Abstract
Some novel derivatives of Bis-chalcone were synthesized and characterized by their physical and spectral data. All the synthesized compounds were subsequently screened for in vitro globin hydrolysis, β-hematin formation, and murine Plasmodium berghei, using chloroquine as the reference drug. Most of the synthesized compounds exhibited mild to moderate susceptibilities toward the parasite in comparison with the standard. The most active antimalarial compound was 1,1-Bis-[(3′,4′-N-(urenylphenyl)-3-(3″,4″,5″-trimethoxyphenyl)]-2-propen-1-one 5, with a percentage of inhibition of heme polymerization of 87.05 ± 0.77, and this compound increased the survival time after infection, reduce the parasitemia and delay the progression of malaria.
Introduction
Infectious diseases are influencing the world with their morbidity and mortality. Malaria is the most infectious parasitic disease in the world caused by Plasmodium falciparum, which has become the most virulent among the four species infecting humans and is responsible for most of mortalityCitation1–3. In 2008, among 3.3 billion people at risk, there were 243 million malaria cases, causing an estimated 863,000 deaths, mostly of children below 5 yearsCitation4,Citation5. The current chemotherapy is based on age old drugs such as chloroquine, pyrimethamine-sulfadoxine for malariaCitation1–3. The efficacy of these drugs has been deteriorated by the emerging resistant strainsCitation6.
The development of new drugs will be greatly facilitated by a complete understanding of the molecular mechanism underlying previously successful antimalarial drugsCitation7. For structurally simple group of compounds which act on more than one target site are liable to be more active. The chalcones have displayed an impressive array of pharmacological activities such as antiprotozoaCitation8–10, anti-inflammatoryCitation11,Citation12, antituberculosis agentsCitation13, immunomodulatoryCitation14, and anticancerCitation15.
As our research is devoted to the synthesis of diverse structures as anti-infective agents, we identified the chalcone as good anti-infectious agentsCitation16–18. Keeping this in mind, we designed and synthesized new prototypes by combining both urenyl Bis-acetophenone and substituted benzaldehyde to yield the corresponding Bis-chalcones () and highlighted their in vitro and in vivo antimalarial activities.
Materials and methods
Melting points were determined in a Thomas micro hot stage apparatus and are uncorrected. Infrared spectra were determined as KBr pellets on a Shimadzu model 470 spectrophotometer and are expressed in cm−1. 1H NMR and 13C NMR spectra were recorded on a JEOL GSX (270 MHz) spectrometer; chemical shifts are expressed in δ (ppm) relative to tetramethylsilane. Mass spectral data were obtained with a Varian CP3800 model coupled with Saturn 2000/Gas Chromatograph ionization energy 70 eV, using chemical ionization mass spectrometry (CIMS). Elemental analyses were performed by Atlantic Microlab; Norcross, GA, USA, results were within ± 0.4% of predicted values for all compounds. All solvents were dried and distilled under nitrogen atmosphere. Analytical TLCs were run on commercially precoated plates (Merck, silica gel 60F254) and visualized under UV lamps (254 nm). The progress of the reactions was monitored by TLC with ethyl acetate: hexane (1:1 v/v) as eluent.
Synthesis of 3, 4-N-urenil Bis-acetophenone (3)
A mixture of the corresponding p-aminoacetophenone (1 mmol) and recently distilled m-acethylphenylisocyanates(1 mmol) in dry acetone (5 mL) was stirred under nitrogen atmosphere at room temperature for 3–7 h, according to the previously described methodology with some modificationsCitation19. The resulting precipitate was washed with cold water and then filtered off; the crude product was recrystallized in methanol-water.
Yield 78–90%, mp. 245–247°C. 1H NMR: δH 2.51 (s, 3H, CH3), 2.57 (s, 3H, CH3), 7.46 (d, 1H, H4a, J = 7.47 Hz), 7.61 (d, 2H, H3–5, J = 7.92 Hz), 7.68 (d, 1H, H6a, J = 7.91 Hz), 7.91 (d, 2H, H2–6, J = 8.41 Hz), 9.04 (s, 1H, NH), 9.15 (s, 1H, NH); 13C NMR: δ13C 26.18 (CH3), 26.38 (CH3), 116.88 (C6′), 118.15 (C3–5), 118.36 (C2′), 121.98 (C4′), 129.11 (C5′), 129.68 (C1), 130.12 (C2–6), 132.57 (C1′), 138.77 (C3′), 140.98 (C4), 152.75 [CO (NH)2], 196.78 (CO), 197.15 (CO).
General procedure for the synthesis of urenyl Bis-chalcone (4–17)
A mixture of 3, 4-N-urenyl-Bis-acetophenone (3) (1 mmol), the appropriate substituted benzaldehyde (2 mmol), and sodium hydroxide (2.5 mmol) in dry methanol was usedCitation20. The reaction mixture was stirred at room temperature for 3–7 h, and the resulting precipitates were filtered and recrystallized from methanol-water.
1, 1,-Bis-[(3′, 4′-N-) urenylphenyl)-3(2″,4″-dimethoxyphenyl)]-2-propen-1-one (4)
Yield 17%, mp. 185–186°C 1H NMR: δH 3.81 (s, 6H, OCH3), 3.85 (s, 6H, OCH3), 7.14 (d, 2H, H5, J = 8.15 Hz), 7.47 (d, 2H, Hα, J = 15.85 Hz), 7.61 (d, 2H, H3′,5′, J = 8.66 Hz), 7.63 (d, 1H, H6′a, J = 8.68 Hz), 7.81 (d, 2H, Hβ, J = 15.84 Hz), 7.85 (d, 2H, H2′,6′, J =8.42 Hz), 8.07 (s, 1H, H2′a).; 13C NMR: δ13C 55.33 (OCH3), 55.63 (OCH3), 97.43 (C3″), 105.75 (C5″), 116.99 (C1″), 120.04 (C3′–5′), 120.55 (Cα), 121.22 (C2′a), 123.97 (C6′a), 125.28 (C4′a), 130.14 (C5′a), 130.34 (C6″), 131.95 (C2′–6′), 132.15 (C1′), 138.02 (C1′a), 138.98 (Cβ), 141.02 (C3′a), 145.12 (C4′), 152.77 (CO(NH)2), 159.23 (C4″), 163.03 (C2″), 188.46 (CO), 190.01 (CO); CIMS (m/z) data 593 [M+H]; Anal. Calculated: C, 70.93%; H, 5.44%; N, 4.73%; O, 18.90%; Found: C, 70.14%; H, 5.82%; N, 4.88%; O, 19.05%.
1, 1-Bis-[(3′,4′-N-(urenylphenyl)-3-(3″,4″,5″-trimethoxyphenyl)]-2-propen-1-one (5)
Yield 96%, mp. 145–146°C 1H NMR: δH 3.72 (s, 6H, OCH3), 3.87 (s, 12H, OCH3) 7.23 (d, 2H, H2′,6′, J = 9.60 Hz) 7.67 (d, 2H, Hα, J = 15.34 Hz), 7.70 (d, 2H, H3′,5′, J = 7.18 Hz), 7.90 (d, 2H, Hβ, J = 15.58 Hz), 8.17 (s, 1H, H2′a), 9.68 (bs, 2H, NH); 13C NMR: δ13C 59.93 (OCH3), 60.17 (OCH3), 108.75 (C2″–6″), 119.98 (C3′,5′), 120.55 (C2′a), 123.22 (Cα), 124.17 (C6′a), 125.28 (C4′a), 130.14 (C5′a), 131.34 (C2′–6′), 131.65 (C1″), 132.15 (C1′), 139.02 (C1′a), 141.02 (C3′a), 144.42 (Cβ), 145.12 (C4′), 149.95 (C4″), 152.77 (CO(NH)2), 153.02 (C3′–5′), 188.53 (CO), 190.01 (CO); Anal. Calculated: C, 68.09%; H, 5.56%; N, 4.29%; O, 22.06%; Found: C, 67.99%; H, 5.32%; N, 4.39%; O, 21.98%.
1,1-Bis-[(3′,4′-N-(urenylphenyl)-3-(2″,5″-dimethoxyphenyl)]-2-propen-1-one (6)
Yield 43%, mp. 164–165°C. 1H NMR data: δH 3.90 (s, 6H, OCH3), 3.92 (s, 6H, OCH3), 7.47 (d, 2H, Hα, J = 15.82 Hz), 7.69 (d, 4H, H3′,5′, J = 8.81 Hz), 7.86 (d, 2H, H2′,6′, J = 8.10 Hz), 7.91 (d, 2H, Hβ, J = 15.88 Hz), 7.69 (d, 1H, H6′a, J =8.91 Hz), 8.02 (s, 1H, H2′a), 10.06 (s, 1H, NH), 10.17 (s, 1H, NH);13C NMR: δ13C 55.93 (OCH3), 56.97 (OCH3), 112.43 (C3″), 113.75 (C6″), 116.67 (C4″), 119.99 (C3′–5′), 120.04 (C2′a), 123.65 (Cα), 124.07 (C6′a), 124.88 (C1″), 125.28 (C4′a), 130.04 (C5′a), 131.15 (C2′–6′), 132.07 (C1′), 138.02 (C1′a), 139.11 (Cβ), 140.82 (C3′a), 144.27 (C4′), 150.65 (C5″), 152.73 (CO(NH)2), 153.23 (C2″), 188.86 (CO), 190.01 (CO); CIMS (m/z) data 593 [M+H]; Anal. Calculated:C, 70.93%; H, 5.44%; N, 4.73%; O, 18.90%; Found: C, 71.02%; H, 5.29%; N, 4.55%; O, 19.01%.
1,1-Bis-[(3′,4′-N-(urenylphenyl)-3-(3″,4″-dichlorophenyl)]-2-propen-1-one (7)
Yield 80%, mp. 182–185°C. 1H NMR: δH 7.60 (d, 2H, Hα, J = 15.36 Hz), 7.71 (d, 2H, H3′,5′, J = 7.10 Hz), 7.88 (d, 1H, H6′a, J = 8.66 Hz), 7.85 (d, 2H, H2′,6′, J = 8.62 Hz), 7.96 (d, 2H, Hβ, J = 15.82 Hz), 8.09 (s, 1H, H2′a); 13C NMR: δ13C 119.17 (C3′–5′), 120.74 (C2′a), 123.29 (Cα), 123.99 (C6′a), 125.28 (C4′a), 127,53 (C6″), 128.04 (C2″), 129.88 (C5′a), 130.44 (C5″), 131.13 (C2′–6′), 132.02 (C4″), 132.43 (C1′), 132.86 (C3″), 135.23 (C1″), 140.04 (C1′a), 140.94 (C3′a), 142.74 (Cβ), 144.72 (C4′), 152.77 (CO(NH)2), 188.96 (CO), 190.01 (CO); CIMS (m/z) data 611 [M+H]; Anal. Calculated:C, 61.01%; H, 3.30%; Cl, 23.24%; N, 4.59%; O, 7.86%; Found: C, 60.97%; H, 3.24%; Cl, 23.56%; N, 4.55%; O, 7.79%.
1,1-Bis-[(3′,4′-N-(urenylphenyl)-3-(3″,4″-dimethoxyphenyl)]-2-propen-1-one (8)
Yield 52%, mp. 225–227°C. 1H NMR: δH 3.81 (s, 6H, OCH3), 3.86 (s, 6H, OCH3), 7.49 (d, 2H, Hα, J = 15.41 Hz), 7.68 (d, 1H, H6′a, J = 8.15 Hz), 7.71(d, 2H, H3′,5′, J = 7.63 Hz), 7.78 (d, 2H, Hβ, J = 15.86 Hz), 7.85 (d, 2H, H2′,6′, J = 8.13 Hz), 8.14 (s, 1H, H2′a), 9.84 (bs, 2H, NH); 13C NMR: δ13C 56.33 (OCH3), 113.95 (C2″), 114.75 (C5″), 119.84 (C3′–5′), 120.98 (C2′a), 121.34 (C6″), 123.55 (Cα), 124.07 (C6′a), 125.28 (C4′a), 128.49 (C1″), 130.14 (C5′a), 131.75 (C2′–6′), 132.15 (C1′), 139.32 (C1′a), 140.88 (C3′a), 144.52 (C4′), 145.18 (Cβ), 149.43 (C3″), 152.23 (C4″), 152.77 (CO(NH)2), 188.66 (CO), 190.01 (CO); CIMS (m/z) data 593 [M+H]; Anal. Calculated: C, 70.93%; H, 5.44%; N, 4.73%; O, 18.90%; Found: C, 71.02%; H, 5.42%; N, 4.56%; O, 18.88%.
1,1-Bis-[(3′,4′-N-(urenylphenyl)-3-(2″,4″,6″-trimethoxyphenyl)]-2-propen-1-one (9)
Yield 49%, mp. 198–200°C. 1H NMR: δH 3.81 (s, 6H, OCH3), 3.86 (s, 12H, OCH3) 7.49 (d, 2H, Hα, J = 15.82 Hz), 7.56 (d, 2H, H3′,5′, J = 7.43 Hz) 7.65 (d, 1H, H6′a, J = 8.91 Hz) 7.73 (d, 2H, H2′,6′, J = 6.18 Hz), 7.89 (d, 2H, Hβ, J = 15.58 Hz), 8.12 (s, 1H, H2′a); 13C NMR data: δ13C 55.19 (OCH3),55.69 (OCH3), 89.85 (C3″–5″), 104.89 (C1″), 120.02 (C3′–5′), 120.57 (C2′a), 121.32 (Cα), 124.11 (C6′a), 125.32 (C4′a), 130.23 (C5′a), 131.34 (C2′–6′), 131.85 (C1′), 139.03 (C1′a), 140.32 (Cβ), 140.42 (C3′a), 144.33 (C4′), 152.77 (CO(NH)2), 162.02 (C2″–6″), 162.25 (C4″), 188.53 (CO), 190.01 (CO); Anal. Calculated: C, 68.09%; H, 5.56%; N, 4.29%; O, 22.06%; Found: C, 68.22%; H, 5.34%; N, 4.13%; O, 21.96%.
1,1-Bis-[(3′,4′-N-(urenylphenyl)-3-(2″,4″,5″-trimethoxyphenyl)]-2-propen-1-one (10)
Yield 75%, mp. 145–147°C. 1H NMR: δH 3.81 (bs, 18H, −OCH3), 7.49 (d, 2H, Hα, J = 15.43 Hz), 7.63 (d, 2H, H3′,5′, J = 7.66 Hz), 7.88 (d, 1H, H6′a, J = 8.67 Hz), 7.87 (d, 2H, H2′,6′, J = 8.66 Hz), 8.00 (d, 2H, Hβ, J = 15.58 Hz), 8.14 (s, 1H, H2′a), 9.65 (bs, 2H, NH); 13C NMR: δ13C 55.49 (OCH3),55.69 (OCH3), 56.62 (OCH3), 99.35 (C3″), 115.89 (C1″), 116.58 (C6″), 119.96 (C3′–5′), 120.87 (C2′a), 123.32 (Cα), 124.37 (C6′a), 125.30 (C4′a), 130.14 (C5′a), 131.12 (C2′–6′), 131.85 (C1′), 138.22 (C1′a), 139.76 (Cβ), 140.33 (C3′a), 144.53 (C4′), 144.87 (C5″), 152.25 (C4″), 152.67 (CO(NH)2), 156.05 (C2″), 188.82 (CO), 189.98 (CO); Anal. Calculated: C, 68.09%; H, 5.56%; N, 4.29%; O, 22.06%; Found: C, 68.27%; H, 5.53%; N, 4.25%; O, 22.09%.
1,1-Bis-[(3′,4′-N-(urenylphenyl)-3-(phenyl)]-2-propen-1-one (11)
Yield 63%, mp. 210–212°C. 1H NMR: δH 7.43 (d, 2H, Hα, J = 15.58 Hz), 7.62 (d, 2H, H3′,5′, J = 8.41 Hz), 7.71 (d, 2H, H6′a, J = 7.92 Hz) 7.87 (d, 2H, H2′,6′, J = 8.66 Hz), 7.91 (d, 2H, Hβ, J = 15.58 Hz), 8.11 (s, 1H, H2′a), 9.69 (bs, 2H, NH); 13C NMR δ 120.34 (C3′–5′), 120.43 (Cα), 120.95 (C2′a), 124.21 (C6′a), 125.24 (C4′a), 128.75 (C4″), 128.92 (C2″–6″), 129.85 (C3″–5″), 130.07 (C5′a), 131.14 (C2′–6′), 131.98 (C1′), 134.17 (C1″), 139.33 (C1′a), 140.22 (C3′a), 144.33 (C4′), 146.62 (Cβ), 152.71 (CO(NH)2), 188.95 (CO), 190.01 (CO); CIMS (m/z) data 473 [M+H]; Anal. Calculated: C, 78.80%; H, 5.12%; N, 5.93%; O, 10.16%; Found: C, 78.51%; H, 5.31%; N, 5.63%; O, 10.18%.
1,1-Bis-[(3′,4′-N-(urenylphenyl)-3-(4″-fluorophenyl)]-2-propen-1-one (12)
Yield 94%, mp. 178–181°C 1H NMR: δH 7.29 (d, 2H, H3′,5′, J = 8.64 Hz), 7.49 (d, 2H, Hα, J = 15.82 Hz), 7.63 (d, 2H, H2′,6′, J = 8.66 Hz), 7.90 (d, 2H, Hβ, J = 15.81 Hz), 8.11 (d, 1H, H6′a, J = 8.40 Hz), 8.21 (s, 1H, H2′a), 9.56 (bs, 2H, NH); 13C NMR: δ13C 116.15 (C3″–5″), 119.24 (C3′–5′), 120.86 (Cα), 120.95 (C2′a), 124.44 (C6′a), 125.24 (C4′a), 130.07 (C5′a), 131.01 (C2″–6″), 131.10 (C2′–6′), 131.16 (C1″), 132.08 (C1′), 139.23 (C1′a), 140.37 (C3′a), 143.62 (Cβ), 144.30 (C4′), 152.71 (CO(NH)2), 164.25 (C4″), 188.95 (CO), 190.01 (CO); CIMS (m/z) data 509 [M+H]; Anal. Calculated: C, 73.22%; H, 4.36%; F, 7.47%; N, 5.51%; O, 9.44%; Found: C, 73.34%; H, 4.12%; F, 7.42%; N, 5.61%; O, 9.42%.
1,1-Bis-[(3′,4′-N-(urenylphenyl)-3-(2″,3″-difluorophenyl)]-2-propen-1-one (13)
Yield 98%, mp. 190–192°C. 1H NMR: δH 7.29 (d, 2H, H3′,5′, J = 7.89 Hz), 7.49 (d, 2H, Hα, J = 15.58 Hz), 7.69 (d, 2H, H2′,6′, J = 8.42 Hz), 7.94 (d, 2H, Hβ, J = 15.82 Hz), 8.11 (d, 1H, H6′a, J = 8.39 Hz), 8.19 (s, 1H, H2′a); 13C NMR: δ13C 118.35 (C4″), 119.54 (C3′–5′), 120.92 (C2′a), 121.66 (C6″), 123.94 (C5″), 124.21 (C6′a), 124.37 (C1″), 124.43 (Cα), 125.24 (C4′a), 126.61 (Cβ), 130.07 (C5′a), 131.15 (C2′–6′), 131.38 (C1′), 138.13 (C1′a), 140.34 (C3′a), 144.35 (C4′), 148.72 (C2″), 149.85 (C3″), 152.75 (CO(NH)2), 188.98 (CO), 190.00 (CO); CIMS (m/z) data 545 [M+H]; Anal. Calculated: C, 68.38%; H, 3.70%; F, 13.96%; N, 5.14%; O, 8.81%; Found: C, 68.17%; H, 3.78%; F, 14.03%; N, 5.22%; O, 8.85%.
1,1-Bis-[(3′,4′-N-(urenylphenyl)-3-(4″-chlorophenyl)]-2-propen-1-one (14)
Yield 90%, mp. 206–208°C. 1H NMR d: δH 7.39 (d, 2H, Hα, J = 15.21 Hz) 7.51 (d, 2H, H3′,5′, J = 8.16 Hz), 7.69 (d, 2H, H6′a, J = 8.91 Hz), 7.70 (d, 2H, H2′,6′, J = 8.16 Hz), 8.11 (d, 2H, Hβ, J = 15.82 Hz) 8.19 (s, 1H, H2′a), 10.00 (bs, 2H, NH); 13C NMR: δ13C 120.07 (C3′–5′), 121.00 (Cα), 121.15 (C2′a), 124.31 (C6′a), 125.32 (C4′a), 138.21 (C4″), 129.62 (C2″–6″), 130.04 (C3″–5″), 130.16 (C5′a), 131.08 (C2′–6′), 131.98 (C1′), 134.01 (C1″), 141.03 (C1′a), 140.46 (C3′a), 144.14 (C4′), 143.06 (Cβ), 152.71 (CO(NH)2), 189.03 (CO), 190.01 (CO); CIMS (m/z) data 541 [M+H], 542 [M+2]; Anal. Calculated: C, 68.77%; H, 4.10%; Cl, 13.10%; N, 5.17%; O, 8.86%; Found: C, 68.56%; H, 4.23%; Cl, 13.16%; N, 5.21%; O, 8.90%.
1,1-Bis-[(3,4-N-(urenylphenyl)-3-(4″-methoxyphenyl)]-2-propen-1-one (15)
Yield 98%, mp. 179–180°C. 1H NMR: δH 3.81 (s, 6H, -OCH3), 7.01 (d, 2H, H3′,5′, J = 7.41 Hz), 7.49 (d, 2H, Hα, J = 15.58 Hz), 7.69 (d, 2H, Hβ, J = 15.82 Hz), 7.70 (d, 1H, H6′a, J = 8.66 Hz), 8.10 (d, 2H, H2′,6′, J = 8.41 Hz), 8.19 (s, 1H, H2′a), 9.86 (bs, 2H, NH); 13C NMR: δ13C 55.54 (4″-OCH3), 120.16 (C3′–5′), 120.63 (Cα), 120.96 (C2′a), 124.18 (C6′a), 125.21 (C4′a), 163.35 (C4″), 130.92 (C2″–6″), 112.89 (C3″–5″), 130.10 (C5′a), 131.10 (C2′–6′), 132.38 (C1′), 128.17 (C1″), 139.23 (C1′a), 140.42 (C3′a), 143.62 (Cβ), 144.35 (C4′), 152.71 (CO(NH)2), 188.90 (CO), 190.03 (CO); CIMS (m/z) data 533 [M+H]; Anal. Calculated: C, 74.42%; H, 5.30%; N, 5.26%; O, 15.02%; Found: C, 74.51%; H, 5.24%; N, 5.32%; O, 14.99%.
1,1-Bis-[(3′,4′-N-(urenylphenyl)-3-(3″,4″-methylendioxyphenyl)]-2-propen-1-one (16)
Yield 94%, mp. 181–183°C. 1H NMR: δH 6.09 (s, 4H, −OCH2O−), 6.97 (d, 2H, H3′,5′, J = 8.16 Hz), 7.49 (d, 2H, Hα, J = 15.82 Hz), 7.68 (s, 1H, H6′a, J = 7.88 Hz), 7.77 (d, 2H, Hβ, J = 15.83 Hz), 8.10 (d, 2H, H2′,6′, J = 8.41 Hz), 8.19 (s, 1H, H2′a), 9.88 (bs, 2H, NH); 13C NMR: δ13C 101.79 (OCH2O), 105.68 (C5″), 110.02 (C2″), 119.71 (C3′–5′), 120.95 (C2′a), 123.55 (C6″), 123.65 (Cα), 124.39 (C6′a), 125.27 (C4′a), 128.86 (C1″), 130.18 (C5′a), 131.13 (C2′–6′), 132.17 (C1′), 138.99 (C1′a), 140.44 (C3′a), 143.86 (C4′), 144.22 (Cβ), 149.88 (C3″), 150.46 (C4″), 152.77 (CO(NH)2), 188.90 (CO), 190.00 (CO); CIMS (m/z) data 561 [M+H]; Anal. Calculated: C, 70.71%; H, 4.32%; N, 5.00%; O, 19.98%; Found: C, 70.87%; H, 4.11%; N, 4.95%; O, 19.77%.
1,1-Bis-[(3′,4′-N-(urenylphenyl)-3-(4″-methylphenyl)]-2-propen-1-one (17)
Yield 98%, mp. 220–222°C. 1H NMR: δH 3.41 (s, 6H, 4-OCH3)7.27 (d, 2H, H3′,5′, J = 7.92 Hz), 7.49 (d, 2H, Hα, J = 15.82 Hz), 7.68 (d, 1H, H6′a, J = 7.42 Hz), 7.90 (d, 2H, Hβ, J = 15.34 Hz), 8.11 (d, 2H, H2′,6′, J = 8.41 Hz), 8.21 (s, 1H, H2′a), 9.94 (bs, 2H, NH); 13C NMR: δ13C 22.54 (4″-CH3), 119.69 (C3′–5′), 120.69 (Cα), 120.76 (C2′a), 124.68 (C6′a), 125.31 (C4′a), 129.87 (C3″–5″), 130.02 (C2″–6″), 130.23 (C5′a), 131.08 (C2′–6′), 132.27 (C1′), 133.16 (C1″), 138.93 (C1′a), 140.56 (C3′a), 141.35 (C4″), 144.16 (C4′), 144.72 (Cβ), 152.69 (CO(NH)2), 188.90 (CO), 190.01 (CO); CIMS (m/z) data 501 [M+H]; Anal. Calculated: C, 79.18%; H, 5.64%; N, 5.60%; O, 9.59%; Found: C, 79.23%; H, 5.54%; N, 5.65%; O, 9.37%.
Parasite and experimental host
Male NIH mice, weighing 18–22 g were maintained on a commercial pellet diet and housed under conditions approved by Ethics Committee. Plasmodium berghei (ANKA strain), a rodent malaria parasite, was used for infection. Mice were infected by ip passage of 1 × 106 infected erythrocytes diluted in phosphate-buffered saline solution (PBS, 10 mM, pH 7.4, 0.1 mL). Parasitemia was monitored by microscopic examination of Giemsa-stained smears.
Parasite extracts
Blood of infected animals, at a high level of parasitemia (30–50%), was collected by cardiac puncture with a heparinised syringe, and the blood pool was centrifuged (500 g × 10 min, 4°C). Plasma and Buffy coat were removed, and the red blood cells (RBC) pellet was washed twice with chilled PBS-Glucose (5.4 %). The washed pellet was centrifuged on a discontinuous percoll gradient (80–70% percoll in PBS-Glucose, 20.000 g × 30 min × 4°CCitation21). The upper band (mature forms) was removed by aspiration, collected in Eppendorf tubes and washed twice with chilled PBS-glucose, and the infected erythrocytes were lysed with the nonionic detergent saponin (0.1% in PBS × 10 min). Cold PBS (1 mL) was added and the samples were centrifuged (13.000 g × 5 min, 4°C) and washed three times to remove erythrocyte cytoplasm content (including erythrocyte hemoglobin). The free parasites were placed in PBS-Glucose (5.4 %), and subjected to three freeze-thaw cycles (−70°C/+37°C).
Mice native hemoglobin
Native hemoglobin from noninfected mice was obtained by treating one volume of pellet erythrocytes with two volumes of water. The resulting solution was used as the substrate in the inhibition of the hemoglobin hydrolysis assay.
Inhibition of heme polymerization
The heme polymerization assay was performed according toCitation22, briefly, a solution of hemin chloride (50 µL, 4 mM), dissolved in dimethyl sulfoxide (DMSO) (5.2 mg/mL), was distributed in 96-well micro plates. Different concentrations (100–1 mM) of the compounds dissolved in DMSO were added in triplicate in test wells (50 µL) with a final concentration of 25 mM–125 µM/well. Controls contained either water (50 µL), chloroquine (2.5 mM–125 µM/well, 50 µL) or DMSO (50 µL). β-Hematin formation was initiated by the addition acetate buffer (100 µL 0.2 M, pH 4.4). Plates were incubated at 37°C for 48 h to allow completion of the reaction and centrifuged (4000 RPM × 15 min, IEC-CENTRA, MP4R). After discarding the supernatant, the pellet was washed twice with DMSO (200 µL) and finally, dissolved in NaOH (200 µL, 0.2 N). The solubilized aggregates were further diluted 1:2 with NaOH (0.1 N) and absorbance recorded at 405 nm (Microplate Reader, BIORAD-550). The results were expressed as a percentage of inhibition of flavoprotein (FP) polymerization ().
Table 1. Percentage of inhibition of heme polymerization and FACS.
Hemoglobin hydrolysis assay
The proteolytic effect of the parasite extract on the native mice haemoglobin was assayed using 96-wells plates (Greiner Bio-One). The assay mixture contained mice native hemoglobin (10 µL), parasite extract (50 µL), GSH (10 µL, 10 µM) and acetate buffer (0.2 M, pH 5.4) to a final volume of 200 µL. Compounds, chloroquine, leupeptin and pepstatin (5 mM) were incorporated in the incubation mixture dissolved in DMSO. The incubations were carried out at 37°C for 18 h and the reactions were stopped by addition of reduced sample buffer. The degree of digestion was evaluated electrophoretically by SDS-PAGECitation23 by visual comparison the globin bands (14 kDa). A DMSO control was electrophoresed at the same time. Once we get the bands, the densitometer registers the band density values, and they were expressed as intensity/mm2 ± SD, so we proceed to check the densities of them in order to have a percentage of inhibition of hemoglobin hydrolysis.
Activity against murine malaria and data analysis
NIH mice (18e23 g) were infected intraperitoneal with 106 P. berghei-infected RBC. Treatment began 2 h after infection. These compounds were dissolved in DMSO (0.1 M), diluted with saline Tween 20 solutions (2%). Each compound (20 mg/kg) was administered once daily for 4 days. At day 4, the parasitemia was counted by examination of Giemsa-stained smears. Chloroquine (25 mg/kg) was used as a positive control. The survival time beyond the control group (without drug treatment) was recorded. The results were expressed as percentage of parasitemia in relation to the control (percentage of parasitemia) and percentage of survival miceCitation24. Data were statistically analyzed using one way ANOVA and t-tests for specific group comparisons; assuming 95% of confidence according Graph Pad Prism 3.02. The densitometer registers the band density values and it reports as intensity/mm2 ± SD. SEM was calculated as basis of number of pixels (n = 400).
Results and discussion
One compound 5 that inhibited parasite development was evaluated in vitro assay for the inhibition of globin hydrolysis, and trophozoyte-rich extract was used to digest the native hemoglobin of mice. The electrophoretic analysis indicated that this compound was effectively inhibiting the degradation of hemoglobin more than 90% as the pepstatin control does (intact band at 14 kDa) and even better than leupeptin control in a significant way ( and ).
Figure 1. Effects on globin hydrolysis of chalcone derivate. The samples were solubilized in SDS-sample buffer containing β-mercapto ethanol and boiled before electrophoresis in 15% SDS-PAGE gels. The gels were stained with Coomassie blue. The position of MW standard is shown in kDa. Undergraded globin appears at 14 kDa. A, control human hemoglobin; B, control hemoglobin, without enzyme; C, control, enzyme with hemoglobin; 5, compound (100 mM); MW, molecular weight; LEP, leupeptin (100 mM); PEP, pepstatin (100 mM).
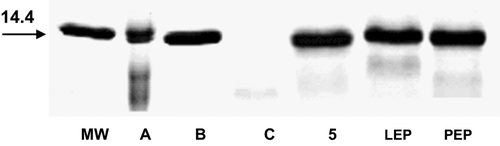
Figure 2. Percentage of inhibition of hemoglobin hydrolysis. The results are expressed by the media ± SEM. Compound 5 compared with LEP and PEP. *p < 0.01 compared with LEP. LEP, leupeptin; PEP, pepstatin.
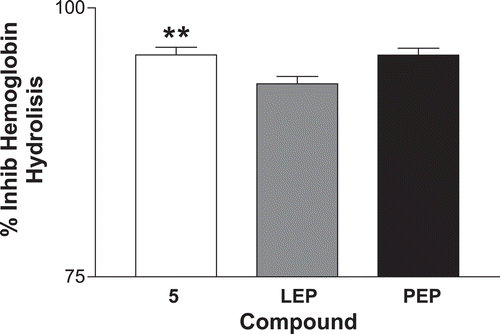
Compound 5, identified as the most active by in vitro tests, it was tested in mice infected with P. berghei (ANKA), a chloroquine-susceptible strain of murine malaria. The mice were treated with compound 5 (20 mg/kg, ip once daily) and chloroquine (25 mg/kg, ip once daily) for 4 consecutive days (days 0–4 postinfection), and their survival times and parasitemia at 4th day were monitored and compared with control mice receiving saline (untreated mice). Evaluating the parasitemia, we noticed an important decrease and 5-treated mice comparing with saline-treated mice (p < 0.05), so this compound was able to reduce the level of parasites in blood at 4th day after infection in a significant way (). Control mice died between 9 and 11 days after P. berghei infection, and compound 5 increased the survival time they died between days 6–19 after infection. This compound was able to reduce the parasitemia and delay the progression of malaria but did not eradicate the infection. A different regimen of drug treatment might be more effective in order to get best results.
Acknowledgments
The study was supported by Consejo de Desarrollo Científico y Humanístico Universidad Central de Venezuela. CDCH, Grants PI 06-7477-2009, Universidad Pedagógica Experimental Libertador, Instituto Pedagógico de Caracas. CICNAT No. 09-066.
Declaration of interest
The authors report no conflicts of interest.
References
- Schlitzer M. Malaria chemotherapeutics part I: History of antimalarial drug development, currently used therapeutics and drugs in clinical development. ChemMedChem 2007;2:944–986.
- Wiesner J, Ortmann R, Jomaa H, Schlitzer M. New antimalarial drugs. AngewChemInt Ed 2003;42:5274–5293.
- Talisuma AO, Bloland P, Alessandro UD. History dynamic and public health importance of malaria resistance. ClinMicrobiol Rev 2004;17:236–242.
- World Health Organization. World Malaria Report 2009. Geneva, Switzerland: WHO.
- Jana S, Paliwal J. Novel molecular targets for antimalarial chemotherapy. Int J Antimicrob Agents 2007;30:4–10.
- Wernsdorfer WH, Payne D. The dynamics of drug resistance in Plasmodium falciparum. Pharmacol Ther 1991;50:95–121.
- Zhang K, Rathod PK. Divergent regulation of dihydrofolate reductase between malaria parasite and human host. Science 2002;296:545–547.
- Nielsen SF, Christensen SB, Cruciani G, Kharazmi A, Liljefors T. Antileishmanial chalcones: statistical design, synthesis, and three-dimensional quantitative structure-activity relationship analysis. J Med Chem 1998;41:4819–4832.
- Liu M, Wilairat P, Go ML. Antimalarial alkoxylated and hydroxylated chalcones [corrected]: structure-activity relationship analysis. J Med Chem 2001;44:4443–4452.
- Hayat F, Moseley E, Salahuddin A, Van Zyl RL, Azam A. Antiprotozoal activity of chloroquinoline based chalcones. Eur J Med Chem 2011;46:1897–1905.
- Hsieh HK, Lee TH, Wang JP, Wang JJ, Lin CN. Synthesis and anti-inflammatory effect of chalcones and related compounds. Pharm Res 1998;15:39–46.
- Araico A, Terencio MC, Alcaraz MJ, Domínguez JN, León C, Ferrándiz ML. Phenylsulphonyl urenyl chalcone derivatives as dual inhibitors of cyclo-oxygenase-2 and 5-lipoxygenase. Life Sci 2006;78:2911–2918.
- Sivakumar PM, Geetha Babu SK, Mukesh D. QSAR studies on chalcones and flavonoids as anti-tuberculosis agents using genetic function approximation (GFA) method. Chem Pharm Bull 2007;55:44–49.
- Barford L, Kemp K, Hansen M, Kharazmi A. Chalcones from Chinese liquorice inhibit proliferation of T cells and production of cytokines. IntImmunopharmacol 2002;2:545–555.
- Rodrigues J, Abramjuk C, Vásquez L, Gamboa N, Domínguez J, Nitzsche B et al. New 4-maleamic acid and 4-maleamide peptidyl chalcones as potential multitarget drugs for human prostate cancer. Pharm Res 2011;28:907–919.
- Dominguez JN, Leon C, Rodrigues J, Gamboa de Dominguez N, Gut J, Rosenthal PJ. Synthesis of chlorovinyl sulfones as structural analogs of chalcones and their antiplasmodial activities. Eur J Med Chem 2009;44:1457–1462.
- Domínguez JN, León C, Rodrigues J, Gamboa de Domínguez N, Gut J, Rosenthal PJ. Synthesis and evaluation of new antimalarial phenylurenyl chalcone derivatives. J Med Chem 2005;48:3654–3658.
- Guantai EM, Ncokazi K, Egan TJ, Gut J, Rosenthal PJ, Bhampidipati R et al. Enone- and chalcone-chloroquinoline hybrid analogues: in silico guided design, synthesis, antiplasmodial activity, in vitro metabolism, and mechanistic studies. J Med Chem 2011;54:3637–3649.
- Bukvic Krajacic M, Peric M, Smith KS, Schönfeld ZI, Žiher D, Fajdetic A et al. Synthesis, structure-activity relationship, and antimalarial activity of ureas and thioureas of 15-membered azalides. J Med Chem 2011;54:3595–3605.
- Li R, Kenyon GL, Cohen FE, Chen X, Gong B, Dominguez JN et al. In vitro antimalarial activity of chalcones and their derivatives. J Med Chem 1995;38:5031–5037.
- Deharo E, Gautret P, Ginsburg H, Chabaud AG, Landau I. Synchronization of Plasmodium yoelii nigeriensis and P. y. killicki infection in the mouse by means of Percoll-glucose gradient stage fractionation: determination of the duration of the schizogonic cycle. Parasitol Res 1994;80:159–164.
- Solomon VR, Haq W, Smilkstein M, Srivastava K, Puri SK, Katti SB. 4-Aminoquinoline derived antimalarials: synthesis, splasmodial activity and heme polymerization inhibition studies. Eur J Med Chem 2010;45:4990–4996.
- Rosenthal PJ. Plasmodium falciparum: effects of proteinase inhibitors on globin hydrolysis by cultured malaria parasites. Exp Parasitol 1995;80:272–281.
- Peters W, Robinson BL. (1999). Malaria. In: Zak O, Sande MA, eds. Handbook of Animal Models of Infection. San Diego, CA, USA: Academic Press, 757–773.