Abstract
Context: Carbonic anhydrase III (CA III) is a cytosolic enzyme which is known to be highly expressed in the skeletal muscle and has been recently linked to important roles in several physiological processes.
Objective: This review is focused on properties of CA III, including its distribution, function, structure and modulation of enzymatic activity by activators or inhibitors. We also provide some novel data on its expression in murine tissues.
Methods: In this article, the most relevant literature on CA III has been covered. New information on the distribution has been obtained by immunohistochemical staining and western blotting.
Results and conclusion: CA III shows the highest expression in the skeletal muscle and liver. Several other tissues contain lower levels of the enzyme. Activation or inhibition of CA III may offer a novel opportunity to treat some of the diseases linked to the defective expression or function of this enzyme.
Keywords::
Background
Carbonic anhydrases (CAs) are zinc-containing metalloenzymes that are highly expressed in vertebrates including humansCitation1. CAs are encoded by four distinct gene familiesCitation2. The α-CA gene family is dominant in vertebrates, while the other families are mainly present in bacteria, algae and green plants. The CA isozymes differ in their kinetic properties, tissue distributions and subcellular localizationsCitation3. The mammalian α-CA gene family is characterized by 16 different isoforms, 13 of which (CA I, II, III, IV, VA, VB, VI, VII, IX, XII, XIII, XIV, and XV) are enzymatically active, while the other three, the CA-related proteins (CARPs) VIII, X, and XI, lack CA activity2,4,5. CA XV is not expressed in primates, and, therefore, there are only 15 isoforms expressed in humansCitation6. Five of the active CA isozymes are cytosolic (CA I, II, III, VII, and XIII)Citation3,Citation7 and five are membrane-associated enzymes (CA IV, IX, XII, XIV, and XV)Citation8,Citation9. CAs VA and VB are found in mitochondriaCitation10, and CA VI is a secretory protein present in saliva and milkCitation11,Citation12. Most CAs are efficient catalysts for a simple physiological reaction, the hydration of carbon dioxide to bicarbonate and protons: CO2 + H2O ↔ H2CO3 ↔ H+ + HCO3−Citation13,Citation14. The CAs catalyze only the first reaction, while the second occurs instantly. The CAs are produced in different tissues where they participate in numerous biological processes, such as acid-base balance, respiration, carbon dioxide and ion transport, bone resorption, ureagenesis, gluconeogenesis, body fluid generation, and lipogenesisCitation3,Citation7. In addition to these variable physiological processes, the CAs also participate in many pathological processesCitation2. Recently, several studies have identified different CA isozymes in tumors where they contribute to acidification of the extracellular space, which has been linked to the malignant behavior of cancer cells. At least three CA isozymes, CA II, CA IX and CA XII, are overexpressed in various tumors, and are therefore associated with cancer progressionCitation6,Citation15. Many of the CA enzymes are intriguing as drug targets, and some CA inhibitors are already clinically used as therapeutic agents in the prevention and treatment of many diseases including glaucoma and epilepsyCitation16,Citation17. Currently, novel anticancer therapies targeted to CA IX and CA XII are widely investigatedCitation15,Citation18.
In the current review, we explore the data that have emerged on carbonic anhydrase III (CA III). Here, we present the basic structure and putative function of this enzyme. In addition, we explore the activators and inhibitors of CA III. Furthermore, this review contains information of the distribution pattern of CA III in different rodent and human tissues. We also present novel data on the localization of CA III in various mouse tissues. To our knowledge this was the first time when the localization of CA III was thoroughly investigated in mouse tissues by means of immunohistochemistry.
Distribution
The distribution of CA III has been previously studied mainly in human and rat tissues. In rats, CA III is remarkably abundant in the Type I (slow twitch) skeletal muscleCitation19,Citation20, adipocytesCitation21,Citation22 and liver, where it has been localized to the perivenous zoneCitation23,Citation24. Lower concentrations of the enzyme have been detected in the Type II (fast twitch) skeletal muscle, heart, prostate, kidney, brain, and erythrocytesCitation25. CA III has also been located in the epithelium in some regions of the salivary gland ducts, colon, bronchi, and male genital tractCitation26. In humans, CA III has been found abundantly in the skeletal muscleCitation27. It appears that the concentration of CA III in the Type I fibers is higher than in the Type II fibers, a phenomenon also detected in ratsCitation28–30. Trace levels of CA III have also been detected in the human smooth muscle, heart, and lungCitation31.
The concentration of CA III has been found to be higher in the liver of male rats than in female ratsCitation25. Androgen-linked control of CA III expression was investigated by Carter et alCitation24. using a radioimmunosorbent technique. They found that castration of males reduced the CA III concentration to the same level as in females, while testosterone treatment partially restored the enzyme levels. They assumed that CA III belongs to the enzymes of rat liver, which are regulated via the hypothalamo-pituitary-liver axis. Immunohistochemistry also showed that the regulation of CA III is confined to the perivenous hepatocytes. Nevertheless, the marked sex-related difference in CA III distribution, described for rat liver, was not seen in the mouseCitation32.
Our laboratories have recently produced recombinant human CA III protein in Escherichia coliCitation33, and the enzyme was used to raise specific anti-human CA III serum in a rabbit. The novel anti-CA III antibody, which strongly identified a single 30-kDa polypeptide band on the western blot of the purified recombinant human CA III while recognized no other CA isozyme (), was further used to study the distribution pattern of CA III in mouse tissues by western blotting () and immunohistochemistry (). The results obtained in this study are summarized in , which provides a basic overview of CA III distribution in mouse tissues. Our findings indicated the highest expression in the striated muscle and liver. Also, the pancreatic α-cells showed strong immunohistochemical staining, but there was no detectable signal from total pancreas extracts in western blotting. On the basis of western blot results, the stomach, kidney, submandibular gland, heart, cerebellum, and testis expressed CA III. Immunohistochemical staining confirmed that the gastric surface epithelial cells, renal tubules, epithelium of the submandibular salivary ducts, heart, some neuronal axons of the cerebellum, and testicular interstitial cells were indeed positive for CA III immunostaining. Other tissues, including the ileum, colon and lung, also showed weak signals for CA III in the western blot, suggesting that the expression rate is relatively low in those tissues. The results of this study are mainly in agreement with the previously published data from other species. The rat liver, striated muscle21,23,25 and human skeletal muscle20,29–31 are commonly known to contain large amounts of CA III. Previous investigations have provided variable information about the expression of CA III in tissues where the enzyme’s concentration is low and therefore hard to detect. We can assume, though, that the main physiological functions of the enzyme occur in cells where its concentration is high.
Table 1. Distribution of CA III in different mouse tissues.
Figure 1. Western blot of recombinant human CA III identified with the new anti-CA III antibody. The antibody identifies a single 30-kDa polypeptide band. No reactions are seen with the other isozymes.
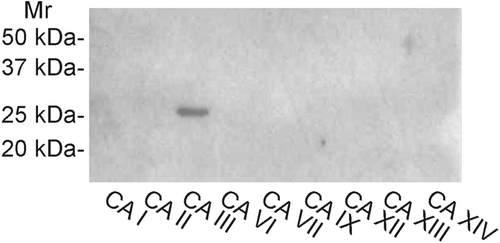
Figure 2. Western blotting of mouse tissues. The strongest 30-kDa polypeptide bands of CA III are detected in the liver, soleus and EDL muscles. Moderate or very faint bands are seen in the stomach, ileum, colon, submandibular gland, kidney, testis, epididymis, uterus, heart, lung, and cerebellum. Female (above) and male (below) mouse tissues were investigated separately.
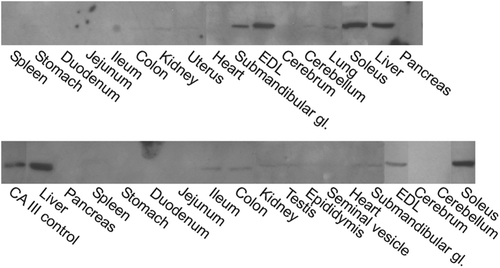
Figure 3. Immunohistochemical staining of CA III in different mouse tissues. The EDL muscle (a) shows occasional strongly stained muscle fibers, while a weaker positive signal is observed in the heart (b). The kidney (c) shows only very faint staining in the renal tubules. In the testis (d), a very weak positive signal is detected in the interstitial cells (arrow). The lung (e) and spleen (f) are completely negative. Bars 50 µm.
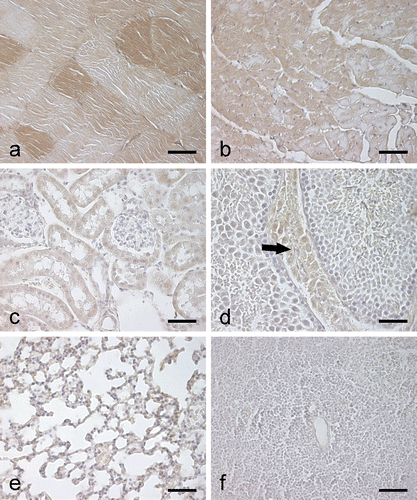
Figure 4. Immunohistochemical staining of CA III in mouse gastrointestinal organs. In the liver (a), the most intense staining is observed in the hepatocytes of perivenular region (cv, central vein). Control staining using pre-immune rabbit serum is negative except for nonspecific nuclear reactions (b). In the submandibular gland (c), the epithelial cells of the salivary duct (arrows) show very weak immunostaining. The stomach also shows a weak positive signal (d), while no staining is detected in the small intestine (e) and colon (f). Bars 50 µm.
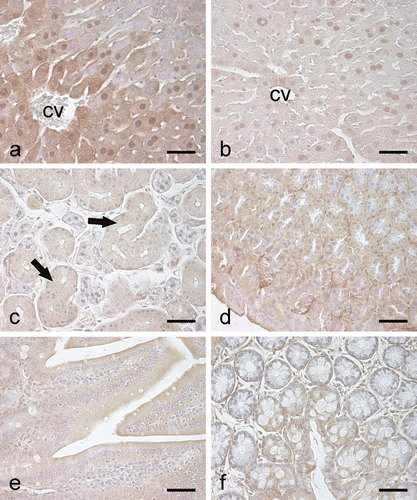
Figure 5. Immunostaining of CA III in the mouse brain. In the cerebrum, the capsula interna (a) shows moderate positive reaction, while the control staining is negative (b). (ci, capsula interna; s, striatum). Some neurons in the hypothalamic region are stained (c) (h, hypothalamus). Control shows no reaction (d). (e) A higher-magnification image in which the CA III-positive staining in the hypothalamic neurons (arrows) is seen more clearly. In the cerebellum (f), some neuronal axons and the Purkinje cells are positively stained. (m, molecular layer; g, granule cell layer; *, neuronal axons). Bars 50 µm.
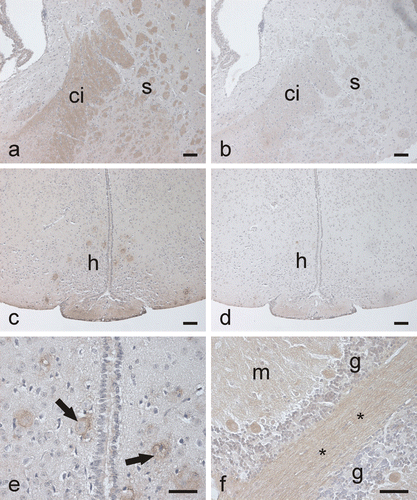
In the present study, we showed that CA III is abundantly expressed in the α-cells of the pancreatic Langerhans islets. This finding raises an interesting question about the function of CA III in the pancreas. Previous studies indicate that CA I is also expressed in the pancreatic α-cells, though there is no information about its functions in this endocrine cell populationCitation34. Therefore, an interesting area for future investigations would be the possible actions of CAs in the synthesis and secretion of glucagon.
In our study, immunohistochemical staining provided evidence that CA III is present in the internal capsule and some neurons of the hypothalamus as well as some neuronal axons and Purkinje cells of the cerebellum. In western blot, a weak signal was also observed for CA III in the cerebellum, while the cerebrum remained negative. The presence of CA IX and XIV in the human and mouse brain has been previously establishedCitation8,Citation14. The membrane-bound CA activity has been suggested to participate in the production of an alkaline shift linked to neuronal signal transductionCitation8. Another cytosolic CA isoform, CA VII, has also been demonstrated to participate in the central nervous system physiologyCitation35. CA VII acts as a key molecule in the generation of high frequency stimulation – induced tonic GABAergic excitation and it may also have a crucial role in shaping integrative functions and long-term plasticity. The results of the present study suggest that CA III might be another isozyme that is expressed in some neurons, even though its expression and enzyme activity levels are low. In earlier studies, it had also been designated that CA III, as well as other CA isoforms, are present in the epithelial cells of rodent and human choroid plexuses, where they participate in the production of the cerebrospinal fluid and regulation of its ionic contentCitation36,Citation37. Yet the presence of CA III in the mouse choroid plexus was not detected in this study.
Many studies have confirmed that the CA III concentrations are remarkably high in both the rodent and human Type I fibers of skeletal muscle. To date, a lot of research has been made to elucidate the value of CA III as a diagnostic marker for rhabdomyolysis and neuromuscular diseasesCitation38. Evidently, serum CA III concentration is elevated in healthy subjects following exercise as well as in patients with neuromuscular diseaseCitation39. The enzyme is released into circulation after muscle injury. It has been suggested that the serum myoglobin/CA III ratio could be a potential, early diagnostic indicator for myocardial infarctionCitation40,Citation41. The ratio tends to rise since CA III is not expressed in the myocardium to the same extent as myoglobin. Vuotikka et al.,Citation42 also demonstrated that measuring the myoglobin/CA III ratio during the first hours after initiation of thrombolytic therapy could be useful in evaluating the success of reperfusion after myocardial infarction.
Structure and function of CA III
CA III is a cytosolic protein with molecular weight of near 30 kDaCitation43. It was first characterized from human skeletal muscle in 1979Citation44. Its overall structure is quite similar to CA II, and there is a 62% identity in the amino acid sequences of these two isozymes in humansCitation43. However, CA III has a CO2 hydration activity of about 0.3% compared to CA IICitation2. The underlying cause for this is the major differences in the active site region of the enzyme: the presence of Lys64 and Phe198 in CA III. CA II contains a His residue in position 64, which is much more effective as a proton shuttle than Lys residueCitation45,Citation46. In order to regenerate the basic form of the enzyme from the catalytically inactive form, a proton transfer reaction takes placeCitation2. This reaction is assisted by the proton shuttles, and it is considered a rate-limiting step in the catalysis of CO2 hydration. Furthermore, position 198 in CA II is occupied by a Leu residue. Leu198 forms part of the hydrophobic pocket of the active site cavity of CA II, which is essential for binding CO2 in the neighborhood of nucleophilic zinc-bound water. Kinetic and structural evidence indicates that the phenyl side chain of Phe198 in human CA III is a steric constriction in the active site and may cause altered interactions at the zinc-bound solvent, resulting in decreased catalytic activity of CA IIICitation47. In previous studies, Phe198 in CA III has been replaced with several other amino acids using site-directed mutagenesis. The results concluded that the replacement of Phe198 by other amino acids markedly increases the CA III activityCitation43. The largest effects were observed with the replacement of Phe to AspCitation48.
In addition to its classical CO2 hydration activity, CA III also shows significant phosphatase activityCitation49. Even though a previous study suggested that the phosphatase activity is related to another protein present in the purified enzyme fractionCitation50, it was recently shown that recombinant CA III exhibits significant phosphatase activity and is more efficient as a phosphatase than CA IICitation51.
Physiological role of CA III has turned out to be complicated, and interestingly, despite many studies the primary function of CA III is yet to be determined. To elucidate its function Kim et al.,Citation52 created a mouse lacking a functional Car3 by gene deletion. The analysis of a knockout mouse strain (Car3−/−) revealed that mice lacking CA III develop normally, are fertile and have a normal life span. The major properties of muscle cells also appeared unchanged, despite minor disturbances which may have occurred. It was hypothesized that CA III is required for an effective response to specific stresses which mice do not encounter in the laboratory facilities or that the other isozymes are sufficient to compensate the lacking CA III. Another recent quantitative study on bioenergetics of the Car3−/− mouse showed that these knockout mice, indeed, had significant defects in muscle functionCitation53. It was concluded that the lack of CA III enzyme impairs mitochondrial ATP synthesis in the gastrocnemius muscle. CA III has also been implicated in fatty acid metabolism. It has been shown that the rat white adipose tissue has CA III concentration as high as 24% of the total cytosolic proteinCitation22,Citation54. Adipocyte CA III expression has been observed to be lower in genetically or experimentally induced obese mice than in corresponding lean controlsCitation22. There has also been evidence that leptin and insulin somehow modulate CA III expression in rat adipose tissue, with leptin decreasing and insulin increasing its expressionCitation54. This suggests that the decrease in CA III expression observed in obesity may be related to hyperleptinemia. It is supposed that CA III provides bicarbonate ion to convert acetyl-CoA into malonyl-CoA, necessary in lipogenesis. Since leptin inhibits fatty acid synthesis, it has been suggested that it might be due to leptin’s effect on CA III expressionCitation54.
According to previous studies CA III plays a role as an oxyradical scavenger of reactive oxygen species and protects cells from oxidative damage in both the liver and skeletal muscleCitation55,Citation56. S-glutathiolation of two cysteine residues, Cys181 and Cys186, occurs rapidly on the surface of the CA III protein in hepatocytes under oxidative stressCitation57. In the absence of glutathione, oxidants such as hydrogen peroxide, peroxyradicals, or hypochlorous acid were shown to produce irreversibly oxidized forms of these cysteine residues, primarily cysteine sulfinic acid or cysteic acidCitation58. When GSH was present, the S-glutathionylation of cysteine residues of CA III occurred without any damage to the protein. Thus, CA III presumably participates in protection of proteins and cellular systems from irreversible oxidative processes.
Recently, CAs have been demonstrated to be involved in tumor progressionCitation15. Acidic pH in extracellular environment of cancer cells contributes to an aggressive phenotype, which facilitates invasion and metastasis of tumor cells into new target organs. The expression of CA III in human hepatocellular carcinoma (HCC) has been studied by Kuo et al.Citation59, who discovered that CA I, CA II and CA III protein expression levels are reduced in human HCC cells compared to the adjoining normal tissues. Dai et al.Citation60, found that the overexpression of CA III results in increased acidity of both extracellular and intracellular environment. They also showed that lowered pH may activate the focal adhesion kinase (FAK) signaling pathway, which in turn plays an important role in cell spreading, migration, and survival. They hypothesized that CA III is re-expressed in later stages of metastatic progression of HCC, and it might have an important influence in the development of metastasis in liver cancer.
CA III has been linked among other CAs, such as CA II, to autoimmune diseases. A recent study showed the presence of specific autoantibodies directed to CA III in the sera of rheumatoid arthritis (RA) patientsCitation61. It was also discovered that CA III was overexpressed in the synovial membrane of RA patients but it was not observed in non-RA patients. The results led to the conclusion that CA III expression might result from a physiological response aimed at protecting cells from oxidative damage, and anti-CA III antibodies reduce the protective effect of CA III. However, further investigations are required to confirm the role of CA III in the pathophysiology of RA. Du et al.Citation62, have also shown that the amount of CA III is diminished in the skeletal muscle of myasthenia gravis patients. Thus, CA III insufficiency or antibody response against CA III enzyme may play a role in the pathogenesis of certain autoimmune diseases.
Activation and inhibition of CA III
The activation of CA III has been investigated only in few studies, and in fact, the first activation study of human CA III was published in 2008 by Supuran’s groupCitation63 who investigated the activation of human CA III and CA IV with a series of natural and non-natural amino acids and aromatic/heterocyclic amines. The same group had earlier reported the activation profiles of other mammalian catalytically active isoformsCitation64–70. The results concluded that the activation profile of human CA III was distinct from all other CA isoforms investigated so far. Only one amino acid was a good activator of human CA III while all the other effective derivatives were heterocyclic amines. Human CA III was effectively activated by D-His, serotonin, pyridyl-alkylamines, and aminoethyl-piperazine and morpholine, with their activation constants varying between 91 nM and 1.12 µM. Notably, while the human CA III was very significantly activated by D-His, the enantiomeric form l-His was a much weaker activator. Similar effect was also observed with the other compounds and their enantiomers, also among the other CA isoforms. X-ray crystal data of the complexes of human CA I and II with l- and d-His has shown that both the stereoisomerism of the amino acid activator and the CA isoform define the binding site and orientation. Therefore, precise steric and electric properties are needed in the molecules of CA activators, in order to assure the formation of enzyme-activator complex. It was also observed that small modifications in the scaffold of some activators, for example adding a substituent in the phenyl moiety of l-Phe such as the phenolic moieties of l-DOPA lead to important changes in the CA activator properties. It is estimated that CA activators do not influence the binding of CO2 to the active site, but they interfere in the transfer of protons from the active site to the environment. This is described as the rate-limiting step of the catalysis. It is supposed that the different activation profile of CA III isoform by amino acids and amines may be due to the different amino acid residues present in the active site. Those include Lys residue in position 64 and Leu residue in position 198, as discussed earlier in this articleCitation63.
There are two well-known classes of CA inhibitors: inorganic anions and sulfonamides/sulfamatesCitation2. The inhibitors of the sulfonamide type, which constitute the principal type of classical CA inhibitors, bind to the catalytically critical Zn(II) ion of the enzyme active site. The organic part of the inhibitor also participates in several hydrogen bonds with the hydrophilic and hydrophobic residues of the enzyme active site. For anionic inhibitors such as thiocyanate, nitrite, bisulfate etc. the interactions between inhibitor and enzyme are much simpler. CA III has been reported to be quite insensitive to sulfonamide inhibitors, which do not have enough space to bind in the vicinity of the Zn(II) ion, due to the presence of the bulky residue, Phe198Citation71. Only the very small sulfonamide, trifluoromethanesulfonamide, acts as a potent CA III inhibitor, with an inhibition constant of 0.9 µMCitation2. Clinically used compounds such as acetazolamide, methazolamide, topiramate, zonisamide and celecoxib are less effective CA III inhibitorsCitation72. CA III possesses a completely different inhibition profile with anions such as stannate, selenate, tellurate, persulfate, selenocyanate and trithiocarbonate when compared to the CA isoforms I, II, VII and XIIICitation73. These inorganic anions are somewhat less investigated. Trithiocarbonate inhibited human CA III efficiently, with an inhibition constant of 9.9 µM. Other investigated anionic inhibitors inhibit CA III with inhibition constants in the millimolar range. In another inhibition study with more common anions it was observed that fluoride, nitrate, nitrite, phenylboronic acid, and phenylarsonic acid were weak CA III inhibitors, whereas carbonate, cyanide, thiocyanate, azide and hydrogen sulfide were significantly more effectiveCitation74. It is important to understand the physiological functions of mammalian CA isozymes as well as the catalytic and inhibition mechanisms of these enzymes. This has resulted in the design of potent inhibitors, some of which possess important clinical applicationsCitation75. Some inhibitors of CA isozymes are already widely used as antiglaucoma drugs, diuretics, antiepileptics, in the management of gastric and duodenal ulcers or osteoporosis among othersCitation2. Today, extensive research is devoted in the drug design of anticancer agents and antiobesity drugs and CAs are clearly considered potential targets for some of these drugsCitation6,Citation76.
Conclusions
CA III has long been a neglected isozyme among the alpha-CA enzyme family because of its low catalytic activity. Recent studies have indicated, however, that CA III is involved in important physiological processes or phenomena, such as oxidative stress response, obesity, mitochondrial ATP synthesis, autoimmunity, and carcinogenesis. Therefore, activation or inhibition of this enzyme by various compounds may offer a novel opportunity to treat some of the diseases linked to the defective expression or function of CA III.
Declaration of interest
This study was funded by Academy of Finland, Sigrid Juselius Foundation, and Competitive Research Funding of the Tampere University Hospital (9M075, 9N054). The authors thank Dr. Merja Bläuer for providing the glucagon antibody. The authors report no declarations of interest.
References
- Supuran CT. Carbonic anhydrases: novel therapeutic applications for inhibitors and activators. Nat Rev Drug Discov 2008;7:168–181.
- Supuran CT, Scozzafava A. Carbonic anhydrases as targets for medicinal chemistry. Bioorg Med Chem 2007;15:4336–4350.
- Lehtonen J, Shen B, Vihinen M, Casini A, Scozzafava A, Supuran CT et al. Characterization of CA XIII, a novel member of the carbonic anhydrase isozyme family. J Biol Chem 2004;279:2719–2727.
- Aspatwar A, Tolvanen ME, Parkkila S. Phylogeny and expression of carbonic anhydrase-related proteins. BMC Mol Biol 2010;11:25.
- Taniuchi K, Nishimori I, Takeuchi T, Ohtsuki Y, Onishi S. cDNA cloning and developmental expression of murine carbonic anhydrase-related proteins VIII, X, and XI. Brain Res Mol Brain Res 2002;109:207–215.
- Neri D, Supuran CT. Interfering with pH regulation in tumours as a therapeutic strategy. Nat Rev Drug Discov 2011;10:767–777.
- Sly WS, Hu PY. Human carbonic anhydrases and carbonic anhydrase deficiencies. Annu Rev Biochem 1995;64:375–401.
- Parkkila S, Parkkila AK, Rajaniemi H, Shah GN, Grubb JH, Waheed A et al. Expression of membrane-associated carbonic anhydrase XIV on neurons and axons in mouse and human brain. Proc Natl Acad Sci USA 2001;98:1918–1923.
- Pastoreková S, Parkkila S, Parkkila AK, Opavský R, Zelník V, Saarnio J et al. Carbonic anhydrase IX, MN/CA IX: analysis of stomach complementary DNA sequence and expression in human and rat alimentary tracts. Gastroenterology 1997;112:398–408.
- Fujikawa-Adachi K, Nishimori I, Taguchi T, Onishi S. Human mitochondrial carbonic anhydrase VB. cDNA cloning, mRNA expression, subcellular localization, and mapping to chromosome x. J Biol Chem 1999;274:21228–21233.
- Karhumaa P, Leinonen J, Parkkila S, Kaunisto K, Tapanainen J, Rajaniemi H. The identification of secreted carbonic anhydrase VI as a constitutive glycoprotein of human and rat milk. Proc Natl Acad Sci USA 2001;98:11604–11608.
- Kivelä J, Parkkila S, Parkkila AK, Rajaniemi H. A low concentration of carbonic anhydrase isoenzyme VI in whole saliva is associated with caries prevalence. Caries Res 1999;33:178–184.
- Breton S. The cellular physiology of carbonic anhydrases. JOP 2001;2:159–164.
- Hilvo M, Rafajová M, Pastoreková S, Pastorek J, Parkkila S. Expression of carbonic anhydrase IX in mouse tissues. J Histochem Cytochem 2004;52:1313–1322.
- Parkkila S. Significance of pH regulation and carbonic anhydrases in tumour progression and implications for diagnostic and therapeutic approaches. BJU Int 2008;101 Suppl 4:16–21.
- Mincione F, Scozzafava A, Supuran CT. The development of topically acting carbonic anhydrase inhibitors as antiglaucoma agents. Curr Pharm Des 2008;14:649–654.
- Thiry A, Dogné JM, Supuran CT, Masereel B. Carbonic anhydrase inhibitors as anticonvulsant agents. Curr Top Med Chem 2007;7:855–864.
- Battke C, Kremmer E, Mysliwietz J, Gondi G, Dumitru C, Brandau S et al. Generation and characterization of the first inhibitory antibody targeting tumour-associated carbonic anhydrase XII. Cancer Immunol Immunother 2011;60:649–658.
- Jeffery S, Carter ND, Smith A. Immunocytochemical localization of carbonic anhydrase isozymes I, II, and III in rat skeletal muscle. J Histochem Cytochem 1986;34:513–516.
- Wade R, Gunning P, Eddy R, Shows T, Kedes L. Nucleotide sequence, tissue-specific expression, and chromosome location of human carbonic anhydrase III: the human CAIII gene is located on the same chromosome as the closely linked CAI and CAII genes. Proc Natl Acad Sci USA 1986;83:9571–9575.
- Spicer SS, Ge ZH, Tashian RE, Hazen-Martin DJ, Schulte BA. Comparative distribution of carbonic anhydrase isozymes III and II in rodent tissues. Am J Anat 1990;187:55–64.
- Stanton LW, Ponte PA, Coleman RT, Snyder MA. Expression of CA III in rodent models of obesity. Mol Endocrinol 1991;5:860–866.
- Kelly CD, Carter ND, de Boer P, Jeffery S, Moorman AF, Smith A. Detection of CAIII mRNA in rat skeletal muscle and liver by in situ hybridization. J Histochem Cytochem 1991;39:1243–1247.
- Carter N, Lönnerholm G, Meyerson B, Wistrand P. Androgen-linked control of carbonic anhydrase III expression occurs in rat perivenous hepatocytes; an immunocytochemical study. Ups J Med Sci 2001;106:67–76.
- Shiels A, Jeffery S, Wilson C, Carter N. Radioimmunoassay of carbonic anhydrase III in rat tissues. Biochem J 1984;218:281–284.
- Spicer SS, Ge ZH, Tashian RE, Hazen-Martin DJ, Schulte BA. Comparative distribution of carbonic anhydrase isozymes III and II in rodent tissues. Am J Anat 1990 187(1):55–64.
- Kato K, Mokuno K. Distribution of immunoreactive carbonic anhydrase III in various human tissues determined by a sensitive enzyme immunoassay method. Clin Chim Acta 1984;141:169–177.
- Shima K. Human muscle carbonic anhydrase III (CA-III). Purification, immunohistochemical localization in the human skeletal muscle and its clinical application to the neuromuscular disease. Hokkaido Igaku Zasshi 1984;59:98–116.
- Väänänen HK, Paloniemi M, Vuori J. Purification and localization of human carbonic anhydrase. III. Typing of skeletal muscle fibers in paraffin embedded sections. Histochemistry 1985;83:231–235.
- Zheng A, Rahkila P, Vuori J, Rasi S, Takala T, Väänänen HK. Quantification of carbonic anhydrase III and myoglobin in different fiber types of human psoas muscle. Histochemistry 1992;97:77–81.
- Jeffery S, Edwards Y, Carter N. Distribution of CAIII in fetal and adult human tissue. Biochem Genet 1980;18:843–849.
- Tweedie S, Edwards Y. Mouse carbonic anhydrase III: nucleotide sequence and expression studies. Biochem Genet 1989;27:17–30.
- Bootorabi F, Jänis J, Hytönen VP, Valjakka J, Kuuslahti M, Vullo D et al. Acetaldehyde-derived modifications on cytosolic human carbonic anhydrases. J Enzyme Inhib Med Chem 2011;26:862–870.
- Parkkila S, Parkkila AK, Juvonen T, Lehto VP, Rajaniemi H. Immunohistochemical demonstration of the carbonic anhydrase isoenzymes I and II in pancreatic tumours. Histochem J 1995;27:133–138.
- Ruusuvuori E, Li H, Huttu K, Palva JM, Smirnov S, Rivera C et al. Carbonic anhydrase isoform VII acts as a molecular switch in the development of synchronous gamma-frequency firing of hippocampal CA1 pyramidal cells. J Neurosci 2004;24:2699–2707.
- Nógrádi A, Kelly C, Carter ND. Localization of acetazolamide-resistant carbonic anhydrase III in human and rat choroid plexus by immunocytochemistry and in situ hybridisation. Neurosci Lett 1993;151:162–165.
- Halmi P, Parkkila S, Honkaniemi J. Expression of carbonic anhydrases II, IV, VII, VIII and XII in rat brain after kainic acid induced status epilepticus. Neurochem Int 2006;48:24–30.
- Syrjälä H, Vuori J, Huttunen K, Väänänen HK. Carbonic anhydrase III as a serum marker for diagnosis of rhabdomyolysis. Clin Chem 1990;36:696.
- Väänänen HK, Takala TE, Tolonen U, Vuori J, Myllylä VV. Muscle-specific carbonic anhydrase III is a more sensitive marker of muscle damage than creatine kinase in neuromuscular disorders. Arch Neurol 1988;45:1254–1256.
- Väänänen HK, Syrjälä H, Rahkila P, Vuori J, Melamies LM, Myllylä V et al. Serum carbonic anhydrase III and myoglobin concentrations in acute myocardial infarction. Clin Chem 1990;36:635–638.
- Beuerle JR, Azzazy HM, Styba G, Duh SH, Christenson RH. Characteristics of myoglobin, carbonic anhydrase III and the myoglobin/carbonic anhydrase III ratio in trauma, exercise, and myocardial infarction patients. Clin Chim Acta 2000;294:115–128.
- Vuotikka P, Uusimaa P, Niemelä M, Väänänen K, Vuori J, Peuhkurinen K. Serum myoglobin/carbonic anhydrase III ratio as a marker of reperfusion after myocardial infarction. Int J Cardiol 2003;91:137–144.
- Tu C, Chen X, Ren X, LoGrasso PV, Jewell DA, Laipis PJ et al. Interactions of active-site residues and catalytic activity of human carbonic anhydrase III. J Biol Chem 1994;269:23002–23006.
- Carter N, Jeffery S, Shiels A, Edwards Y, Tipler T, Hopkinson DA. Characterization of human carbonic anhydrase III from skeletal muscle. Biochem Genet 1979;17:837–854.
- An H, Tu C, Ren K, Laipis PJ, Silverman DN. Proton transfer within the active-site cavity of carbonic anhydrase III. Biochim Biophys Acta 2002;1599:21–27.
- Elder I, Fisher Z, Laipis PJ, Tu C, McKenna R, Silverman DN. Structural and kinetic analysis of proton shuttle residues in the active site of human carbonic anhydrase III. Proteins 2007;68:337–343.
- Duda DM, Tu C, Fisher SZ, An H, Yoshioka C, Govindasamy L et al. Human carbonic anhydrase III: structural and kinetic study of catalysis and proton transfer. Biochemistry 2005;44:10046–10053.
- LoGrasso PV, Tu C, Chen X, Taoka S, Laipis PJ, Silverman DN. Influence of amino acid replacement at position 198 on catalytic properties of zinc-bound water in human carbonic anhydrase III. Biochemistry 1993;32:5786–5791.
- Koester MK, Pullan LM, Noltmann EA. The p-nitrophenyl phosphatase activity of muscle carbonic anhydrase. Arch Biochem Biophys 1981;211:632–642.
- Kim G, Selengut J, Levine RL. Carbonic anhydrase III: the phosphatase activity is extrinsic. Arch Biochem Biophys 2000;377:334–340.
- Truppo E, Supuran CT, Sandomenico A, Vullo D, Innocenti A, Di Fiore A et al. Carbonic anhydrase VII is S-glutathionylated without loss of catalytic activity and affinity for sulfonamide inhibitors. Bioorg Med Chem Lett 2012;22:1560–1564.
- Kim G, Lee TH, Wetzel P, Geers C, Robinson MA, Myers TG et al. Carbonic anhydrase III is not required in the mouse for normal growth, development, and life span. Mol Cell Biol 2004;24:9942–9947.
- Liu M, Walter GA, Pathare NC, Forster RE, Vandenborne K. A quantitative study of bioenergetics in skeletal muscle lacking carbonic anhydrase III using 31P magnetic resonance spectroscopy. Proc Natl Acad Sci USA 2007;104:371–376.
- Alver A, Uçar F, Keha EE, Kalay E, Ovali E. Effects of leptin and insulin on CA III expression in rat adipose tissue. J Enzyme Inhib Med Chem 2004;19:279–281.
- Räisänen SR, Lehenkari P, Tasanen M, Rahkila P, Härkönen PL, Väänänen HK. Carbonic anhydrase III protects cells from hydrogen peroxide-induced apoptosis. FASEB J 1999;13:513–522.
- Zimmerman UJ, Wang P, Zhang X, Bogdanovich S, Forster R. Anti-oxidative response of carbonic anhydrase III in skeletal muscle. IUBMB Life 2004;56:343–347.
- Mallis RJ, Poland BW, Chatterjee TK, Fisher RA, Darmawan S, Honzatko RB et al. Crystal structure of S-glutathiolated carbonic anhydrase III. FEBS Lett 2000;482:237–241.
- Mallis RJ, Hamann MJ, Zhao W, Zhang T, Hendrich S, Thomas JA. Irreversible thiol oxidation in carbonic anhydrase III: protection by S-glutathiolation and detection in aging rats. Biol Chem 2002;383:649–662.
- Kuo WH, Chiang WL, Yang SF, Yeh KT, Yeh CM, Hsieh YS et al. The differential expression of cytosolic carbonic anhydrase in human hepatocellular carcinoma. Life Sci 2003;73:2211–2223.
- Dai HY, Hong CC, Liang SC, Yan MD, Lai GM, Cheng AL et al. Carbonic anhydrase III promotes transformation and invasion capability in hepatoma cells through FAK signaling pathway. Mol Carcinog 2008;47:956–963.
- Robert-Pachot M, Desbos A, Moreira A, Becchi M, Tebib J, Bonnin M et al. Carbonic anhydrase III: a new target for autoantibodies in autoimmune diseases. Autoimmunity 2007;40:380–389.
- Du AL, Ren HM, Lu CZ, Tu JL, Xu CF, Sun YA. Carbonic anhydrase III is insufficient in muscles of myasthenia gravis patients. Autoimmunity 2009;42:209–215.
- Vullo D, Nishimori I, Scozzafava A, Supuran CT. Carbonic anhydrase activators: Activation of the human cytosolic isozyme III and membrane-associated isoform IV with amino acids and amines. Bioorg Med Chem Lett 2008;18:4303–4307.
- Temperini C, Scozzafava A, Vullo D, Supuran CT. Carbonic anhydrase activators. Activation of isozymes I, II, IV, VA, VII, and XIV with l- and d-histidine and crystallographic analysis of their adducts with isoform II: engineering proton-transfer processes within the active site of an enzyme. Chemistry 2006;12:7057–7066.
- Vullo D, Innocenti A, Nishimori I, Scozzafava A, Kaila K, Supuran CT. Carbonic anhydrase activators: activation of the human isoforms VII (cytosolic) and XIV (transmembrane) with amino acids and amines. Bioorg Med Chem Lett 2007;17:4107–4112.
- Vullo D, Nishimori I, Innocenti A, Scozzafava A, Supuran CT. Carbonic anhydrase activators: an activation study of the human mitochondrial isoforms VA and VB with amino acids and amines. Bioorg Med Chem Lett 2007;17:1336–1340.
- Nishimori I, Onishi S, Vullo D, Innocenti A, Scozzafava A, Supuran CT. Carbonic anhydrase activators: the first activation study of the human secretory isoform VI with amino acids and amines. Bioorg Med Chem 2007;15:5351–5357.
- Pastorekova S, Vullo D, Nishimori I, Scozzafava A, Pastorek J, Supuran CT. Carbonic anhydrase activators: activation of the human tumor-associated isozymes IX and XII with amino acids and amines. Bioorg Med Chem 2008;16:3530–3536.
- Temperini C, Innocenti A, Scozzafava A, Mastrolorenzo A, Supuran CT. Carbonic anhydrase activators: L-Adrenaline plugs the active site entrance of isozyme II, activating better isoforms I, IV, VA, VII, and XIV. Bioorg Med Chem Lett 2007;17:628–635.
- Temperini C, Scozzafava A, Puccetti L, Supuran CT. Carbonic anhydrase activators: X-ray crystal structure of the adduct of human isozyme II with L-histidine as a platform for the design of stronger activators. Bioorg Med Chem Lett 2005;15:5136–5141.
- Biswas S, Aggarwal M, Güzel Ö, Scozzafava A, McKenna R, Supuran CT. Conformational variability of different sulfonamide inhibitors with thienyl-acetamido moieties attributes to differential binding in the active site of cytosolic human carbonic anhydrase isoforms. Bioorg Med Chem 2011;19:3732–3738.
- Nishimori I, Minakuchi T, Onishi S, Vullo D, Cecchi A, Scozzafava A et al. Carbonic anhydrase inhibitors: cloning, characterization, and inhibition studies of the cytosolic isozyme III with sulfonamides. Bioorg Med Chem 2007;15:7229–7236.
- Innocenti A, Scozzafava A, Supuran CT. Carbonic anhydrase inhibitors. Inhibition of cytosolic isoforms I, II, III, VII and XIII with less investigated inorganic anions. Bioorg Med Chem Lett 2009;19:1855–1857.
- Nishimori I, Minakuchi T, Onishi S, Vullo D, Cecchi A, Scozzafava A et al. Carbonic anhydrase inhibitors. Cloning, characterization and inhibition studies of the cytosolic isozyme III with anions. J Enzyme Inhib Med Chem 2009;24:70–76.
- Supuran CT, Vullo D, Manole G, Casini A, Scozzafava A. Designing of novel carbonic anhydrase inhibitors and activators. Curr Med Chem Cardiovasc Hematol Agents 2004;2:49–68.
- Supuran CT. Carbonic anhydrase inhibitors as emerging drugs for the treatment of obesity. Expert Opin Emerg Drugs 2012;17:11–15.