Abstract
Alkaloid fractions of Psychotria suterella (SAE) and Psychotria laciniata (LAE) as well as two monoterpene indole alkaloids (MIAs) isolated from these fractions were evaluated against monoamine oxidases (MAO-A and -B) obtained from rat brain mitochondria. SAE and LAE were analysed by HPLC-PDA and UHPLC/HR-TOF-MS leading to the identification of the compounds 1, 2, 3 and 4, whose identity was confirmed by NMR analyses. Furthermore, SAE and LAE were submitted to the enzymatic assays, showing a strong activity against MAO-A, characterized by IC50 values of 1.37 ± 1.05 and 2.02 ± 1.08 μg/mL, respectively. Both extracts were also able to inhibit MAO-B, but in higher concentrations. In a next step, SAE and LAE were fractionated by RP-MPLC affording three and four major fractions, respectively. The RP-MPLC fractions were subsequently tested against MAO-A and -B. The RP-MPLC fractions SAE-F3 and LAE-F4 displayed a strong inhibition against MAO-A with IC50 values of 0.57 ± 1.12 and 1.05 ± 1.15 μg/mL, respectively. The MIAs 1 and 2 also inhibited MAO-A (IC50 of 50.04 ± 1.09 and 132.5 ± 1.33 μg/mL, respectively) and -B (IC50 of 306.6 ± 1.40 and 162.8 ± 1.26 μg/mL, respectively), but in higher concentrations when compared with the fractions. This is the first work describing the effects of MIAs found in neotropical species of Psychotria on MAO activity. The results suggest that species belonging to this genus could consist of an interesting source in the search for new MAO inhibitors.
Introduction
Psychotria L. (Rubiaceae) is a taxonomically complex genus whose neotropical species are able to synthesize glucoside monoterpene indole alkaloids (MIAs) with significant biological and pharmacological interest.Citation1–3 The chemical investigation of Brazilian Southern species allowed the isolation of MIAs in P. suterellaCitation4, P. brachycerasCitation5, P. leiocarpaCitation6, P. myrianthaCitation7, P. umbellataCitation8 and P. nudaCitation9. Additionally, MIAs are also found in neotropical Psychotria from Central AmericaCitation10, and Brazilian NortheastCitation11,Citation12 and MidwestCitation13. Most of these alkaloids, except those found in P. brachyceras and P. umbellata, are derived from the common precursor strictosidine, and, therefore, they can present βC, DHβC or THβC nuclei in their indole portionsCitation1,Citation3.
MIAs of Psychotria have been submitted to biological and pharmacological investigations revealing a significant number of activities, mainly related with the central nervous system (CNS). Psychollatine, the major alkaloid found in P. umbellata leaves, displayed mild analgesic activity and anxiolytic, antidepressive and amnesic effects in mice models. Their effects seem to be related to the modulation of the opioid, glutamatergic NMDA and serotonergic 5-hydroxytryptamine (5-HT)2A/C systemsCitation14–16. Strictosidinic acid showed to be able to inhibit the polymorphonuclear leukocytes chemotaxis in vitroCitation7, and it has shown peripheral analgesic and antipyretic activities in mice after oral administrationCitation17. In addition, this compound seems to act on 5-HT and DA systems in rat striatum, affecting the monoamines metabolism in this brain areaCitation18.
Monoamine oxidases (MAOs) are mitochondrial outer membrane-bound flavoenzymes that catalyse the oxidative deamination of several important neurotransmitters, including 5-HT (or serotonin), histamine and the catecholamines dopamine, norepinephrine and epinephrineCitation19. Two subtypes of MAO, MAO-A and MAO-B are similar in their primary sequences but have different substrate and inhibitor affinities. MAO-A is inhibited by low concentrations of clorgyline and catalyses the oxidation of 5-HT, whereas MAO-B is inhibited by low concentrations of l-deprenyl or pargyline and is active towards benzylamine and 2-phenylethylamine. Dopamine, norepinephrine, tryptamine and tyramine are oxidized by both forms of the enzyme in most speciesCitation20,Citation21. MAO-A inhibitors have proven to be effective in the pharmacological treatment of depression, and further developments have provided reversible inhibitors of MAO-A, which offer antidepressant activity without the serious side effects of the earlier inhibitors. On the other hand, selective inhibitors of MAO-B have found a therapeutic role in the treatment of Parkinson’s diseaseCitation22.
βC, DHβC and THβC alkaloids, such as harmane, harmine, harmaline and tetrahydroharmine, are potent and reversible inhibitors of MAO-ACitation23,Citation24. They also possess some degree of activity against MAO-B. Therefore, considering the presence of THβCs and βCs compounds in Psychotria, and the previous effects described for these alkaloids on the CNS, it becomes relevant to investigate the effects of fractions and MIAs of Psychotria on MAO-A and -B activity. Thus, the aim of the present work was to evaluate Psychotria suterella Müll. Arg. and P. laciniata Vell. alkaloid fractions and MIAs against monoamine oxidases (MAO-A and B) obtained from rat brain mitochondria.
Methods
General experimental procedures
Experiments by HPLC were performed in a system composed by a Model 2695 Waters Alliance analytical module equipped with a 996 photodiode array detector and a computerized data station equipped with Waters Empower software (Waters, Milford, MA), using a Luna C18 100 Å column (Phenomenex, 250 mm × 4.6 mm I.D.; 5 µM particle size; Phenomenex Inc., Torrance, CA, USA) and operated at ambient temperature. The column was equipped with a 2-cm LC-18 guard column (Phenomenex Inc.). Reversed-phase medium pressure liquid chromatography (RP-MPLC) was carried out on a BUCHI system equipped with a B-688 BUCHI chromatography pump, a B-687 BUCHI gradient former and a BUCHI borosilicate column filled out with RP-18 LiChrospher® (Merck, Darmstadt, Germany). Centrifugal preparative TLC (CPTLC) was performed by a Chromatotron® (Harrison Reasearch Inc.) in a 2-mm silicagel rotor (Merck, Darmstadt, Germany; SiO2 coated with F254 indicator). Experiments by gas chromatography were performed in a GC System Agilent Technologies 7890A equipped with a Triple-Axis Detector 5975C and a fused silica capillary column (30 m, 0.25 mm, 0.25 μm, coated with HP-5). The temperature was programmed from 80 (hold 3 min) to 250°C (hold 5 min) at 15°C/min and then to 320°C at 15°C/min (hold 5 min). Injector and detector temperatures were both set at 300°C. The GC apparatus was equipped with quadrupole detector (Triple-Axis Detector 5975C, Agilent Technologies) operating at 70 eV and mass range 40–600 a.m.u. (split 1:20). Helium was used as gas carrier at a flow of 1 mL/min. The injection volume was 1 µL. UHPLC/HR-TOF-MS analyses were performed on a Micromass-LCT Premier Time of Flight mass spectrometer from Waters (Milford, MA, USA) with an electrospray (ESI) interface coupled with an Acquity UPLC system from Waters. Detection was performed in positive ion mode in the range m/z 100–1000 in centred mode with a scan time of 0.2 s and an interscan delay of 0.3 s for polarity switching. ESI conditions were capillary voltage 2800 V, cone voltage 40 V, source temperature 120°C, desolvation temperature 250°C, cone gas flow 20 L/h and desolvation gas flow 800 L/h. For internal calibration, a solution of leucine/enkephalin from Sigma-Aldrich (Steinheim, Germany) at 5 μg/mL was infused through the lockmass probe at a flow rate of 5 μL/min, using a second Shimadzu LC-10ADvp LC pump (Duisburg, Germany). The separation was performed on a 150 mm × 2.1 mm i.d., 1.7 μm, Acquity BEH C18 UPLC column (Waters) in the gradient mode at a flow rate of 0.3 mL/min with the following solvent system: (A) 0.1% formic acid—water and (B) 0.1% formic acid—acetonitrile; in 7.0 min. The temperature was set at 30°C. The injected volume was 5 μL. The Citation1H NMR spectra were recorded on a Varian Inova 500 MHz spectrometer (Palo Alto, CA, USA) at 499.87 MHz. The instrument was controlled by Varian VNMR software installed on a Sun workstation (Santa Clara, CA, USA). All NMR measurements were performed in d6-dimethyl sulfoxide (DMSO; Dr Glaser, AG, Basel, Switzerland) at 25°C. Fractions were concentrated on an R-114 BUCHI rotavapor equipped with a V-710 BUCHI vacuum pump. Fluorescence readings were performed on a microplate reader 2104 Perkin-Elmer EnVision®. The mitochondrial fractions were centrifuged on a CR22GII Hitachi Himac centrifuge.
Chemicals
Kynuramine dihydrobromide, pargyline hydrochloride, clorgyline hydrochloride, 4-hydroxyquinoline (4-OH), DMSO, bovine albumin (BSA), HEPES and D-mannitol were purchased from Sigma Chemical Co. (St. Louis, MO, USA). Sucrose, potassium chloride, sodium chloride, diethyl ether and methylene chloride were acquired from Labsynth (Diadema, SP, Brazil). Sodium phosphate monobasic monohydrate, sodium phosphate dibasic dodecahydrate and acetonitrile HPLC grade were purchased from Merck (Darmstadt, Germany). All remaining chemicals used were of analytical grade and were purchased from F. Maia (Cotia, SP, Brazil). Stock solutions of kynuramine, pargyline, clorgyline and 4-OH were prepared in phosphate-buffered solution (PBS) buffer (pH 7.4) and maintained at −20°C for up to 6 months.
Plant material
Leaves of Psychotria suterella Müll. Arg. and P. laciniata Vell. (Rubiaceae) were collected from the Atlantic forest of Dom Pedro de Alcântara (Rio Grande do Sul State, Brazil, S29°23′; W49°50′; ICN 189938) and Cocal do Sul (Santa Catarina State, Brazil S26°80′; W48°95′; ICN 182552), respectively. The material was identified by Dr. Sergio L. Bordignon (Fundação La Salle, RS, Brazil), and the voucher specimens have been deposited in the herbarium of Departamento de Botânica from Universidade Federal do Rio Grande do Sul (UFRGS).
Extraction of plant material
Air-dried and powdered leaves of P. suterella (304 g) and P. laciniata (410 g) were extracted with ethyl alcohol (5 × 48 h) at room temperature. The solvent was removed under vacuum, and the resulting dark green syrups were dissolved in 1 N HCl (0.6 L) and exhaustively extracted with methylene chloride to remove the nonpolar constituents. Subsequently, the acid extracts were alkalinized with 25% NH4OH (pH 9–10) and partitioned with diethyl ether (EtOEt) and methylene chloride (CH2Cl2) until the Mayer reaction for alkaloids was negative. After comparison by TLC and HPLC-PDA, the fraction CH2Cl2 was added to the fraction EtOEt, affording the alkaloid fractions: P. suterella (SAE; 0.77 g, 0.25% w/w) and P. laciniata (LAE; 0.98 g, 0.24% w/w). The chromatograms resulting from the analysis of SAE and LAE by HPLC-PDA are shown in . The results obtained from the UHPLC/HR-TOF-MS analyses made for SAE and LAE are summarized in . In a next step, SAE and LAE were fractionated by RP-MPLC with a water–acetonitrile gradient step to result three major fractions for SAE and four major fractions for LAE: SAE-F1 (250 mg), SAE-F2 (81 mg) and SAE-F3 (128 mg), for P. suterella SAE; and LAE-F1 (211 mg), LAE-F2 (35 mg), LAE-F3 (92 mg) and LAE-F4 (136 mg) for P. laciniata LAE (Table S1 and ). SAE-F2 and LAE-F3 were submitted to MPLC with water–acetonitrile (70:30), affording strictosamide (2, 22 and 38 mg, respectively) (). Finally, SAE-F3 (50 mg) was submitted to CPTLC (Chromatotron®) using 2-mm silicagel rotor with pentane–diethyl ether (1:1) as solvent, affording three main fractions containing mixtures of vallesiachotamine (3) and isovallesiachotamine (4) in different proportions (; Figure S1): F23–F28 (3.2 mg of a mixture containing 20% of 3 and 80% of 4), F29–F35 (8.5 mg of a mixture containing 45% of 3 and 55% of 4) and F36–F44 (5.3 mg of a mixture containing 95% of 3 and 5% of 4). The identity of these two alkaloids was further confirmed by HRMS and Citation1D and Citation2D NMR (Table S2).
Table 1. Description of the main peaks observed in the HPLC–PDA and UHPLC/HR–TOF–MS analyses performed for SAE and LAE.
Figure 1. Chromatograms and UV spectra obtained by LC-PDA for the alkaloids fractions of P. suterella (SAE) and P. laciniata (LAE). Peak 1: UV spectrum characteristic for βC nucleus (further characterized as 1 by co-injection with the isolated standard and by UHPLC/HRTOF-MS and NMR analyses). Peak 2: UV spectrum characteristic of βC nucleus (further characterized as a possible 1 derivative by UHPLC/HR-TOF-MS analysesCitation25). Peak 3: UV spectrum characteristic of THβC MIAs (further characterized as 2 by co-injection with the isolated standard and by UHPLC/HR-TOF-MS and NMR analyses). Peaks 4 and 5: UV spectra characteristic of compounds possessing vallesiachotamine-like nucleus (further characterized as 3 and 4 by GC-MS, UHPLC/HR-TOF-MS and NMR analyses).
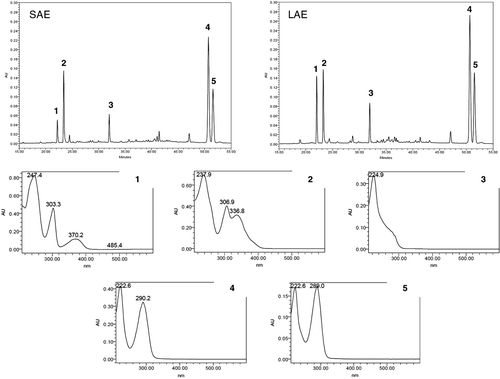
Animals
Male Wistar rats (120-days-old, 300–400 g) that came from the local breeding colony (ICBS–UFRGS) were housed for 1 week in our animal facility under controlled environmental conditions (22°C ± 1°C, 12 h light/dark cycle, free access to food [commercial standard rodent cube diet Nuvilab, CR1] and water), until the beginning of experiments. All animal experiments were carried out in accordance with the guidelines for care, and use of experimental animals contained in the National Research Council Guide for the Care and Use of Laboratory Animals. The project was approved (#17247, 2010) by the Ethics Committee of Federal University of Rio Grande do Sul (CEUA–UFRGS).
Preparation of brain mitochondria
Animals were killed by decapitation. Brains were immediately removed and washed in ice-cold isolation medium with the following composition (in mM): 10 HEPES; 68 sucrose; 10 KCl; 220 D-mannitol; and 0.1% BSA (pH 7.4). Brains tissues were mechanically homogenized in a Potter–Elvehjem tissue grinder in 10 vol of the ice-cold buffer. Then the tissue homogenates were centrifuged at 3000 × g for 10 min at 4°C, and the supernatant was centrifuged at 11,500 × g for 15 min at 4°C. After that, the supernatant was removed, and the resulting pellet was suspended in 3 mL of PBS (pH 7.4) and stored at −80°C. Protein concentrations were determined by Peterson’s modification of the procedure proposed by Lowry and coworkersCitation26.
MAO inhibition assay
Monoamine oxidase (MAO) inhibition assays were conducted with a fluorescence method based on endpoint reading, using kynuramine as nonselective substrate for monoamine oxidases (MAO-A and -B). The incubations were performed according to previously reportedCitation27, with minor modifications. Briefly, assays were carried out in black polystyrene 96-well microtiter plates in a final volume of 200 µL. The concentrations of kynuramine and protein were determined by preliminary experiments, in which 12 different concentrations of substrate (ranging from 2.5 to 200 µM; concentration choose: kynuramine 50 µM) and 12 protein concentrations (ranging from 0.025 to 0.3 mg/mL; concentration choose: protein 0.1 mg/mL in the mitochondrial fractions) were evaluated.
For the MAO-A inhibition assays, the wells containing 120 µL of PBS (pH 7.4), 5 µL of pargyline 10 µM (to get a final concentration of 250 nM), 25 µL of the sample solution prepared in PBS and DMSO (to get a final concentration of 1% DMSO) and 40 µL of the mitochondrial suspension (to get a final protein concentration of 0.1 mg/mL) were preincubated at 37°C for 30 min. The MAO-B inhibition assays were performed in the same way that the MAO-A incubations, except by the use of 5 µL of clorgyline 10 µM to replace the pargyline solution. As positive control for MAO activity (without inhibition), 5 µL of a 40% DMSO solution (in PBS) was used in place of the inhibitor, in the absence of samples. As positive control for MAO-A and MAO-B activities, 5 µL of a 40% DMSO solution (in PBS) and 5 µL of pargyline 10 µM (or clorgyline 10 µM) were used in the absence of the samples.
Fractions and alkaloids were tested in concentrations ranging from 0.1 to 500 µg/mL. For each sample, 8–10 different concentrations were evaluated in duplicate. Data analysis was performed with Prism 5.0 (GraphPad Software, Inc., CA, USA). The degree of inhibition IC50 was assessed by adjusting the experimental data (% inhibition versus concentration of inhibitor) to nonlinear regression curves.
Results and discussion
The alkaloid fractions of P. suterella and P. laciniata evaluated in this study showed to be able to inhibit the enzymes MAO-A and MAO-B obtained from rat brain mitochondria. The IC50 values calculated for SAE (1.37 ± 1.05 and 55.44 ± 1.05 μg/mL for MAO-A and B, respectively) and LAE (2.02 ± 1.08 and 61.15 ± 1.06 μg/mL for MAO-A and B, respectively) indicated high potency against MAO-A and some degree of selectivity for this isoform (Figure S2 and and ). Regarding the activity against MAO-A, the tested extracts displayed maximum inhibition above 95% in the highest concentrations evaluated. Additionally, in the sigmoidal dose-response curve of SAE for MAO-A inhibition, the Hill coefficient in the concentration-response equation is near to the unit, indicating an ideal behavior and suggesting a 1-to-1 binging between inhibitor and enzymeCitation28. On the other hand, the Hill coefficients calculated for the curves of SAE against MAO-B and LAE against MAO-A and B were greater than unit, suggesting a nonspecific mechanism of inhibition.
Table 2. MAO-A inhibitory activity of fractions and monoterpene indole alkaloids from P. suterella and P. laciniata.
Table 3. MAO-B inhibitory activity of fractions and monoterpene indole alkaloids from P. suterella and P. laciniata.
The HPLC-PDA analyses indicated that SAE and LAE present five major compounds ( and ). The UV spectra displayed by the peaks referents to these compounds are consistent with βC, THβC4 and vallesiachotamine-like nucleiCitation29. The presence of the alkaloids 1 and 2 in SAE and LAE was further confirmed by coinjection, in HPLC-PDA, with the pure compounds isolated from P. suterella4. Moreover, UHPLC/HR-TOF-MS analyses were performed comparing the mass spectra and the retention times with those displayed by 1 and 2 (). In addition, high-resolution MS spectra of the peaks in 1.15 (SAE) and 1.16 min (LAE) provided pseudo-molecular ions at m/z 733.2567 (SAE) and 733.2577 (LAE), calculated for C38H41N2O13 [M + H]+ (733.2609), probably displayed by an 1 derivative: (E)-O-(6′)-cinnamoyl-40-hydroxy-30,50-dimethoxy-lyalosideCitation25. The HRMS spectra of the peaks in 2.31 min (SAE and LAE) provided pseudo-molecular ions at m/z 351.1696 (SAE) and 351.1697 (LAE), calculated for C21H23N2O3 [M + H]+ (351.1709), corresponding to vallesiachotamine/isovallesiachotamine (3 and 4).
Despite the apparent morphological similarity, P. suterella and P. laciniata remain segregatedCitation30 based on single characters, such as the size of their corolla. In the present work, these two species, collected from different sites in the Brazilian States of Rio Grande do Sul e Santa Catarina, showed very similar chemical content suggesting a close relationship between them.
The results displayed by SAE and LAE against MAO-A and -B could be attributed to the substances possessing βC and THβC nuclei, because that βC, DHβC and THβC alkaloids are well-known MAO-A inhibitorsCitation23,Citation24. Therefore, a bioassay-guided fractionation of SAE and LAE by RP-MPLC was undertaken in order to identify the active groups. The fractionation of SAE afforded three major fractions: SAE-F1, SAE-F2 and SAE-F3 ( and Table S1). SAE-F1 was characterized by the presence of two major peaks presenting UV spectra characteristics of βC, attributed to lyaloside (1) () and to a 1 derivative. In the UHPLC/HR-TOF-MS analyses, SAE-F1 presented two peaks: one in 1.08 min (pseudo-molecular ion at m/z 527.2023) and other in 1.15 min (pseudo-molecular ion at 733.2631), corresponding to 1 and 1 derivative (Table S1)Citation25. SAE-F2 presented one major peak in HPLC-PDA chromatogram displaying the characteristic UV spectrum of THβC MIAs. In the UHPLC-MS experiment, SAE-F2 displayed three peaks, corresponding to 1, 1 derivative and 2 (Table S1). The third fraction, SAE-F3, showed two major peaks in HPLC-PDA chromatogram presenting the typical chromophores of vallesiachotamine-like alkaloids (high absortivity in 290 nm region, associated with the β-N-acrylate group). The UHPLC-MS analyses displayed a peak in 2.32 min (pseudo-molecular ion at m/z 351.1702) corresponding to a mixture of 3 and 4 (Table S1).
The fractionation of LAE afforded four major fractions: LAE-F1, LAE-F2, LAE-F3 and LAE-F4 ( and Table S1). LAE-F2 presented one major peak in HPLC-PDA chromatogram corresponding to 1 derivative. In the UHPLC-MS analyses, LAE-F1 presented two peaks in 1.07 and 1.16 min corresponding to 1 and 1 derivativeCitation25, respectively (Table S1). On the other hand, LAE-F1, LAE-F3 and LAE-F4 showed chromatographic profiles in HPLC-FDA and UHPLC/HR-TOF-MS analyses similar to those displayed for SAE-F1, SAE-F2 and SAE-F3, respectively (Table S1).
The fractions of SAE and LAE obtained by RP-MPLC and the alkaloids lyaloside (1) and strictosamide (2) were tested against the enzymes MAO-A and -B from rat brain mitochondria (Figure S2 and and ). The fractions SAE-F3 and LAE-F4 showed high potency against MAO-A, presenting IC50 values of 0.57 ± 1.12 and 1.05 ± 1.15 μg/mL, respectively. As well as was observed for SAE and LAE, these two fractions exhibited some degree of selectivity for MAO-A. Moreover, both extracts were able to inhibit MAO-A and MAO-B in more than 90% in the highest concentrations tested, and the sigmoidal curves displayed Hill coefficients near to the unit.
In a subsequent step, SAE-F3 and LAE-F4 were analyzed by GC-MS aiming to identify their main alkaloids. In addition, SAE-F3 was fractionated by CPTLC in order to isolate the compounds 3 and 4. In the GC–MS analyses, both fractions showed peaks in 26 min displaying the mass spectrum characteristic for vallesiachotamine-like nucleiCitation31. The mass spectrum showed a strong molecular ion at m/z 350. Fragments for the loss of CH3, CH3O and CHO were also observed. The major fragments at m/z 291, 279, 221 and 263 are diagnostic for the alkaloids 3 and 4Citation29,Citation31,Citation32. Three main fractions corresponding to mixtures of 3 and 4 in different proportions were obtained from CPTLC. 1D and 2D NMR experiments confirmed the identity of 3 and 4 and also their relative proportion in the mixtures (Table S2 and Figure S1). Vallesiachotamine and isovallesiachotamine were previously described in Psychotria bahiensisCitation10 and in two other species belonging to Rubiaceae family: Palicourea rigidaCitation33 and Chimarrhis turbinataCitation34.
The other fractions tested against MAO-A and B also showed important inhibition on MAO-A (Figure S2 and and ). All of them were able to inhibit this isoform, displaying IC50 values ranging from 2 to 22 μg/mL, maximum inhibition above 95%, and Hill coefficients near the unit. For MAO-B, these fraction displayed a weak activity characterized by IC50 values ranging from 55 to 240 μg/mL and maximum inhibition above 70%. Despite the weak activities, the Hill coefficients calculated for MAO-B inhibition presented values near the unit, except for LAE-F3, whose Hill coefficient was above the unit. The alkaloids lyaloside (1) and strictosamide (2) showed weak activity against MAO-A and B when compared with the tested fractions (Figure 2S and and ).
This is the first study reporting the inhibitory activity of fractions and MIAs from neotropical species of Psychotria on the enzymes MAO-A and B. These data are in agreement with the presence of compounds possessing βC and THβC nuclei in P. suterella and P. laciniata. A great number of studies has been demonstrated the effect of βC alkaloids on MAO-A and B activitiesCitation23,Citation35–37. Harmaline and harmine seem to behave as potent and selective inhibitors of MAO A, being approximately 10,000-fold more potent for human recombinant MAO-A than for MAO-B.
The elevated IC50 values obtained for the MIAs lyaloside (MAO-A IC50 = 50.0 µg/mL, corresponding to 117.5 µM; MAO-B IC50 = 306.6 µg/mL, corresponding to 724.4 µM) and strictosamide (MAO-A IC50 = 132.5 µg/mL, corresponding to 275.4 µM; MAO-B IC50 = 162.8 µg/mL, corresponding to 645.5 µM) could be explained by some structural features, such as the glucose moiety attached at the monoterpene substituent. Studies carried out with flavonoids and anthocyanins have been demonstrated that compounds possessing sugar substituent are less potent inhibitors of MAO-A and -B when compared with their respective aglyconesCitation38,Citation39. Moreover, both alkaloids possess voluminous groups near their pyridine (in lyaloside) and piperidine (in strictosamide) nitrogens, which could hinder the free electrons pair. On the contrary, compounds 3 and 4 present neither glucose moiety nor voluminous groups hindering the free electrons pair of piperidine. These structural characteristics, together with the MAO-A and -B inhibition observed for fractions containing 3 and 4 as major compounds, might suggest that vallesiachotamine and isovallesiachotamine are able to inhibit both enzymes obtained from rat brain mitochondria.
Supplementary Material
Download PDF (1.1 MB)Acknowledgments
Authors acknowledge Professors Muriel Cuendet, Pierre-Alain Carrupt and Jean-Luc Wolfender from the University of Geneva for receiving C.S.P. in their laboratories and for making NMR and MS analyses available. Authors are also thankful to Dr. Sergio Bordignon for the identification of the species employed in this work.
Declaration of interest
C.S.P. acknowledges the fellowships from CNPq/Brazil and CAPES/Brazil (for financial support during her internship at the University of Geneva). T.C.S is recipient of a fellowship from CNPq (BIC/UFRGS). This work was supported by CNPq, grant no. 472287/2009-5.
References
- Lopes S, Von Poser GL, Kerber VA, Farias FM, Konrath EL, Moreno P, et al. Taxonomic significance of alkaloids and iridoid glucosides in the tribe Psychotrieae (Rubiacea). Biochem Syst Ecol 2004;32:1187–1195.
- Pasquali G, Porto DD, Fett-Neto AG. Metabolic engineering of cell cultures versus whole plant complexity in production of bioactive monoterpene indole alkaloids: recent progress related to old dilemma. J Biosci Bioeng 2006;101:287–296.
- Porto DD, Henriques AT, Fett-Neto AG. Bioactive alkaloids from South American Psychotria and related species. Open Bioact Compound J 2009;2:29–36.
- De Santos LV, Fett-Neto AG, Kerber VA, Elisabetsky E, Quirion JC, Henriques AT. Indole monoterpene alkaloids from the leaves of Psychotria suterella Müll. Arg. (Rubiaceae). Biochem Syst Ecol 2001;29:1185–1187.
- Kerber VA, Gregianini TS, Paranhos JT, Schwambach J, Farias FM, Fett JP, et al. Brachycerine, a novel monoterpene indole alkaloid from Psychotria brachyceras. J Nat Prod 2001;64:677–679.
- Henriques AT, Lopes SO, Paranhos JT, Gregianini TS, Von Poser GL, Fett-Neto AG et al. N,beta-D-Glucopyranosyl vincosamide, a light regulated indole alkaloid from the shoots of Psychotria leiocarpa. Phytochemistry 2004;65:449–454.
- Simões-Pires CA, Farias FM, Marston A, Queiroz EF, Chaves CG, Henriques AT, et al. Indole monoterpenes with antichemotactic activity from Psychotria myriantha. Nat Prod Commun 2006;1: 1101–1106.
- Kerber VA, Passos CS, Verli H, Fett-Neto AG, Quirion JP, Henriques AT. Psychollatine, a glucosidic monoterpene indole alkaloid from Psychotria umbellata. J Nat Prod 2008;71:697–700.
- Farias FM, Konrath EL, Zuanazzi JAS, Henriques AT. Strictosamide from Psychotria nuda (Cham. et Schltdl) Wawra (Rubiaceae). Biochem Syst Ecol 2008;36:919–920.
- Paul JH, Maxwell AR, Reynolds WF. Novel bis(monoterpenoid) indole alkaloids from Psychotria bahiensis. J Nat Prod 2003;66: 752–754.
- Pimenta AAT, Braz-Filho R, Delprete PG, Souza EB, Silveira ER, Lima MAS. Unusual monoterpene indole alkaloids from Psychotria stachyoides Benth. Biochem Syst Ecol 2010;38:846–849.
- Pimenta AT, Braz-Filho R, Delprete PG, de Souza EB, Silveira ER, Lima MA. Structure elucidation and NMR assignments of two unusual monoterpene indole alkaloids from Psychotria stachyoides. Magn Reson Chem 2010;48:734–737.
- Faria EO, Kato L, De Oliveira CMA, Carvalho BG, Silva CC, Sales LS, et al. Quaternary β-carboline alkaloids from Psychotria prunifolia (Kunth) Steyerm. Phytochem Lett 2010;3:113–116.
- Both FL, Kerber VA, Henriques AT, Elisabetsky E. Analgesic properties of umbellatine from Psychotria umbellata. Pharm Biol 2002;40:336–341.
- Both FL, Meneghini L, Kerber VA, Henriques AT, Elisabetsky E. Psychopharmacological profile of the alkaloid psychollatine as a 5HT2A/C serotonin modulator. J Nat Prod 2005;68:374–380.
- Both FL, Meneghini L, Kerber VA, Henriques AT, Elisabetsky E. Role of glutamate and dopamine receptors in the psychopharmacological profile of the indole alkaloid psychollatine. J Nat Prod 2006;69:342–345.
- Reanmongkol W, Subhadhirasakul S, Kongsang J, Tanchong M, Kitti J. Analgesic and Antipyretic Activities of n-Butanol Alkaloids Extracted from the Stem Bark Hunteria Zeylanica and its Major Constituent, Strictosidinic Acid, in Mice. Pharm Biol 2000;38:68–73.
- Farias FM, Passos CS, Arbo MD, Zuanazzi JA, Steffen VM, Henriques AT. Monoamine levels in rat striatum after acute intraperitoneal injection of strictosidinic acid isolated from Psychotria myriantha Mull. Arg. (Rubiaceae). Phytomedicine 2010;17:289–291.
- Soto-Otero R, Méndez-Alvarez E, Hermida-Ameijeiras A, Sánchez-Sellero I, Cruz-Landeira A, Lamas ML. Inhibition of brain monoamine oxidase activity by the generation of hydroxyl radicals: potential implications in relation to oxidative stress. Life Sci 2001;69:879–889.
- Ma J, Yoshimura M, Yamashita E, Nakagawa A, Ito A, Tsukihara T. Structure of rat monoamine oxidase A and its specific recognitions for substrates and inhibitors. J Mol Biol 2004;338:103–114.
- Youdim MB, Edmondson D, Tipton KF. The therapeutic potential of monoamine oxidase inhibitors. Nat Rev Neurosci 2006;7:295–309.
- Youdim MB, Bakhle YS. Monoamine oxidase: isoforms and inhibitors in Parkinson’s disease and depressive illness. Br J Pharmacol 2006;147 Suppl 1:S287–S296.
- Herraiz T. Identification and occurrence of beta-carboline alkaloids in raisins and inhibition of monoamine oxidase (MAO). J Agric Food Chem 2007;55:8534–8540.
- Wang YH, Samoylenko V, Tekwani BL, Khan IA, Miller LS, Chaurasiya ND et al. Composition, standardization and chemical profiling of Banisteriopsis caapi, a plant for the treatment of neurodegenerative disorders relevant to Parkinson’s disease. J Ethnopharmacol 2010;128:662–671.
- Valverde J, Tamayo G, Hesse M. β-Carboline monoterpenoid glucosides from Palicourea adusta. Phytochemistry 1999;52:6.
- Peterson GL. A simplification of the protein assay method of Lowry et al. which is more generally applicable. Anal Biochem 1977;83:346–356.
- van Diermen D, Marston A, Bravo J, Reist M, Carrupt PA, Hostettmann K. Monoamine oxidase inhibition by Rhodiola rosea L. roots. J Ethnopharmacol 2009;122:397–401.
- Copeland R. (2005). Evaluation on enzyme inhibitors in drug discovery: a guide for medicinal chemists and pharmacologists. New York: Wiley, 111–140.
- Djerassi C, Monteiro HJ, Walser A, Durham LJ. Alkaloid studies. LVI. The constitution of vallesiachotamine. J Am Chem Soc 1966;88:1792–1798.
- Taylor C. Psychotria in Lista de Espécies da Flora do Brasil. Jardim Botânico do Rio de Janeiro 2010 [online]. Available at: http://floradobrasil.jbrj.gov.br/2010/FB014153. Accessed on 27 April 2009.
- Waterman PG, Zhong S. Vallesiachotamine and isovallesiachotamine from the seeds of Strychnos tricalysioides. Planta Med 1982;44:696–700.
- van Beek TA, Verpoorte R, Baerheim Svendsen A. Alkaloids from Tabernaemontana psorocarpa. Planta Med 1983;47:83–86.
- Vencato I, Da Silva FM, De Oliveira CMA, Kato L, Tanaka CMA, Da Silva CC, Sabino JR. Vallesiachotamine. Acta Crystallogr E 2006;62:o429–o431.
- Cardoso CL, Siqueira Silva DH, Tomazela DM, Verli H, Young MC, Furlan M et al. Turbinatine, a potential key intermediate in the biosynthesis of corynanthean-type indole alkaloids. J Nat Prod 2003;66:1017–1021.
- Herraiz T, Chaparro C. Analysis of monoamine oxidase enzymatic activity by reversed-phase high performance liquid chromatography and inhibition by beta-carboline alkaloids occurring in foods and plants. J Chromatogr A 2006; 1120:237–243.
- Samoylenko V, Rahman MM, Tekwani BL, Tripathi LM, Wang YH, Khan SI et al. Banisteriopsis caapi, a unique combination of MAO inhibitory and antioxidative constituents for the activities relevant to neurodegenerative disorders and Parkinson’s disease. J Ethnopharmacol 2010;127:357–367.
- Buckholtz NS, Boggan WO. Monoamine oxidase inhibition in brain and liver produced by beta-carbolines: structure-activity relationships and substrate specificity. Biochem Pharmacol 1977;26:1991–1996.
- Dreiseitel A, Korte G, Schreier P, Oehme A, Locher S, Domani M et al. Berry anthocyanins and their aglycons inhibit monoamine oxidases A and B. Pharmacol Res 2009;59:306–311.
- Han XH, Hong SS, Hwang JS, Lee MK, Hwang BY, Ro JS. Monoamine oxidase inhibitory components from Cayratia japonica. Arch Pharm Res 2007;30:13–17.