Abstract
Context: Characterization of the pro-oxidant activity of QNACR.
Objectives: Reactive oxygen species (ROS) induce cellular damage and represent unique opportunities to kill malignant cells. In this study, we synthesized and evaluated the new compound, (E)-methyl 2-(7-chloroquinolin-4-ylthio)-3-(4-hydroxyphenyl) acrylate (QNACR) as potential pro-oxidative agent against breast cancer.
Methods: Oxidative stress biomarkers such as ROS, thiobarbuturic acid reactive species (TBARs) and different antioxidant enzyme activities were determined in cell lysates.
Results: QNACR showed cytotoxic and more selective effects to tumour MCF7 cells (IC50 < 25 µM) compared to antitumour controls, inducing ROS and TBARs parallel to inhibitions of catalase (CAT), glucose-6-phosphate dehydrogenase (G6PDH) and 6-phosphogluconate dehydrogenase (6PGDH). Longer exposures to QNACR triggered adaptive effects increasing the overall activities of CAT, glutathione reductase, G6PDH and 6PGDH, but eventually the adaptation changes faded and cells died.
Conclusion: QNACR led to remarkable modifications in the oxidative status of tumour cells, proposing this compound as potential alternative for antitumour therapy.
Introduction
The search of new compounds targeting diverse human cancers represents an important key combating this disease. Over the last years, there has been a strong interest in the development of new heteroaromatic compounds derived from quinolines due to their wide range of pharmacological properties. Indeed, quinoline derivatives have shown effects as antineoplastics such as tasquinimod (ABR-215050) which is one of the main quinoline compounds that is currently in clinical research whose effects are related to the inhibition of angiogenesis in patients with tumour metastasisCitation1. On the other hand, substitutions with an acrylate group on organic structures such as benzofuranes improves the antitumour activity in vitroCitation2, being proposed as potential DNA-intercalating compounds, inhibitors of angiogenesis and apoptosis inducersCitation3,Citation4 confirming the multi-target effects of these structures.
Reactive oxygen species (ROS) are well known to produce oxidative stress and cause damage to lipids, DNA and other cellular molecules in vitro and in vivo. These effects could be taken for the design and development of new possible structures as antineoplastics and could be related to a direct/indirect inhibition of antioxidant enzymes or by chemical production of ROS inside the cells. Indeed, the therapeutic potential of abietyl-Isothiocyanate (ABITC) to treat endometrial cancer is related to its actions on cell-cycle progression and apoptosis as well as on the ROS generationCitation5.
Pro-oxidative compounds are being considered as potential novel antitumour therapies. Indeed, (−)-Epigallocatechin-3-gallate (EGCG), the major polyphenol in green tea, has been shown to inhibit tumourigenesis and cancer cell growth in animal models by a time-dependent production of intracellular ROS, effect that was reversed in the presence of superoxide dismutase and catalaseCitation6.
In this study, we characterized the dose and time–response relationship of the new quinoline acrylate compound (E)-Methyl 2-(7-chloroquinolin-4-ylthio)-3-(4 hydroxyphenyl) acrylate (QNACR) in human breast cancer cells, as well as its involvement on the oxidative status in vitro.
Methods
Synthesis of (E)-methyl 2-(7-chloroquinolin-4-ylthio)-3-(4 hydroxyphenyl) acrylate (QNACR)
The synthesis and physicochemical evaluations of QNACR () were performed according to a previous procedureCitation7. Briefly, the melting point was determined on a Thomas micro hot stage apparatus and infrared spectra as KBr pellets on a Shimadzu model 470 spectrophotometer. The 1H NMR, 13C NMR spectra were recorded using a Jeol Eclipse 270 (270 MHz/67.9 MHz) spectrometer using DMSO-d6, and are reported in ppm downfield from the residual DMSO. Elemental analyses were performed on a Perkin Elmer 2400 CHN analyzer, results were within ±0.4% of the predicted values for all compounds. Chemical reagents were obtained from Aldrich Chemical Co, USA. All solvents were distilled and dried in the usual manner.
Figure 1. Chemical structure of (E)-methyl 2-(7-chloroquinolin-4-ylthio)-3-(4 hydroxyphenyl) acrylate (QNACR).
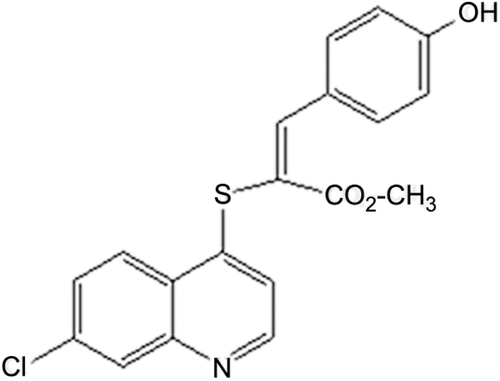
A mixture of methyl 2-(7-chloroquinolin-4-ylthio) acetate (0.37 mmol), appropriate benzaldehyde (0.45 mmol), piperidine 0.02 mL, glacial acetic acid (0.05 mL) in benzene dry (10 mL) was refluxed overnight. After cooling, the mixture was washed with a solution of HCl 0.1 M (3 times), water (3 times), brined and dried over MgSO4, filtered, and concentrated. The crude product was purified by flash chromatography n-hexane:ethyl acetate (7:3).
Cell cultures
Breast adenocarcinoma cell line (MCF7) and non-tumour retinal pigment epithelium (RPE) cells were obtained from European Collection of Cell Cultures, Salisbury Wiltshire, UK. Cells were grown in RPMI medium supplemented with 10% fetal bovine serum (FBS), L-glutamine 1%, penicillin (50 units/mL) and streptomycin (50 µg/mL), in a humidified atmosphere of 95% air and 5% CO2 at 37°C. Cell culture medium was from HyClone Laboratories, Inc. South Logan, Utah, USA. Reagents were purchased from Sigma-Aldrich (St. Louis, MO).
Effect of QNACR on cell viability and cell growth
5 × 103 MCF7 or 5 × 103 RPE cells were cultured in a 96-well microtiter plate containing 0.1 mL of RPMI growth medium/well for 24 h. Cells were incubated with the compound dissolved in dimethylsulfoxide (DMSO)/medium 0.02% (5–100 µg/mL, 72 h) and evaluated for their cytotoxicity. The dose-dependent effects of each compound on cell viability were assessed using the Alamar Blue test (Invitrogen®) according toCitation7 and the IC50 values obtained were defined as the concentration of tested compounds resulting in a 50% reduction of the cell viability compared to vehicle-treated cells. Further evaluations of QNACR were performed using its IC50 value. The time-dependent effects of this compound on cell growth were measured according to a previous procedureCitation8. All experiments were carried out in triplicates.
Effect of antioxidants on QNACR cytotoxicity
5 × 103 MCF7 cultured for 24 h in a 96-well microtiter plate containing 0.1 mL of RPMI growth medium/well were incubated with either vehicle, QNACR at its cytotoxic IC50, N-acetyl-Cysteine (0.5 mM) or TROLOX (0.1 mM) in different incubation combinations (37°C × 72 h). Cells were then incubated with Alamar Blue (37°C × 4 h) and resorufin was measured at 570/600 nm. The results were expressed as percentage of cell viability compared to control vehicle.
Intracellular ROS determinations
The determination of intracellular reactive oxygen species (ROS) after QNACR incubations over the time was measured with the non-fluorescent probe 2′,7′-dichlorofluorescein diacetate (DCFH-DA) as described previouslyCitation9, measuring the fluorescence of DCFH at 480/530 nm.
Sample preparation and compound incubations
For the determination of lipid peroxidation, enzyme activities and total glutathione, 2 × 106 MCF7 cells were treated either with vehicle or QNACR at its cytotoxic IC50 (37°C × 3, 6, 12 and 24 h), were trypsinized and 1 × 106 cells were suspended in PBS and centrifuged (1000 rpm × 5 min). Samples were subjected to different protocols, depending on the enzyme activity/metabolite determinationsCitation9.
Lipid peroxidation evaluation
To determine lipid peroxidation induction by the compound, we measured the production of thiobarbituric reactive oxygen species TBARs at 535/580 nmCitation10. Results are expressed as nmol TBARs/mg.
Activities of superoxide dismutase and catalase
Superoxide dismutase (SOD) and catalase (CAT) activities were measured by tracking the reduction of cytochrome C at 550 nm and of H2O2 at 240 nm, respectively, following previous protocolsCitation9. For SOD, results were expressed as U/mL·mg, while for CAT activity results were expressed as U/mg.
Glutathione cycle determinations
To determine the total glutathione levels in cells treated with the compound, we followed a previously modified protocolCitation9. To evaluate the glutathione reductase activity (GR), we followed a modification of a procedure previously reportedCitation11. For glutathione-S-transferase (GST) activity, we followed a procedure previously describedCitation12.
Activities of dehydrogenase enzymes from the hexose-phosphate pathway
To determine the activities of glucose-6-phosphate dehydrogenase (G6PDH) and 6-phosphogluconate dehydrogenase (6PGDH), we followed a microplate modification of a procedure previously describedCitation13, measuring the NADPH fluorescence detected as 460 nm emission excited at 340 nm. To obtain the accurate activities of both enzymes, 6PGDH and total dehydrogenase activity (G6PDH + 6PGDH) were measured separately. G6PDH activity was calculated by subtracting the activity of 6PGDH from total enzyme activityCitation9.
Protein levels were determinedCitation14, and data were presented as the average of four independent experiments (n = 4) and tested for statistical significance using unpaired t-tests for specific group comparisons and ANOVA for multiple data sets, assuming 95% confidence limits using GraphPad Prism 4.02 software.
Results
A new quinoline acrylate derivative, the (E)-methyl 2-(7-chloroquinolin-4-ylthio)-3-(4 hydroxyphenyl) acrylate (QNACR, ) was synthesized and evaluated for its potential antineoplastic activity in vitro. The parameters of QNACR purity and structure confirmation were as follows:
Yellow solid. Yield 75%. mp. 210–212°C. IR (KBr) cm−1: 3456 (OH), 1698 (CO), 1601 (C=C). 1H NMR, DMSO-d6, δ ppm: 3.69 (s, 3H, OCH3); 6.79 (d, 2H, H3`,5´, J: 8.9 Hz); 7.09 (d, 1H, H3, J: 5.0 Hz); 7.73 (dd, 1H, H6, J: 9.1, 2.2 Hz); 7.87 (d, 2H, H2´, 6´, J: 8.9 Hz); 8.09 (d, 1H, H8, J: 2.2 Hz); 8.22 (d, 1H, H5, J: 9.1 Hz); 8.49 (s, 1H, =CH); 8.66 (d, 1H, H2, J: 5.0 Hz); 10.33 (s, 1H, OH). 13C NMR, DMSO-d6, δ ppm: 53, 114, 116, 117, 121, 125, 126, 128, 129, 134, 135, 139, 146, 148, 152, 153, 161. Anal. Calcd. for C19H14ClNO3S. C: 61.37, H: 3.79, N: 3.77. Found C: 61.36, H: 3.73, N: 3.61.
The evaluation of this compound on cell viability in the cell lines demonstrated that QNACR is cytotoxic to tumour MCF7 cells in a dose-dependent manner, showing an IC50 < 25 µM. The results also remarked the more toxic effect on this cell line compared to non-tumour cells and additionally, demonstrating a higher specificity rate compared to cisplatin and doxorubicin controls (). In this context, the effect of QNACR on cell viability also showed a time-dependency fashion (n = 3, p < 0.01; Supplemental Figure 1).
Table 1. Comparison of the cytotoxic effect of QNACR with cisplatin and doxorubicin.
This cytotoxic effect was partially reversed by addition of two functionally unrelated antioxidants N-acethyl-cysteine (NAC), a compound that reduces thiol groups and is a substrate for the synthesis of glutathione, and trolox, a vitamin E water-soluble analogue that scavenges free radicals. This suggests the involvement of increased oxidative stress in the cytotoxic actions of QNACR ().
Figure 2. Effect of antioxidants on the cytotoxicity of QNACR (24.8 µM) on MCF7 cells. NAC (0.5 mM) and Trolox (0.1 mM) themselves resulted in the cell viability of 99.75 ± 2.2% and 103.12 ± 2.67, respectively, compared to untreated control. Results are expressed as the mean ± SEM. ***p < 0.001.
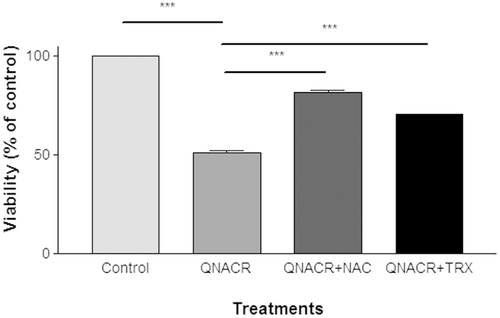
In the next step, we investigated whether QNACR increases oxidative markers in MCF7 cells. Thus, we used the oxidant-sensitive fluorescence probe DCFH-DA to determine ROS levels. As shown in , this quinoline acrylate compound significantly increased the reactive oxygen species compared to control vehicle over the time, suggesting that the toxic effects of QNACR might be due to an oxidative burst on tumour cells. To confirm the oxidative effect of QNACR, we determined lipid peroxidation in these tumour cells. Results showed that this compound increased TBARs levels in a time-dependent manner ().
Figure 3. Effect of QNACR (24.8 µM) on oxidative markers in MCF7 cells over the time. a) Reactive-oxygen species (ROS) levels. b) Thiobarbituric acid reactive species (TBARs) levels. Results are expressed as the mean ± SEM. (a) *p < 0.05 and ***p < 0.001. (b) ***p < 0.001 compared to control vehicle.

Defence against oxidative stress represents a very important pathway for the detoxification of free radicals and a compound able to increase ROS in tumour cells could modify the different antioxidant mechanisms, making the cells more vulnerable to the oxidant species and resulting in cell death. The effects of QNACR on the several antioxidant enzymes are shown in . SOD and CAT by removing superoxide radical and the oxidant hydrogen peroxide respectively, constitute a first-line of defence against ROS. SOD activity was slightly affected by QNACR while CAT was strongly affected and interestingly in a non-monotonous manner: at 3–6 h occurred a 50% inhibition, at 12 h a two-fold increase, and at 24 h a near 50% inhibition. This non-monotonous behaviour was observed for many of the enzymes studied (see below) indicating that in these studies it is critical to perform time-dependent studies.
Table 2. Effect of QNACR (24.8 µM) on different antioxidant parameters over the time in breast cancer MCF7 cells.
The glutathione system is the most important redox buffer essential for maintaining the redox balance on cells. Thus, the modification of the glutathione cycle in MCF7 cells, as shown in , is highly relevant. The activity of glutathione reductase (GR) was strongly increased after 12 h of incubation, as it occurred with catalase. Detoxification enzyme GST was initially increased by 15%, however decreased by 25% after 24 h. The results on the glutathione-dependent enzymes are paralleled with an initial 30% decrease followed by 50% increase in the total glutathione levels ().
The pentose phosphate pathway (PPP) is a main route for glucose catabolism and biosynthesis of nucleic acids and aromatic aminoacids. In an oxidative phase, the PPP generates nicotinamide adenine dinucleotide phosphate (NADPH), by the enzymes glucose-6-phosphate dehydrogenase (G6PDH) and 6-phosphogluconate dehydrogenase (6PGDH). NADPH is the main intracellular reductant that is required for the normal function of the fatty acid synthesis and antioxidant systems such as the glutathione and thioredoxin cycles. Activities of G6PDH and 6PGDH were affected by QNACR due to overall decreases in short-time incubations and strong increases after longer periods of time compared to untreated controls ( and ). Of notice, the 60% increase in the activity of 6PGDH ().
Figure 4. Effect of QNACR (24.8 µM) on the activity of the dehydrogenase enzymes of the penthose phosphate pathway. (a) G6PDH: glucose-6-phosphate dehydrogenase activity; (b) 6PGDH; 6-phosphogluconate dehydrogenase activity. Results are expressed as the mean ± SEM. *p < 0.05; **p < 0.01 and ***p < 0.001 compared to control vehicle.
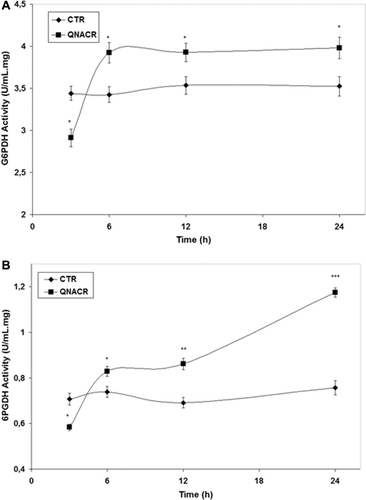
Discussion
Breast cancer represents the most common cause of cancer death among women worldwide while the incidence of and mortality rates are higher in more developed countries, rates in less developed countries are increasingCitation15 and since available therapies are limited, expensive and with remarkable toxicological effects, new alternative drugs are needed to fight this disease.
The effects of quinolines and acrylates as potential anticancer agents have been previously demonstratedCitation3,Citation4; however, there are few data available about the effect of these combined structures on tumour cells. It has been recently reported the potential antitumour properties of different quinoline acrylate derivativesCitation7, and especial attention has been paid to (E)-Methyl 2-(7-chloroquinolin-4-ylthio)-3-(4-hydroxyphenyl) acrylate (QNACR), due to its ability to inhibit cell adhesion, migration, invasion, angiogenesis matrix metalloproteases in vitro and tumour growth in vivoCitation7. Thus, in the present study we addressed the cytotoxic and pro-oxidative effects of QNACR in breast cancer cells.
Preferential killing of tumour cells (therapeutic selectivity) is one of the most important considerations in developing new cancer chemotherapies. Results of this study showed a more specific cytotoxic response of QNACR in MCF7 compared to non-tumour RPE cells with an increased rate of specificity compared to cisplatin and doxorubicin controls. Our results also showed that the effects of this compound on the cell lines are dose and time-dependent.
Previous evidences have suggested that neoplastic cells are under increased oxidative stress associated to a generation of reactive oxygen species (ROS)Citation16. Since ROS are prone to induce cellular damage, they are considered an unique opportunity to kill malignant cells based on their susceptibility to lead to oxidative insults (Supplemental, Figure 2a). Further exogenous ROS damage by potential antitumour agents may increase the ROS stress to a point that triggers cell death with therapeutic implicationsCitation9. In this context, QNACR was able to induce ROS levels over the time suggesting that the toxicological effects of this compound could be due to the induction of oxidative stress. To confirm that the cytotoxic actions of QNACR involved the ROS production, we also demonstrated that two unrelated antioxidant compounds such as NAC and TROLOX protected cells from the toxic effects of this compound.
Due to the highly reactive nature of ROS, increased generation of these species in tumour cells often leads to the accumulation of oxidative products, such as lipid peroxides. The increase of ROS levels by QNACR is indeed associated with higher levels of TBARs over the time, confirming the damage effects of this compound on cell membranes as we also previously reported with a benzothiazole compoundCitation9 and once lipid peroxidation is initiated, it would form membrane damage end products resulting in a possible decrease in membrane fluidity and increase in the membrane permeability, contributing to apoptosisCitation17.
The oxidative status regulates the expression and activity of superoxide dismutase (SOD), catalase (CAT), and glutathione-S-transferase (GST), as well as other antioxidant enzymes, leading to adaptive effects of these proteins. In this context, adaptation is an event in which oxidants triggered an antioxidant response in the cells resulting in higher levels of antioxidant and repair enzymes and lower membrane permeability, making cells more resistant to subsequent doses of oxidantsCitation18. Thus, QNACR showed non-monotonous effects in several enzymes revealing a complex response. Most enzymes analysed were inhibited within the first 3 h of incubation, which may be consistent with a damage exerted by ROS-derived QNACR in an acute oxidative challenge (Supplemental, Figure 2b). In fact, cellular membranes were also damaged within this short time window. Since the function of G6PDH and 6PGDH is to lead the production of NADPH for antioxidant defence, we hypothesized that the decrease in both enzyme activities during the beginning of QNACR effects may contribute to a decreased antioxidant defence that is exacerbated by the inhibition of CAT and thus leading to an accumulation in ROS in this acute initial phase. The dehydrogenase enzymes are possible targets for anticancer therapy and could lead to a profound decrease in the total glutathione level enhancing cellular damage. Thus, the initial inhibition of G6PDH and 6PGDH activities by QNACR compared to vehicle support the anticancer potential of this compound.
Two exceptions to the initial decrease at 3 h were a slight increase of SOD activity and an increase in GST activity. GST is an important detoxification enzyme, and QNACR being a xenobiotic may have triggered an immediate detoxification response. In addition, QNACR may have produced strong toxicants since ROS and TBARs are greatly elevated from the beginning of incubations, these toxicants could represent other types of compounds that need to be detoxified by GST, using large amounts of glutathione and suggesting that the levels of this free metabolite is decreased when GST in strongly increased, as we have observed in this study.
After 3 h, QNACR increased the activity of most enzymes, which is consistent with an adaptive response against the increase in ROS. In the present case, catalase, GR, glutathione levels and hexose-monophosphate enzymes responsible for NADPH production had their levels increased at 6 h. Again, GST and SOD were the exception to this behaviour having activity levels at 6 h lower than at 3 h. The decrease in GST activity may have helped spare glutathione. Of note, the large increase, up to two-fold, in catalase activity observed at 6 h. This could be indicative that H2O2 production triggered by QNACR may have been a key ROS mediator for the observed cellular effects, and that catalase was specifically up-regulated to counteract H2O2. This is consistent with the increase in DCFH signal, a probe often used to detect H2O2, and it is also consistent with the protective effect observed for Trolox and NAC.
At longer incubation times, glutathione levels and hexose-monophosphate enzymes were the only parameters that were still higher than control. All other antioxidants were inhibited, or similar to control as in the case of GR, indicating probably a failed antioxidant response that resulted in cell-death. This failed antioxidant response is a critical parameter for the anticancer potential of a compound (Supplemental, Figure 2b).
In this context, ROS overproduction could be considered as potential alternative for novel anticancer therapy developments leading to irreversible cell injury which may exhaust the capacity of SOD and other antioxidant enzymesCitation9. Thus, the quinoline derivative, FBA-TPQ, exerts its activity through ROS-associated activation of apoptosis in ovarian cancer in vitroCitation19. In addition, it has been demonstrated that epigallocatechin gallate (EGCG) induces ROS leading to DNA oxidative damage, which could be responsible for its antitumour actionsCitation6. QNACR could also inhibits angiogenesis, as we already reported recently in another studyCitation7 and this effect could be due to its pro-oxidative properties besides its actions inhibiting MMP9; indeed neovascularization suppression in vivo and in vitro could be a response of augmenting ROS generationCitation20. The involvement of QNACR on angiogenesis inhibition and apoptosis should be addressed in further studies to understand the more specific molecular mechanism of this analogue. However, a therapeutic strategy could be using agents that cause ROS accumulation to preferentially kill cancer cells and/or inhibiting the antioxidant enzymes as we demonstrated in this study.
Conclusions
QNACR was able to trigger ROS levels and lipid peroxidation as well as inhibited catalase and dehydrogenase enzymes of the pentose phosphate pathway in short periods of incubations. The adaptive response that followed this acute oxidative injury was not sufficient to spare MCF-7 cells from the toxic effects of QNACR. These results suggest that besides its effects on the inhibition of matrix metalloproteases and its ability to decrease tumour growth in animal modelsCitation7, the antitumour activity of this compound could be also involved in the disturbance of the oxidant/antioxidant status.
Supplementary Material
Download PDF (58.2 KB)Acknowledgements
JRR acknowledges a postdoctoral fellowship (SFRH/BPD/64619/2009) from FCT.
Declaration of interest
This study was supported through Grants PTDC/QUI/69466/2006 and PEst-OE/QUI/UI0612/2011 from Fundação para a Ciência e Tecnologia (FCT), Portugal and grant CDCH-UCV No. PG-06-7548-2009/1 from Central University of Venezuela.
References
- Bratt O, Häggman M, Ahlgren G, Nordle O, Björk A, Damber JE. Open-label, clinical phase I studies of tasquinimod in patients with castration-resistant prostate cancer. Br J Cancer 2009;101:1233–1240.
- Chen Y, Chen S, Lu X, Cheng H, Ou Y, Cheng H et al. Synthesis, discovery and preliminary SAR study of benzofuran derivatives as angiogenesis inhibitors. Bioorg Med Chem Lett 2009;19:1851–1854.
- Chien CM, Yang SH, Lin KL, Chen YL, Chang LS, Lin SR. Novel indoloquinoline derivative, IQDMA, suppresses STAT5 phosphorylation and induces apoptosis in HL-60 cells. Chem Biol Interact 2008;176:40–47.
- Mabeta P, Auer R, Mphahlele MJ. Evaluation of the antiangiogenic effects of 2-aryl-3-bromoquinolin-4(1H)-ones and a NCH3-4-oxo derivative. Biol Pharm Bull 2009;32:937–940.
- Horan TC, Zompa MA, Seto CT, Kim KK, Moore RG, Lange TS. Description of the cytotoxic effect of a novel drug Abietyl-Isothiocyanate on endometrial cancer cell lines. Invest New Drugs 2012;30:1460–1470.
- Li GX, Chen YK, Hou Z, Xiao H, Jin H, Lu G et al. Pro-oxidative activities and dose-response relationship of (-)-epigallocatechin-3-gallate in the inhibition of lung cancer cell growth: A comparative study in vivo and in vitro. Carcinogenesis 2010;31:902–910.
- Rodrigues JR, Charris J, Ferrer R, Gamboa N, Angel J, Nitzsche B et al. Effect of quinolinyl acrylate derivatives on prostate cancer in vitro and in vivo. Invest New Drugs 2012;30:1426–1433.
- Rodrigues J, Abramjuk C, Vásquez L, Gamboa N, Domínguez J, Nitzsche B et al. New 4-maleamic acid and 4-maleamide peptidyl chalcones as potential multitarget drugs for human prostate cancer. Pharm Res 2011;28:907–919.
- Rodrigues JR, Charris J, Camacho J, Barazarte A, Gamboa N, Antunes F. Cytotoxic effects of N’-formyl-2-(5-nitrothiophen-2-yl) benzothiazole-6-carbohydrazide in human breast tumor cells by induction of oxidative stress. Anticancer Res 2012;32:2721–2726.
- Ohkawa H, Ohishi N, Yagi K. Assay for lipid peroxides in animal tissues by thiobarbituric acid reaction. Anal Biochem 1979;95:351–358.
- Goldberg DM, Spooner RJ. (1983).Glutathione reductase. In: Bergmeyer HU, ed. Methods of Enzymatic Analysis. Deerfield Beach, Florida: Verlag Chemie, 258–265.
- Rice KP, Penketh PG, Shyam K, Sartorelli AC. Differential inhibition of cellular glutathione reductase activity by isocyanates generated from the antitumor prodrugs Cloretazine and BCNU. Biochem Pharmacol 2005;69:1463–1472.
- Tian WN, Pignatare JN, Stanton RC. Signal transduction proteins that associate with the platelet-derived growth factor (PDGF) receptor mediate the PDGF-induced release of glucose-6-phosphate dehydrogenase from permeabilized cells. J Biol Chem 1994;269:14798–14805.
- Bradford M. A Rapid and sensitive method for the quantitation of microgram quantities of protein utilizing the principle of protein-dye binding. Anal Biochem 1976;72:248–254.
- Key TJ, Verkasalo PK, Banks E. Epidemiology of breast cancer. Lancet Oncol 2001;2:133–140.
- Pelicano H, Carney D, Huang P. ROS stress in cancer cells and therapeutic implications. Drug Resist Updat 2004;7:97–110.
- Zdolsek JM, Svensson I. Effect of reactive oxygen species on lysosomal membrane integrity. A study on a lysosomal fraction. Virchows Arch, B, Cell Pathol 1993;64:401–406.
- Crawford DR, Davies KJ. Adaptive response and oxidative stress. Environ Health Perspect 1994;102 Suppl 10:25–28.
- Chen T, Xu Y, Guo H, Liu Y, Hu P, Yang X et al. Experimental therapy of ovarian cancer with synthetic makaluvamine analog: In vitro and in vivo anticancer activity and molecular mechanisms of action. PLoS ONE 2011;6:e20729.
- Yang C, Mwaikambo BR, Zhu T, Gagnon C, Lafleur J, Seshadri S et al. Lymphocytic microparticles inhibit angiogenesis by stimulating oxidative stress and negatively regulating VEGF-induced pathways. Am J Physiol Regul Integr Comp Physiol 2008;294:R467–R476.