains of these compounds elongated into the bottom of the gorge with difficulty and could badly interact with the internal residue at the catalytic site. As a result, compounds 9a, 9b also did not exhibit any inhibitory activity.
To investigate the binding mode of the target compounds synthesized, the most potent compound (3h) was selected for kinetic measurements. The inhibition mechanisms towards both ChE s were analysed by recording substrate–velocity curves in the presence of varying concentrations of the inhibitor. The inhibitor concentrations selected were slightly higher and lower than the respective IC50 value ().The nature of AChE inhibition caused by the most potent compound 3h was investigated by the graphical analysis of steady-state inhibition data. Reciprocal plots (Lineweaver–Burk plots) describing 3h inhibition showed both increasing slopes and increasing intercepts with higher inhibitor concentration. The pattern indicated the mixed-type inhibition which was similar to that of rutaecarpine derivatives. The result revealed that compound 3h was able to bind both of the active site and PAS of AChE, which was also in agreement with the results of molecular modelling studies.
Molecular modelling
With the aim of obtaining useful information about the binding interactions between the synthetic compounds and TcAChE (PDB code: 1EVE), molecular modelling study was carried out using docking program AUTODOCK 4.0 package with PyMOL programCitation26,Citation27, and the result is shown in . The docking studies indicated that all of these compounds exhibited multiple-binding modes with AChE. In the complex of TcAChE-3h, compound 3h occupied the PAS and gorge. The quinazoline moiety was bound to PAS with a classic π−π stacking interaction with Trp279 and the ring-to-ring distance was 3.6 Å, and the protonated nitrogen atom of the indolyl ring had a hydrogen bond (2.2 Å) interaction with the carbonyl group of Ile 287. The conformation of the side chain made good fit with the shape of the gorge, in the mid-gorge, a hydrogen bond was observed between the carbonyl group of the substituted side chains and Tyr121 with a distance of 2.1 Å, and in the bottom of the gorge, the charged nitrogen of piperidine also made a hydrogen bond interaction with the Asp 72 and the distance was 1.8 Å. The simultaneous interactions of 3h in the peripheral pocket and catalytic triad side of TcAChE explained the higher inhibitory potency of AChE; however, different interaction mode were found in 3h in complex with HuBuChE (PDB code: 1POI). A π−π stacking interaction was found between indolyl moiety and Trp82, while the distance was 4.5 Å, and a hydrogen bond was observed between the charged nitrogen of piperidine of the side chain and Pro285 with a distance of 2.2 Å. As a result, the compound gave weaker inhibitory activity for BuChE and exhibited higher selectivity for AChE/BuChE. The docking studies showed that the compounds had a mixed type of inhibition, which was consistent with our kinetic analysis result. In the complexes of TcAChE-9a and HuBuChE-9a, compound 9a with a wide shape impaired the entrance into the AChE and BuChE gorge and cannot interact with the internal residue at the catalytic site. These results may explain why compounds 9a, 9b show little inhibitory activity towards ChE.
Figure 4. (Colour online) Docking models of compound–enzyme complex. Representations of compound 3h (A, B) and 9a (C, D) interacting with residues in the binding site of TcAChE (A, C) and HuBuChE (B, D). The compounds are rendered in green stick models, and the residues are rendered in golden sticks. Pictures are created with PyMOLCitation27.
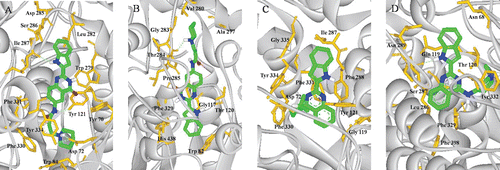
Conclusions
In conclusion, a structure-based drug design allowed us to synthesize a series of novel 2-(2-Indolyl-)-4(3H)-quinazolines derivates as the ring-opened analogues of rutaecarpine alkaloid and subjected to pharmacological evaluation as AChE inhibitors. Among them, derivates 3a–d and 3f–i exhibited strong inhibitory activity for AChE and high selectivity for AChE over BuChE. The SARs were discussed and their binding conformation and simultaneous interactions mode were further clarified by kinetic characterization and the molecular docking studies. Finally, the results indicate that these new compounds could be considered as a new lead for further optimization.
Acknowledgement
Zeng Lia and Bin Wang have equally contributed to this paper.
Declaration of interest
The authors report no conflicts of interest. The authors alone are responsible for the content and writing of the paper. This study was financially supported by the Natural Science Foundation of China (Grants U0832005, 21172272), the International S&T Cooperation Program of China (2010DFA34630) and the Science Foundation of Guangzhou (2009A1-E011-6).
References
- Davies P, Maloney AJ. Selective loss of central cholinergic neurons in Alzheimer’s disease. Lancet 1976;2:1403.
- Bartus RT, Dean RL 3rd, Beer B, Lippa AS. The cholinergic hypothesis of geriatric memory dysfunction. Science 1982;217:408–414.
- Talesa VN. Acetylcholinesterase in Alzheimer’s disease. Mech Ageing Dev 2001;122:1961–1969.
- Medications for Alzheimer’s disease. Benefits of currently approved drugs are limited, but research continues. Harv Ment Health Lett 2011; 27: 4–5.
- Doody RS, Cummings JL, Farlow MR. Reviewing the Role of Donepezil in the Treatment of Alzheimer’s Disease. Curr Alzheimer Res 2011; DOI: 10.2174/1567212221756532050
- Weintraub D, Somogyi M, Meng X. Rivastigmine in Alzheimer’s disease and Parkinson’s disease dementia: an ADAS-cog factor analysis. Am J Alzheimers Dis Other Demen 2011;26:443–449.
- Wallin AK, Wattmo C, Minthon L. Galantamine treatment in Alzheimer’s disease: response and long-term outcome in a routine clinical setting. Neuropsychiatr Dis Treat 2011;7:565–576.
- Bosak A, Katalinic M, Kovarik Z. [Cholinesterases: structure, role, and inhibition]. Arh Hig Rada Toksikol 2011;62:175–190.
- Scarpini E, Scheltens P, Feldman H. Treatment of Alzheimer’s disease: current status and new perspectives. Lancet Neurol 2003;2:539–547.
- Liu A, Guang H, Zhu L, Du G, Lee SM, Wang Y. 3D-QSAR analysis of a new type of acetylcholinesterase inhibitors. Sci China, C, Life Sci 2007;50:726–730.
- Akula N, Lecanu L, Greeson J, Papadopoulos V. 3D QSAR studies of AChE inhibitors based on molecular docking scores and CoMFA. Bioorg Med Chem Lett 2006;16:6277–6280.
- De Ferrari GV, Canales MA, Shin I, Weiner LM, Silman I, Inestrosa NC. A structural motif of acetylcholinesterase that promotes amyloid β-peptide fibril formation. Biochemistry 2001;40:10447–10457.
- Bartolini M, Bertucci C, Cavrini V, Andrisano V. β-Amyloid aggregation induced by human acetylcholinesterase: inhibition studies. Biochem Pharmacol 2003;65:407–416.
- Mesulam MM, Asuncion Morán M. Cholinesterases within neurofibrillary tangles related to age and Alzheimer’s disease. Ann Neurol 1987;22:223–228.
- Cummings JL, Doody R, Clark C. Disease-modifying therapies for Alzheimer disease: challenges to early intervention. Neurology 2007;69:1622–1634.
- Liston DR, Nielsen JA, Villalobos A, Chapin D, Jones SB, Hubbard ST et al. Pharmacology of selective acetylcholinesterase inhibitors: implications for use in Alzheimer’s disease. Eur J Pharmacol 2004;486:9–17.
- Cerbai F, Giovannini MG, Melani C, Enz A, Pepeu G. N1phenethyl-norcymserine, a selective butyrylcholinesterase inhibitor, increases acetylcholine release in rat cerebral cortex: a comparison with donepezil and rivastigmine. Eur J Pharmacol 2007;572:142–150.
- Rydberg EH, Brumshtein B, Greenblatt HM, Wong DM, Shaya D, Williams LD et al. Complexes of alkylene-linked tacrine dimers with Torpedo californica acetylcholinesterase: Binding of Bis5-tacrine produces a dramatic rearrangement in the active-site gorge. J Med Chem 2006;49:5491–5500.
- Cavalli A, Bolognesi ML, Minarini A, Rosini M, Tumiatti V, Recanatini M et al. Multi-target-directed ligands to combat neurodegenerative diseases. J Med Chem 2008;51:347–372.
- Cavalli A, Bolognesi ML, Capsoni S, Andrisano V, Bartolini M, Margotti E et al. A small molecule targeting the multifactorial nature of Alzheimer’s disease. Angew Chem Int Ed Engl 2007;46:3689–3692.
- Skovronsky DM, Lee VM, Trojanowski JQ. Neurodegenerative diseases: new concepts of pathogenesis and their therapeutic implications. Annu Rev Pathol 2006;1:151–170.
- Wang B, Mai YC, Li Y, Hou JQ, Huang SL, Ou TM et al. Synthesis and evaluation of novel rutaecarpine derivatives and related alkaloids derivatives as selective acetylcholinesterase inhibitors. Eur J Med Chem 2010;45:1415–1423.
- Hermecz I, Kökösi J, Podányi B, Szász G. Synthesis of Indolyl-4(3H)-Quinazolinones. Heterocycles 1994;37:903–914.
- Ellman GL, Courtney KD, Andres V Jr, Feather-Stone RM. A new and rapid colorimetric determination of acetylcholinesterase activity. Biochem Pharmacol 1961;7:88–95.
- Decker M. Novel inhibitors of acetyl- and butyrylcholinesterase derived from the alkaloids dehydroevodiamine and rutaecarpine. Eur J Med Chem 2005;40:305–313.
- Morris GM, Goodsell DS, Halliday RS, Huey R, Hart WE, Belew RK and Olson AJ. Automated docking using a lamarckian genetic algorithm and empirical binding free energy function. J Comput Chem 1998;19:1639–1662.
- Seeliger D, de Groot BL. Ligand docking and binding site analysis with PyMOL and Autodock/Vina. J Comput Aided Mol Des 2010;24:417–422.